A Bold Critic of the Big Bang’s ‘Smoking Gun’
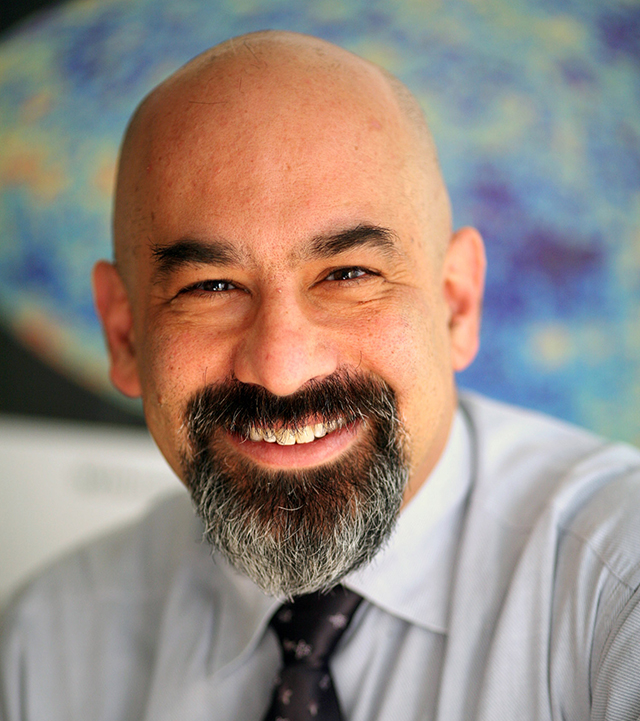
In mid-March, a panel of four astrophysicists working on an experiment to probe the first moments of time held an extraordinary press conference at the Harvard-Smithsonian Center for Astrophysics (CfA) in Cambridge, Mass. The scientists announced that a radio telescope located at the South Pole had discovered gravitational waves generated by the Big Bang. They posted a non-peer-reviewed paper on the Internet that proclaimed the beginning of a “new era” in cosmology.
Sharing the spotlight at the press conference were Andrei Linde and Alan Guth, two theoretical physicists who have developed seminal theories of how our universe rapidly inflated at its birth. The new results validated those theories — or so it seemed.
Stanford University and the CfA both distributed press releases calling the discovery a “smoking gun” showing that the theory of inflation is true, a phrase that appeared in international headlines about the findings. A short video of Stanford researcher Chao-Lin Kuo walking up Linde’s driveway to share the news of the discovery was viewed by millions. Smiling physicists and cosmologists nearly danced with excitement in media interviews.
Most of them were careful to point out that the revolutionary claim from the scientists involved in the experiment, which used the BICEP2 telescope (for Background Imaging of Cosmic Extragalactic Polarization, second generation), must be confirmed by additional experiments that could rule out alternative explanations. But because BICEP2’s very technical paper asserted that the possibility of the gravitational-wave signal being false was very low, the discovery seemed like a done deal to most people.
But not to David Spergel. Spergel earned his stripes in astrophysics as a team member on the Wilkinson Microwave Anisotropy Probe (WMAP), a radio telescope that launched into orbit in 2001. The satellite’s measurements of the microwave afterglow of the Big Bang pinpointed the age of our expanding universe (13.77 billion years) and revealed its ratio of ordinary matter (4.6 percent of the total) to dark matter (24 percent) and dark energy (71.4 percent). (The Planck satellite data has since revised the age closer to 13.8 billion years and the percentages of ordinary matter, dark matter and dark energy to 4.9, 26.8 and 68.3, respectively.)
Spergel is involved in several other experiments that are looking for evidence of gravitational waves from the Big Bang. He is not a member of BICEP2, which also measures the cosmic microwave background (CMB), but he had been lecturing on it to his students at Princeton University. Reading the BICEP2 paper on the train, he spotted serious problems in the interpretation of the data, especially in light of new CMB measurements that were published after the BICEP2 announcement. In late May, Spergel collaborated with Raphael Flauger, a physicist at New York University and the Institute for Advanced Study in Princeton, N.J., and J. Colin Hill, a Princeton University graduate student, to co-publish a critique of the BICEP2 team’s results. The three concluded that there was a strong possibility that the signal could have been generated by cosmic dust in the galactic foreground rather than by gravitational waves. Other cosmologists joined in, including Michael J. Mortonson and Uroš Seljak of the University of California, Berkeley, who wrote their own critical paper, concluding, “It is thus too early to celebrate the BICEP2 results as a definitive proof of inflation.”
The BICEP2 astrophysicists should have been more cautious.
On June 20, Physical Review Letters published a revised version of BICEP2’s original paper. In this new paper the astrophysicists seriously qualified their gravitational-wave interpretation. Most significantly, they deleted references to data about dust that was obtained from an unpublished presentation given by scientists from the European Space Agency’s Planck space observatory, which was doing similar research. They also incorporated Planck data about dust that had not been available when they posted the first version of the paper. With footnoted nods to Spergel and his colleagues, the BICEP2 scientists acknowledged that they could no longer “exclude the possibility of dust emission bright enough to explain the entire excess signal.”
In mid-June, Quanta Magazine sat down with Spergel to talk about the BICEP2 controversy. This is a condensed and edited version of that conversation.
QUANTA MAGAZINE: You are a leading critic of the BICEP2 team’s claim to have discovered the existence of primordial gravitational waves. Why?
DAVID SPERGEL: The BICEP2 astrophysicists should have been more cautious. The evidence they have reported fails to convince me that the signal they interpret as being caused by gravitational waves is not, in fact, caused by galactic dust.
Did the press overreact?
I do not blame the press for going with the smoking-gun story, but I do fault the BICEP2 project scientists for overreaching, for making claims that are not fully supported by their own data.
Does BICEP2’s modification of its smoking-gun claim in the revised paper published in Physical Review Letters satisfy you?
The revised paper still uses unrealistic foreground models that very likely underestimate the strength of the dust signal. But it is a positive sign that the team members now recognize that they can no longer rule out the possibility that their gravitational-wave signal is false.
The BICEP2 papers are extremely technical. In ordinary language, why is polarization important?
Light is describable as an electromagnetic wave and as a particle, a photon. Direct sunlight is unpolarized — the photons scatter randomly in all directions. But when a photon bounces off a surface, such as water or a mirror, the scattered light wave is constrained to oscillate up and down or left to right in relation to its plane of travel. That is polarization.
We see polarized light in everyday life: At the beach on a hazy day, polarized sunglasses filter out polarized glare from water-scattered photons, so that we can better see our surroundings.
In astrophysics, radio telescopes measure the polarization of cosmic microwaves, which are photons that have been traveling toward us for more than 13 billion years. The polarization patterns created as our instruments measure the properties of these photons can reveal the past presence of gravitational waves.
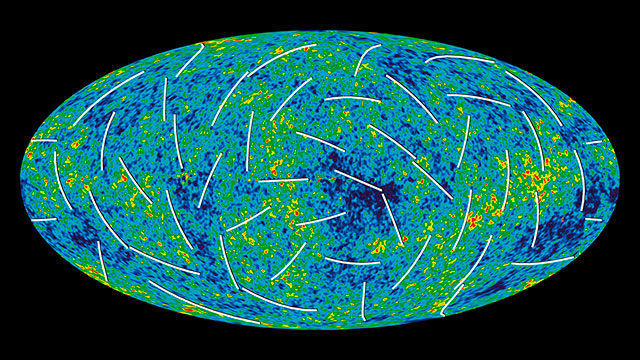
What is a gravitational wave?
A gravitational wave is a distortion in the fabric of space-time. The waves propagate in a way that’s akin to water waves, which ripple concentrically away from a rock thrown into a lake. Colliding neutron stars are thought to emit large gravitational waves, but they are so energetically weak that we have yet to find one experimentally. However, if the theory of inflation is true, during the micro-instant of post-Big Bang inflation, the universe expanded exponentially, faster than the speed of light, driven by a spurt of incredibly high energy.
During this trillionth of a trillionth of a trillionth of a second, quantum mechanical fluctuations in space-time generated gravitons, gravitational ripples in space-time. These tiny waves gradually stretched to hundreds of thousands of light years across as the expanding, lightless universe cooled down following the super-hot instant of inflation.
And then, suddenly, there was light: At the “surface of last scattering,” which emerged about 380,000 years after inflation ended, photons were set free to travel in all directions. And here is the trick: Gravitational waves move electrons around. Some of the photons bounced off electrons that were being moved around by gravitational waves that had been quantum-mechanically created during inflation and had become classical objects.
The theory is that these photons produced a curling pattern of polarization, called a B-mode, that is causally connected to what happens when an electron interacts with a gravitational wave.
Astrophysicists have predicted that this B-mode polarization pattern is recorded ever so faintly in the cosmic microwave background. The polarization pattern that BICEP2 detected in the CMB could effectively be a snapshot of primordial gravitational waves.
Or, it could be a pattern produced by photons polarized by scattering off flecks of silicon dust floating around the Milky Way, a younger B-mode pattern that is identical to the 13.8-billion-year-old pattern.
Why is finding evidence of gravitational waves such a big deal?
Discovering the presence of primordial gravitational waves opens a window into the kind of physics that happened 10-35 seconds after the Big Bang at an energy scale that is a trillion times larger than the energy regime that can be probed by the Large Hadron Collider. In effect, we would be seeing the motion of gravitons — quantized gravity.
And that would mean that the theory of inflation is provably true?
What does it mean to prove a theory? We mostly disprove things. Inflation is a scenario more than a theory, because there are so many versions of it. The inflation scenario makes predictions that have been verified by experiments, and that is great. My view, though, is that while inflation points us in the right direction, it is not the final theory.
That said, if the non-dust B-mode signal is as large as the BICEP2 researchers claim, it requires the existence of large-field inflation at an energy scale that unites the realms of quantum mechanics and general relativity. The existence of strong gravitational waves would rule out many competing theories of quantum gravity and indicate the correct path forward.
The BICEP2 team members incorrectly analyzed the Planck data.
What is the problem with the BICEP2 researchers’ interpretation of their own data?
The experimental difficulty lies in determining how much of a B-mode signal is dust and other possible data contaminants. Subtract those quantities from the total B-mode signal, and the remainder, if any, is due to ancient gravitational waves. BICEP2 made a very sensitive measurement of the sky at a single radio frequency, 150 GHz. With only a single frequency reading, we cannot tell if the B-mode signal is caused by galactic dust or by gravitational waves. We need multifrequency data.
Why did BICEP2 restrict the search to one frequency?
It turns out that 150 GHz is the frequency at which we can build the most sensitive detectors. That approach is just fine, but we must also explore a range of higher and lower frequencies to confirm the origin of the B-mode pattern. There are signal-to-noise constraints on picking searchable frequencies for ground-based telescopes, however. At ground level, oxygen emits radio noise at 60 GHz, and water is loud at 120 GHz, for example.
Are space-based telescopes, such as the Planck satellite, better equipped to find gravitationally induced B-mode polarization patterns?
Ground-based detectors are more technologically advanced than space-based telescopes, because the design for space instruments has to be frozen five or six years before launch. But space is a much cleaner environment than the sub-atmospheric. There are no worries out there about oxygen and water signals contaminating the data and limiting the use of certain frequencies. Space-based telescopes can search larger swaths of the sky, but they are much more expensive to operate. It’s a trade-off, but we need data from both ground and space to produce maximum coverage.
Can Planck determine if BICEP2’s proposed gravitational-wave signal is the real thing?
Dust is much brighter at higher frequencies. Planck is operating on a 353 GHz channel. Dust is 20 times brighter at 353 GHz than at the 150 GHz searched by BICEP2. If Planck detects only a weak signal from polarized dust at 353 GHz, then we will have more confidence in BICEP2’s claim to have discovered gravitational waves. But if Planck observes a lot of B-mode polarization at 353 GHz, then, sadly, BICEP2’s finding will be polarized dust.
One of the criticisms of BICEP2 is that the researchers factored in summary data about dust distributions obtained from a Planck slide that was shown at a conference a year ago.
The BICEP2 team members incorrectly analyzed the Planck data. They claimed that there is only one chance in a million that dust polarization could account for the B-mode signal. That is because they misinterpreted the summary results displayed on the Planck slide.
Why didn’t they use the raw Planck data?
Planck’s raw data will not be made public until the end of the year.
Did you ask the BICEP2 scientists for access to their data?
Yes, but they declined to release it, saying that their data is not calibrated.
Is it common practice to hold onto such data?
It’s fine to keep raw data secret until it is properly calibrated by scientists who understand the limitations of the instrument that collected the data. But if you are willing to publish a paper based upon your data, then you should make that data public.
How do you think this controversy will affect the public’s perception of how science works?
The optimistic scenario is that the public will say, hey, this is great, it helps science when people check each other’s data. If the sausage is cleanly made, a good restaurant will not mind having a window into the kitchen. The negative side is, as one of my right-wing Facebook friends remarked: If the scientists get gravity waves wrong, why should we believe them on global warming?
Correction: This article was revised on July 4, 2014, to reflect David Spergel’s role as a theorist on the WMAP team. He was not the principal investigator.
This article was reprinted on ScientificAmerican.com.