How Life Turns Asymmetric
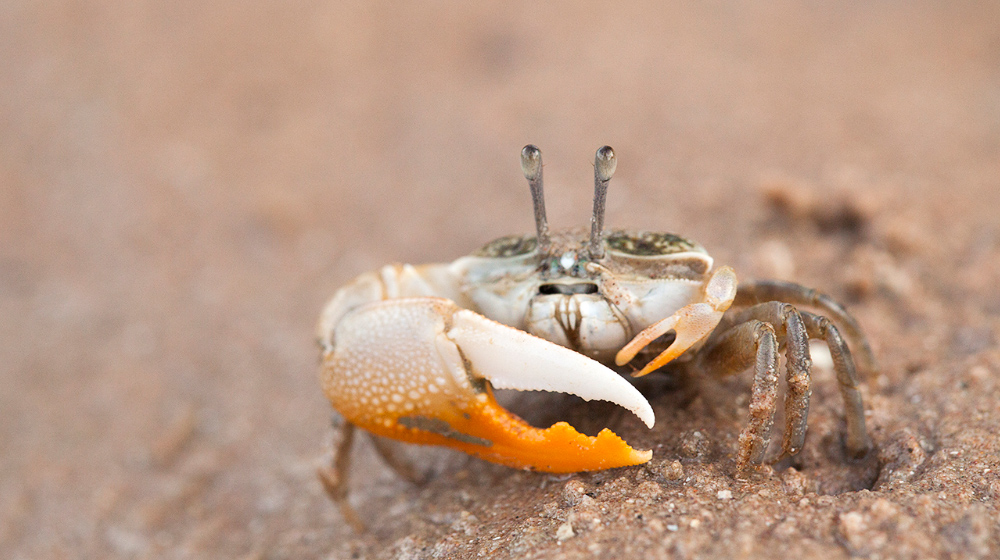
In 2009, after she was diagnosed with stage 3 breast cancer, Ann Ramsdell began to search the scientific literature to see if someone with her diagnosis could make a full recovery. Ramsdell, a developmental biologist at the University of South Carolina, soon found something strange: The odds of recovery differed for women who had cancer in the left breast versus the right. Even more surprisingly, she found research suggesting that women with asymmetric breast tissue are more likely to develop cancer.
Asymmetry is not readily apparent. Yet below the skin, asymmetric structures are common. Consider how our gut winds its way through the abdominal cavity, sprouting unpaired organs as it goes. Or how our heart, born from two identical structures fused together, twists itself into an asymmetrical pump that can simultaneously push oxygen-rich blood around the body and draw in a new swig from the lungs, all in a heartbeat. The body’s natural asymmetry is crucially important to our well-being. But, as Ramsdell knew, it was all too often ignored.
In her early years as a scientist, Ramsdell never gave asymmetry much thought. But on the day of her dissertation defense, she put a borrowed slide into a projector (this in the days before PowerPoint). The slide was of a chick embryo at the stage where its heart begins to loop to one side. Afterward a colleague asked why she put the slide in backward. “It’s an embarrassing story,” she said, “but I had never even thought about the directionality of heart looping.” The chick’s developing heart could distinguish between left and right, same as ours. She went on to do her postdoctoral research on why the heart loops to one side.
Years later, after her recovery, Ramsdell decided to leave the heart behind and to start looking for asymmetry in the mammary glands of mammals. In marsupials like wallabies and kangaroos, she read, the left and the right glands produce a different kind of milk, geared toward offspring of different ages. But her initial studies of mice proved disappointing — their left and right mammary glands didn’t seem to differ at all.
Then she zoomed in on the genes and proteins that are active in different cells of the breast. There she found strong differences. The left breast, which appears to be more prone to cancer, also tends to have a higher number of unspecialized cells, according to unpublished work that’s undergoing peer review. Those allow the breast to repair damaged tissue, but since they have a higher capacity to divide, they can also be involved in tumor formation. Why the cells are more common on the left, Ramsdell has not yet figured out. “But we think it has to do with the embryonic environment the cells grow up in, which is quite different on both sides.”
Ramsdell and a cadre of other developmental biologists are trying to unravel why the organisms can tell their right from left. It’s a complex process, but the key orchestrators of the handedness of life are beginning to come into clearer focus.
A Left Turn
In the 1990s, scientists studying the activity of different genes in the developing embryo discovered something surprising. In every vertebrate embryo examined so far, a gene called Nodal appears on the left side of the embryo. It is closely followed by its collaborator Lefty, a gene that suppresses Nodal activity on the embryo’s right. The Nodal-Lefty team appears to be the most important genetic pathway that guides asymmetry, said Cliff Tabin, an evolutionary biologist at Harvard University who played a central role in the initial research into Nodal and Lefty.
But what triggers the emergence of Nodal and Lefty inside the embryo? The developmental biologist Nobutaka Hirokawa came up with an explanation that is so elegant “we all want to believe it,” Tabin said. Most vertebrate embryos start out as a tiny disk. On the bottom side of this disk, there’s a little pit, the floor of which is covered in cilia — flickering cell extensions that, Hirokawa suggested, create a leftward current in the surrounding fluid. A 2002 study confirmed that a change in flow direction could change the expression of Nodal as well.
Damaged cilia have long been associated with asymmetry-related disease. In Kartagener syndrome, for example, immobile cilia in the windpipe cause breathing difficulties. Intriguingly, the body asymmetry of people with the syndrome is often entirely inversed, to become an almost perfect mirror image of what it would otherwise. In the early 2000s, researchers discovered that the syndrome was caused by defects in a number of proteins driving movement in cells, including those of the cilia. In addition, a 2015 Nature study identified two dozen mouse genes related to cilia that give rise to unusual asymmetries when defective.
Yet cilia cannot be the whole story. Many animals, even some mammals, don’t have a ciliated pit, said Michael Levin, a biologist at Tufts University who was the first author on some of the Nodal papers from Tabin’s lab in the 1990s.
In addition, the motor proteins critical for normal asymmetry development don’t only occur in the cilia, Levin said. They also work with the cellular skeleton, a network of sticks and strands that provides structure to the cell, to guide its movements and transport cellular components.
An increasing number of studies suggest that this may give rise to asymmetry within individual cells as well. “Cells have a kind of handedness,” said Leo Wan, a biomedical engineer at the Rensselaer Polytechnic Institute. “When they hit an obstacle, some types of cells will turn left while others will turn right.” Wan has created a test that consists of a plate with two concentric, circular ridges. “We place cells between those ridges, then watch them move around,” he said. “When they hit one of the ridges, they turn, and their preferred direction is clearly visible.”
Wan believes the cell’s preference depends on the interplay between two elements of the cellular skeleton: actin and myosin. Actin is a protein that forms trails throughout the cell. Myosin, another protein, moves across these trails, often while dragging other cellular components along. Both proteins are well-known for their activity in muscle cells, where they are crucial for contraction. Kenji Matsuno, a cellular biologist at Osaka University, has discovered a series of what he calls “unconventional myosins” that appear crucial to asymmetrical development. Matsuno agrees that myosins are likely causing cell handedness.
Consider the fruit fly. It lacks both the ciliated pit as well as Nodal, yet it develops an asymmetric hindgut. Matsuno has demonstrated that the handedness of cells in the hindgut depends on myosin and that the handedness reflected by the cells’ initial tilt is what guides the gut’s development. “The cells’ handedness does not just define how they move, but also how they hold on to each other,” he explains. “Together those wrestling cells create a hindgut that curves and turns exactly the way it’s supposed to.” A similar process was described in the roundworm C. elegans.
Nodal isn’t necessary for the development of all asymmetry in vertebrates, either. In a study published in Nature Communications in 2013, Jeroen Bakkers, a biologist at the Hubrecht Institute in the Netherlands, described how the zebra fish heart may curve to the right in the absence of Nodal. In fact, he went on to show that it even does so when removed from the body and deposited into a simple lab dish. “That being said,” he adds, “in animals without Nodal, the heart did not shift left as it should, nor did it turn correctly. Though some asymmetry originates within, the cells do need Nodal’s help.”
How Cells Talk
For Tabin, experiments like this show that while Nodal may not be the entire story, it is the most crucial factor in the development of asymmetry. “From the standpoint of evolution, it turns out, breaking symmetry wasn’t that difficult,” he said. “There are multiple ways of doing it, and different organisms have done it in different ways.” The key that evolution had to solve was making asymmetry reliable and robust, he said. “Lefty and Nodal together are a way of making sure that asymmetry is robust.”
Yet others believe that important links are waiting to be discovered. Research from Levin’s lab suggests that communication among cells may be an under-explored factor in the development of asymmetry.
The cellular skeleton also directs the transport of specialized proteins to the cell surface, Levin said. Some of these allow cells to communicate by exchanging electrical charges. This electrical communication, his research suggests, may direct the movements of cells as well as how the cells express their genes. “If we block the [communication] channels, asymmetrical development always goes awry,” he said. “And by manipulating this system, we’ve been able to guide development in surprising but predictable directions, creating six-legged frogs, four-headed worms or froglets with an eye for a gut, without changing their genomes at all.”
The apparent ability of developing organisms to detect and correct their own shape fuels Levin’s belief that self-repair might one day be an option for humans as well. “Under every rock, there is a creature that can repair its complex body all by itself,” he points out. “If we can figure out how this works,” Levin said, “it might revolutionize medicine. Many people think I’m too optimistic, but I have the engineering view on this: Anything that’s not forbidden by the laws of physics is possible.”
This article was reprinted on Wired.com.