Debating the Evolution of Multicellularity
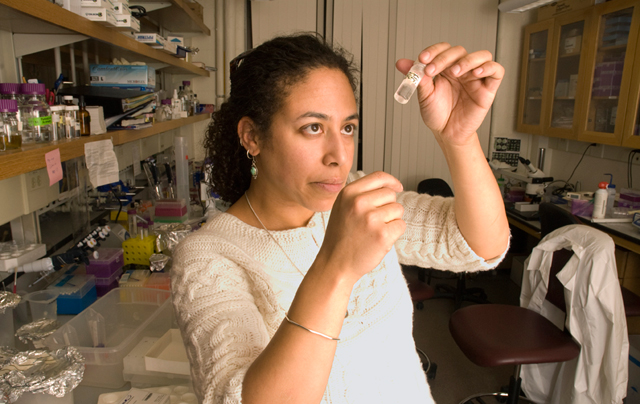
The developmental biologist Cassandra Extavour sings classical and baroque music on stage with the Handel and Haydn Society at Symphony Hall in Boston. Blessed with a beautiful soprano voice, she could easily have chosen to pursue a career as a singer. But a summer working in a developmental genetics laboratory as an undergraduate tipped the scales in favor of science, and Extavour is now an associate professor of organismic and evolutionary biology at Harvard University. By choosing biology, she says, she has been able to pursue her musical career part time; a full-time concert soprano, by contrast, would not have had the time to run a lab on the side.
Extavour directs a national research collaborative called EDEN, which stands for Evo-Devo-Eco (evolutionary-developmental-ecological) Network. The organization, funded by the National Science Foundation, encourages geneticists to dissect more exotic creatures than the ubiquitous fruit fly, Drosophila melanogaster. EDEN researchers model the various evolutionary paths of sea anemones, horseshoe crabs, mosses, crickets, spiders, milkweed bugs and the super-hardy tardigrade. Extavour’s own lab focuses on dissecting insect embryos and ovaries, searching for genetic clues to the origin of multicellularity and the complex organisms that multicellularity made possible, including Homo sapiens. Extavour’s special expertise is in tracking the development of germ cells, the cells created in an embryo that contain the genetic code for reproducing multicellular organisms.
Last winter, Extavour was one of the organizers of a ten-week program on a controversial topic, “Cooperation and the Evolution of Multicellularity,” at the Kavli Institute for Theoretical Physics at the University of California, Santa Barbara. The daily talk sessions were attended by scores of highly regarded scientists from all over the world, including developmental and evolutionary biologists, mathematical physicists, and zoologists — plus an embedded journalist reporting for Quanta Magazine. The event was unusual because of its prolonged and multidisciplinary nature. And many exchanges were heated because, despite 150 years of research on the biology of evolution, scientists still disagree about how and why multicellular creatures and plants emerged from ancient oceans that teemed with robust and self-reliant single-celled entities.
At the conference, biologists who work mostly in the field observing the behaviors of bees, ants, wolves, slime molds and other creatures tended to look for the mechanics of natural selection at the behavioral level by examining how individual organisms self-organize into hives, nests, packs, conglomerates or families. Physicists and molecular biologists focused more on the micromechanics of natural selection at the level of the genome, looking to mathematically measure the “fitness” of what they call competing and cooperating genes or cells.
But the scientists were not always on the same page even regarding the meaning of such key concepts as cooperation, competition and fitness. The physicists and molecular biologists employed statistical mechanics and game theory to help build explanatory mathematical models of DNA, proteins and entire genomes. That preoccupation with micro-quantification sometimes raised the hackles of field-oriented biologists who were more focused on analyzing social behaviors.
Scientists need to be crystal-clear that fitness is a statement of fact and not a value judgment.
Through it all, week after week, the unflappable Extavour kept the conferees focused on the central issue: Precisely what physical mechanisms originally drove single cells to unite for mutual benefit? How can we quantify this benefit in evolutionary terms? A few months after the conference, Quanta Magazine interviewed Extavour about her own point of view. This is a condensed and edited version of that interview, incorporating a portion of her final talk at Kavli.
QUANTA MAGAZINE: Are you evo, devo or eco?
CASSANDRA EXTAVOUR: As a developmental biologist — a devo, if you will — I am intrigued by how cells become eggs and sperm in multicellular creatures. During the development of an animal embryo from a fertilized egg cell, a process that’s called embryogenesis, only a tiny percentage of the millions of genetically identical cells that make up the embryo will become gametes capable of passing their genomes on to successive generations.
Most of an embryo’s cells become soma: cells capable of forming vital organs, muscle, skin and bones. Somatic cells reproduce by dividing — genetically mirroring themselves — but they cannot contribute their specific genomes to the formation of new creatures through sexual reproduction. That is solely the job of the succession of cells in what we call a germ line.
In a sense, the somatic cells sacrifice their genetic “immortality” to protect the germ-line cells. And this primal division of reproductive labor has evolutionary consequences: It allows sexual reproduction and fosters genetic diversity and the evolution of multicellularity.
Now the eco in evo-devo-eco comes into play. The core problem in the study of the development of multicellular organisms is: Why do cells that start out with identical genomes do different things in different environments?
How do you track the development of germ cells in the lab?
We dissect the ovaries and embryos of spiders, crickets and milkweed bugs, using molecular biology and microscopy tools to map the genetic mechanisms that guide the emergence of germ cells. In some organisms, the assignment of a cell to the germ line is caused by an inheritance-based mechanism: Before there is even an embryo, the molecular content of some cells predetermines them to develop as either germ or soma. In other organisms, there is instead a signaling mechanism: An embryonic cell receives chemical signals from neighboring cells that activate (or repress) the genes that allow for germ-line function.
Why bother to be multicellular?
The evolution of a distinct germ line that is protected by the diverse somatic functions of the organism is thought to confer an evolutionary advantage — what we call a fitness benefit — to multicellular organisms, whether plant or animal or slime mold.
How so?
The strict division of labor between somatic and germ-line cells in a multicellular conglomerate may allow it to explore new ecological niches and to manipulate objects with specialized multicellular organs like jaws, paws, stalks, tentacles, tails, roots, leaves or fingers. Unlike the typical single cell that is tethered to a limited environment, a multicellular unit can roam over great distances in search of food or more favorable ecological conditions or other multicellular units. It is possible that multicellular species may find more opportunities to adapt successfully to drastically changing ecosystems that might wipe out a less mobile or less complex unicellular species. So while unicellularity is clearly a successful way of life for many organisms, for others the collective benefit of multicellularity appears to outweigh the loss of individual fitness for each somatic cell that is denied a chance to pass on its particular genome.
There may be no words that can replace the mathematical structures without carrying some normative baggage.
What do you mean by fitness?
Fitness can have an unambiguous mathematical definition in the field of population genetics, but in other areas of biology fitness is hard to pin down semantically. So are words like conflict and cooperation when they are used to describe the behavior of nonhuman organisms — yeast or algae or rabbits. Such words are necessary to communicate what goes on non-mathematically, but they are also sociologically loaded: They imply an ability to make choices. In my lab, we avoid defining fitness using absolute numerical values. We do bookkeeping: We count how many eggs are laid. We count how many eggs are hatched. But we do not suggest that these numbers alone are somehow equivalent to something that we can confidently label fitness.
How has the sociological use of the concept of fitness evolved since the publication of Charles Darwin’s Origin of Species?
Ever since the social Darwinist Herbert Spencer launched the “survival of the fittest” trope 150 years ago, people have equated survival with fitness and fitness with survival. It’s a tautology that causes us trouble, and yet it lingers. For example, the mathematical biologist R. A. Fisher was a founder of population genetics in the 1920s and 1930s, and his work relating fitness to genetic variance was enormously important. But Fisher himself was a eugenicist who believed that upper-class people, because they are upper class, have a genetic predisposition to superior intelligence and “qualities of the moral character.” He feared that since lower-class individuals seemed to have the highest birth rates, the spread of their collectively inferior genes would lead to the downfall of civilization if left unchecked. He advocated that society take certain measures to ensure the proliferation of upper-class genes. This is one reason why scientists need to be crystal-clear that fitness is a statement of fact and not a value judgment.
Then why use these loaded words?
They evolved as a convenient, if inexact, shorthand. In actuality, there may be no words that can replace the mathematical structures without carrying some normative baggage.
What is the meaning of cooperation in experimental biology?
Biological cooperation can occur when two or more individuals — be they single-celled or multicelled units — group together to form a new entity: a new individual or, perhaps, a group of individuals, depending on the perspective of the experimenter. The division of reproductive labor in a multicellular entity requires that cells work together to maximize the reproductive potential of the entity of which they are a part.
Cells are not conscious beings competing in a game like Prisoner’s Dilemma, say, which treats outcomes as decisions.
To that end, cooperative cells exhibit behaviors that improve the ability of other cells to transmit their genomes, sometimes at the expense of minimizing their own genetic contribution to the next generation. These cells are often called “altruists” in the lab. They may produce or preserve energy resources for the group.
On the other hand, a noncooperative cell — or what is labeled a “defector” in the lab — will move around an ecosystem eating up scarce resources without making any contributions to sustainability. The defector’s “selfish” behavior tends to preserve its own genome for intergenerational transmission at the expense of the group.
Sexually reproducing organisms have capitalized on these two conflicting approaches in a way that benefits the entire organism: Somatic cells act as cooperators and germ cells act as defectors. Multicellularity as we know it might not have been possible without having both the defectors and the cooperators — each type of behavior is necessary.
Does Darwinian evolution reward cooperative behaviors?
Behaviors have an effect on the evolution of species and vice versa. But let’s not forget that evolution is the result of random events that generate consequences that are subject to natural selection. Some random changes improve an organism’s chance of surviving and reproducing in a specific environment, and some decrease it. Scientists strive to quantify these changes statistically so that we can make predictions and figure out what happened in the past.
To be clear: There is no conscious goal or direction or systemic intentionality built into the evolutionary trajectory of a given entity, be it unicellular or multicellular. Nonetheless, in scientific conversation, it is often convenient to talk about behaviors as if they are the result of choices. But cells are not conscious beings competing in a game like Prisoner’s Dilemma, say, which treats outcomes as decisions.
Why, then, is game theory used so much in evolutionary biology?
It was originally developed as a tool for finding solutions to zero-sum games played by conscious agents, and it was used extensively for economic analysis in a Western capitalist environment. It is riddled with anthropomorphic, value-laden language and, partly as a result, its adoption by many scholars of evolutionary biology means that we now casually talk about “altruistic” cells that commit genetic “suicide” by “cooperating” with “defectors” who “won” a “competition” for “control” of “public goods” in a test tube.
These human-centric metaphors become problematic when we forget that the linguistic shorthand does not refer to human societies and our own choices. That is why in the evo-devo-eco scheme of things, I am drawn toward developmental biology and its emphasis on understanding physical and chemical structures quantitatively.
Is it possible to unlock the secret of how we — as multicellular creatures — came to be?
My goal is to understand the genetic programs that allowed cells in multicellular entities to evolve a germ-soma division of reproductive labor in specific environments, not to analyze how our multicellular origin has affected the evolution of our societies. Frankly, I am distressed by the amount of subjectivity in human society, and I seek to avoid that in science. I grant, though, that biologists must be fascinated by the evolution of multicellularity, because we are multicellular creatures. A single cell cannot sing with the symphony!
This article was reprinted on ScientificAmerican.com.