The Particle That Broke a Cosmic Speed Limit
On the night of October 15, 1991, the “Oh-My-God” particle streaked across the Utah sky.
A cosmic ray from space, it possessed 320 exa-electron volts (EeV) of energy, millions of times more than particles attain at the Large Hadron Collider, the most powerful accelerator ever built by humans. The particle was going so fast that in a yearlong race with light, it would have lost by mere thousandths of a hair. Its energy equaled that of a bowling ball dropped on a toe. But bowling balls contain as many atoms as there are stars. “Nobody ever thought you could concentrate so much energy into a single particle before,” said David Kieda, an astrophysicist at the University of Utah.
Five or so miles from where it fell, a researcher worked his shift inside an old, rat-infested trailer parked atop a desert mountain. Earlier, at dusk, Mengzhi “Steven” Luo had switched on the computers for the Fly’s Eye detector, an array of dozens of spherical mirrors that dotted the barren ground outside. Each of the mirrors was bolted inside a rotating “can” fashioned from a section of culvert, which faced downward during the day to keep the sun from blowing out its sensors. As darkness fell on a clear and moonless night, Luo rolled the cans up toward the sky.
“It was a pretty crude experiment,” said Kieda, who operated the Fly’s Eye with Luo and several others. “But it worked — that was the thing.”
The faintly glowing contrail of the Oh-My-God particle (as the computer programmer and Autodesk founder John Walker dubbed it in an early Web article) was spotted in the Fly’s Eye data the following summer and reported after the group spent an extra year convincing themselves the signal was real. The particle had broken a cosmic speed limit worked out decades earlier by Kenneth Greisen, Georgiy Zatsepin and Vadim Kuzmin, who argued that any particle energized beyond approximately 60 EeV will interact with background radiation that pervades space, thereby quickly shedding energy and slowing down. This “GZK cutoff” suggested that the Oh-My-God particle must have originated recently and nearby — probably within the local supercluster of galaxies. But an astrophysical accelerator of unimagined size and power would be required to produce such a particle. When scientists looked in the direction from which the particle had come, they could see nothing of the kind.
“It’s like you’ve got a gorilla in your backyard throwing bowling balls at you, but he’s invisible,” Kieda said.
Where had the Oh-My-God particle come from? How could it possibly exist? Did it really? The questions motivated astrophysicists to build bigger, more sophisticated detectors that have since recorded hundreds of thousands more “ultrahigh-energy cosmic rays” with energies above 1 EeV, including a few hundred “trans-GZK” events above the 60 EeV cutoff (though none reaching 320 EeV). In breaking the GZK speed limit, these particles challenged one of the farthest-reaching predictions ever made. It seemed possible that they could offer a window into the laws of physics at otherwise unreachable scales — maybe even connecting particle physics with the evolution of the cosmos as a whole. At the very least, they promised to reveal the workings of extraordinary astrophysical objects that had only ever been twinkles in telescope lenses. But over the years, as the particles swept brushstrokes of light across sensors in every direction, instead of painting a telltale pattern that could be matched to, say, the locations of supermassive black holes or colliding galaxies, they created confusion. “It’s hard to explain the cosmic-ray data with any particular theory,” said Paul Sommers, a semiretired astrophysicist at Pennsylvania State University who specializes in ultrahigh-energy cosmic rays. “There are problems with anything you propose.”
Only recently, with the discovery of a cosmic ray “hotspot” in the sky, the detection of related high-energy cosmic particles, and a better understanding of physics at more familiar energies, have researchers secured the first footholds in the quest to understand ultrahigh-energy cosmic rays. “We’re learning things very rapidly,” said Tim Linden, a theoretical astrophysicist at the University of Chicago.
Ankle Problems
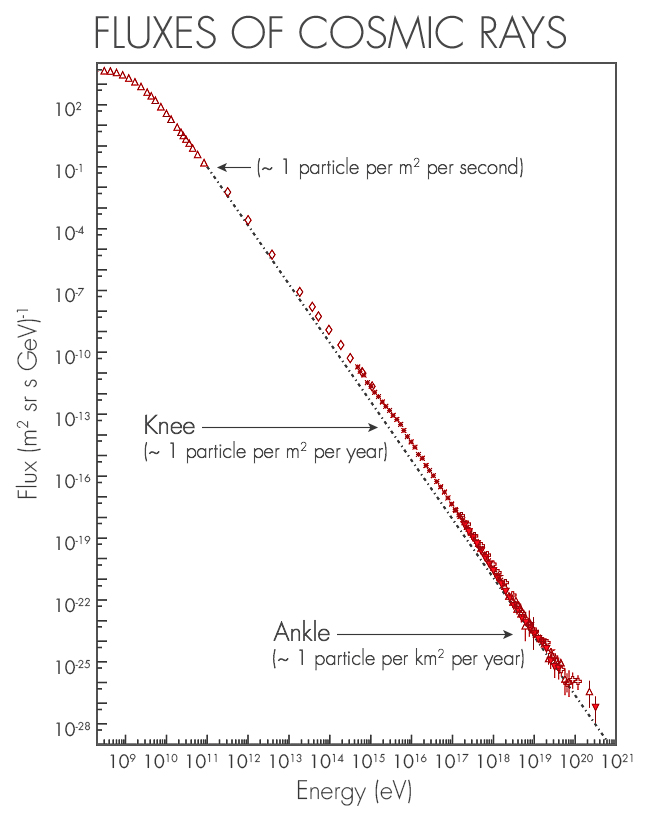
Original data via S. Swordy, U. Chicago.
Thousands of cosmic rays bombard each square foot of Earth’s atmosphere every second, and yet they managed to elude discovery until a series of daring hot-air-balloon rides in the early 1910s. As the Austrian physicist Victor Hess ascended miles into the atmosphere, he observed that the amount of ionizing radiation increased with altitude. Hess measured this buzz of electrically charged particles even during a solar eclipse, establishing that much of it came from beyond the sun. He received a Nobel Prize in Physics for his efforts in 1936.
Cosmic rays, as they became known, arc through Earth’s magnetic field from every direction, and with a smooth spread of energies. (At sea level, we experience the low-energy, secondary radiation produced as the cosmic rays crash through the atmosphere.) Most cosmic rays are single protons, the positively charged building blocks of atomic nuclei; most of the rest are heavier nuclei, and a few are electrons. The more energetic a cosmic ray is, the rarer it is. The rarest of all, those that are labeled “ultrahigh-energy” and exceed 1 EeV, strike each square kilometer of the planet only once per century.
Plotting the number of cosmic rays that sprinkle detectors according to their energies produces a downward-sloping line with two bends — the energy spectrum’s “knee” and “ankle.” These seem to mark transitions to different types of cosmic rays or progressively larger and more powerful sources. The question is, which types, and which sources?
Like many experts, Karl-Heinz Kampert, a professor of astrophysics at the University of Wuppertal in Germany and spokesperson for the Pierre Auger Observatory, the world’s largest ultrahigh-energy cosmic ray detector, believes cosmic rays are accelerated by something like the sonic booms from supersonic jets, but on grander scales. Shock acceleration, as it’s called, “is a fundamental process which you find on any scale in the universe,” Kampert said, from solar flares to star explosions (supernovas) to rapidly spinning stars called pulsars to the enormous lobes emanating from mysterious, super-bright galaxies known as active galactic nuclei. All are cases of heated matter (or “plasma”) flowing faster than the speed of sound, producing an expanding shock wave that accumulates a crust of protons and other particles. The particles reflect back and forth across the shock wave, trapped between the magnetic field of the plasma and the vacuum of empty space like little balls ping-ponging between table and paddle. A particle gains energy with every bounce. “Then it will escape,” Kampert said, “and move through the universe and be detected by an experiment.”
Emily Fuhrman for Quanta Magazine
Cosmic rays are most likely energized through “shock acceleration,” reflecting back and forth across a shock wave that is produced when plasma flows faster than the speed of sound. The stronger and larger the magnetic field of the plasma, the more energy it can impart to a particle. Ultrahigh-energy cosmic rays surpass 1 exa-electron volt (EeV).
Trying to match different shock waves to parts of the cosmic-ray energy spectrum puts astrophysicists on shaky ground, however. They would expect the knee and ankle to mark the highest points to which protons and heavier nuclei (respectively) can be energized in the shock waves of supernovas — the most powerful accelerators in our galaxy. Calculations suggest the protons should max out around 0.001 EeV, and indeed, this aligns with the knee. Heavier nuclei from supernova shock waves are thought to be capable of reaching 0.1 EeV, making this number the expected transition point to more powerful sources of “extragalactic” cosmic rays. These would be shock waves from singular objects that aren’t found in the Milky Way or in most other galaxies, and which could well be galaxy-size themselves. However, the measured ankle of the spectrum — “the only place where it looks like there’s a clear transition,” Sommers said — lies around 5 EeV, an order of magnitude past the theoretical maximum for galactic cosmic rays. No one is sure what to make of the discrepancy.
Past the ankle, at around 60 EeV, the line dips toward zero, forming a sort of toe. This is probably the GZK cutoff, the point beyond which cosmic rays can only tarry for so long before losing energy to ambient cosmic microwaves generated by a phase transition in the early universe. The existence of the cutoff, which Kampert calls “the only firm prediction ever made” about cosmic rays, was established in 2007 by the Fly’s Eye’s successor — the High Resolution Fly’s Eye experiment, or HiRes. From there, the energy spectrum reduces to a trickle of trans-GZK cosmic rays, finally ending, at 320 EeV, with a single data point: the Oh-My-God particle.
The presence of the GZK cutoff means that the laws of physics are operating as expected. Rather than disproving those laws, trans-GZK cosmic rays probably do originate nearby (reaching Earth before ambient microwaves sap their energy). But where, and how? For a maddening 20 years, the particles appeared to come from everywhere and nowhere in particular. But finally a hotspot has developed in the Northern Hemisphere. Could this be the invisible gorilla hurtling bowling balls toward Earth?
Getting Hotter
In Utah, a three-hour drive from the site of the original Fly’s Eye, its latest descendant sprawls across the desert: a 762–square-kilometer grid of detectors called the Telescope Array. The experiment has been tracking the multi-billion-particle “air showers” produced by ultrahigh-energy cosmic rays since 2008. “We’ve been watching the hotspot increase in statistical significance for several years,” said Gordon Thomson, a professor of physics and astronomy at the University of Utah and spokesperson for the Telescope Array.
The hotspot of trans-GZK cosmic rays, which centers on the constellation Ursa Major, was initially too weak to be taken seriously. But in the past year, it has reached an estimated statistical significance of “four sigma,” giving it a 99.994 percent chance of being real. Thomson and his team must reach five-sigma certainty to definitively claim a discovery. (Thomson hopes this will happen in the group’s next data analysis, due out in June.) Already, theorists are treating the hotspot as an anchor for their ideas.
“It’s really exciting,” said Linden. With more data, he explained, the location of the source can be pinpointed within the hotspot (which gets smeared out by the deflection of cosmic rays as they pass through the galaxy’s and Earth’s magnetic fields). By tracking other types of particles coming from the same spot in the sky, “you have a model of how the source works over many orders of magnitude in energy,” he said. The invisible gorilla would materialize.
Meanwhile, some of those other particles are slowly piling up in the sensors of the IceCube detector, a cable-infused, cubic-kilometer block of ice buried beneath the South Pole. For the past four years, IceCube has monitored the rare ice tracks of neutrinos, lightweight elementary particles that usually flit right through matter and thus require immense efforts to detect, but which are produced in abundance from physical processes throughout the universe.
Every so often, cosmic neutrinos interact with atoms and produce radiation as they pass through IceCube; their directions of travel trace a new map of the cosmos that can be compared to the maps of ultrahigh-energy cosmic rays and those of light. In 2013, IceCube scientists reported the observation of the first-ever very-high-energy neutrinos — a pair of 0.001-EeV particles nicknamed “Bert” and “Ernie” that might have come from the same sources that yield ultrahigh-energy cosmic rays. Neutrinos have a big advantage over cosmic rays as messengers from the most powerful objects in the universe: Because they are electrically neutral, they move in straight lines. “Since neutrinos travel to us uninhibited from the source, they might be able to open up a new window on the universe,” said Olga Botner of Uppsala University in Sweden, IceCube’s spokesperson.
Of the 54 high-energy neutrinos that IceCube has detected as of its latest analysis, reported in early May, four originate from the vicinity of the cosmic-ray hotspot. (Neutrinos can enter the detector after traveling through Earth from the northern sky.) This “hint of a correlation,” as Linden described it, could be a clue: Cosmic rays take longer to get to Earth than neutrinos, so a common source would have to have been pumping out energetic particles for many years. Short-lived source candidates such as gamma-ray bursts would be ruled out in favor of stable objects — perhaps a star-forming galaxy with a supermassive black hole at its center. “In the next few years we’re going to get that many more neutrinos, and we’ll see how this correlation plays out,” Linden said. For now, though, the correlation is very weak. “I’m not staking my foot in the ground,” he said.
Alongside cosmic rays and neutrinos, cosmic “gamma rays” (high-energy photons) will serve as a third messenger in the coming years. They’re the subject of several major searches including the HESS (High Energy Stereoscopic System) experiment in Namibia — named in honor of the father of cosmic rays — and VERITAS (Very Energetic Radiation Imaging Telescope Array System) in Arizona, for which Kieda, the former Fly’s Eye scientist, now works. The combination of cosmic-ray, neutrino and gamma-ray data should help locate and sharpen astrophysicists’ picture of the most powerful accelerators in the universe. The search will organize around the hotspot.
Thomson has his money on threads of galaxies and dark matter called “filaments” that are draped throughout the cosmos and which, at hundreds of millions of light-years long, are among the largest structures in existence. There’s a filament in the direction of the hotspot. “It’s probably something in the filament,” Thomson said. In any case, he added, “we have an idea now of interesting places to look. And all we need to do is collect more data.”
Draining the Pool
Kampert, of the Pierre Auger Observatory, is approaching the mystery of ultrahigh-energy cosmic rays from a different direction, by asking: What are they?
Some astrophysicists say the Auger Observatory has been “unlucky.” Covering 3,000 square kilometers of Argentina grasslands, it collects far more data than the Telescope Array, but it does not see a hotspot in the Southern Hemisphere with anywhere near the prominence of the one in the north. It has detected evidence of a slight concentration of trans-GZK cosmic rays in the sky that overlays an active galactic nucleus called Centaurus A as well as another filament. But Kampert says Auger might never collect enough data to prove this so-called “warmspot” is real. Still, the dearth of clues is a mystery in itself.
“It’s a very rich data set and we don’t see anything,” said Sommers, who helped design and organize the Auger Observatory. “That’s absolutely amazing to me. Back in the 1980s I would have bet good money that if we had the statistics we have now, there would be obvious hotspots and patterns. It makes me really wonder.”
Kampert thinks he and his colleagues must simply get smarter about how they look for hotspots, which are surely there; the local region of the universe is not uniformly blanketed by objects capable of accelerating particles to trans-GZK energies. The problem is magnetic deflection, he said. Galactic and extragalactic magnetic fields bend protons five to 10 degrees off-course, and they bend heavier nuclei many times that, depending on the number of protons they contain. Auger’s analysis of its air-shower events (which integrates cutting-edge results from particle collisions at the Large Hadron Collider) suggests that the highest-energy cosmic rays tend to be on the heavy side, consisting of carbon or even iron nuclei.
“If at the highest energies we have [heavier nuclei], then your sky is always fuzzy or smeared out,” Kampert said. “It would be like doing astronomy from the bottom of a swimming pool.”
He and his team hope to update their experiment with the ability to identify the composition of cosmic rays on an event-by-event basis. This will allow them to look for correlations between only the lightest, least deflected particles. “Composition is really the key to understanding the origin of the highest-energy particles,” he said.
And the shift toward heavier nuclei at the far end of the cosmic-ray energy spectrum could be a major clue itself. Just as supernovas accelerate protons no further than the “knee” of the spectrum and can propel only heavier nuclei beyond that point, so too might the most powerful astrophysical accelerators in the universe peter out. Scientists could be glimpsing the true edge of the cosmic-ray spectrum: the points where protons, and then helium, carbon and iron, max out. Measuring this falloff will help expose how the giant accelerators work — and favor certain candidates over others.
Theorists still struggle to imagine any of those candidates producing the sprinkle of particles in the 200-EeV range or the Oh-My-God particle at 320 — even if they are made of iron. “How you get a [320 EeV] particle is not easy from any theory,” Thomson said. “But it was there. It happened.”
Even that fact is called into question. Back in the early 1990s, Sommers, who was temporarily working at the University of Utah, helped the Fly’s Eye scientists analyze their 320-EeV signal. But although the “big event” (as he calls it) was “pretty well measured by the standards of the time,” the Fly’s Eye hadn’t fully transitioned away from being a “monocular” experiment, analogous to one fly’s eye rather than two (a second eye was under construction); it lacked the precision and redundancy of later stereoscopic arrays. Sommers said that although no serious reasons for doubting the energy estimate are known, “one must be suspicious of it now. With vastly greater exposure, the more precise, new observatories have failed to detect any particle of such high energy. The flux of particles at energies that high must be so low that it would have been an incredible fluke that the Fly’s Eye detected one.”
The error bars that went into calculating the Oh-My-God particle’s energy might all have been off in the wrong direction at the same time. If so, it was a lucky mistake for the field, motivating new experiments without greatly misleading researchers, since many other trans-GZK particles have followed. And if the Oh-My-God particle was a mistake, well, probably no one will ever know.
This article was reprinted on BusinessInsider.com.