Searching the Sky for the Wobbles of Gravity
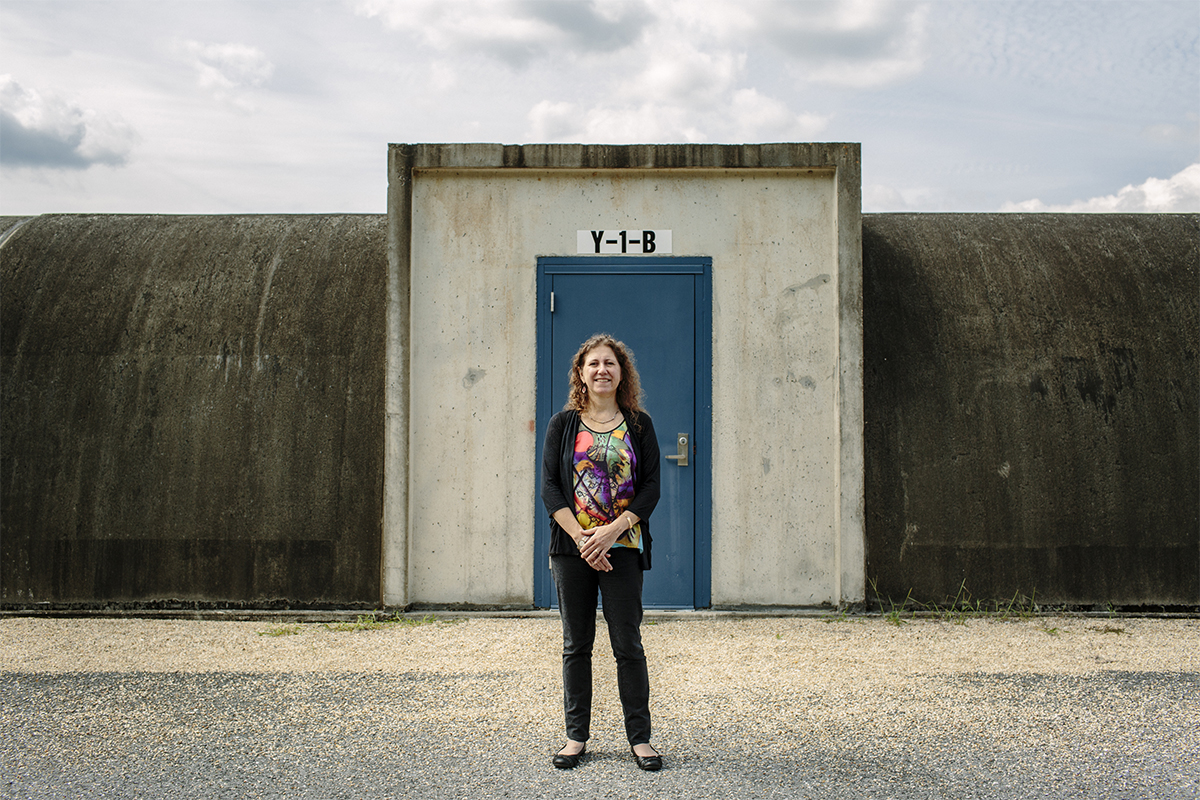
Our ears are now open to gravity’s faint symphony. On September 18, the Advanced Laser Interferometer Gravitational-Wave Observatory, or Advanced LIGO, began to listen for gravitational waves, cosmic ripples that subtly warp the fabric of space-time. Albert Einstein solved the equations that implied that these waves should exist, and nearly a century later, hopes are high that Advanced LIGO is about to directly detect them for the first time.
The long waiting period is an indication of the intense experimental challenges that gravitational-wave researchers face. Even a strong gravitational-wave signal — one produced by the collision of two black holes, for example — might shrink or expand a laser beam several kilometers in length by only a small fraction of the width of a proton. The first iteration of the LIGO experiment, which ran from 2001 to 2010, was thwarted by spurious noises from both human and natural sources — the faint rumbles of a distant cargo train or a felled tree, or even the sudden rush of a gust of wind.
While some experiments are attempting to find indirect evidence for gravitational waves that may have existed just after the Big Bang, Advanced LIGO’s primary aim is to directly detect evidence of gravitational waves from astronomical sources — colliding neutron stars or black holes, for example. It is a telescope that collects gravity, not light.
Like the initial LIGO, Advanced LIGO has detectors at two locations: Hanford, Wash., and Livingston, La. The use of two detectors allows scientists to correlate the signal. A real event should produce a soundlike signal that is recognized by both; a cargo train will not be. The upgraded instrument is some 10 times as sensitive as the initial LIGO.
Gabriela González, a physicist at Louisiana State University in Baton Rouge, has been involved in building, testing and calibrating LIGO since 1993. In 1997 she helped organize the hundreds of scientists involved with the project into the LIGO Scientific Collaboration. Today, the collaboration has more than 900 member scientists. González was elected spokesperson for the collaboration in 2011, effectively making her the organization’s leader. She continues to hold the position today.
Quanta Magazine spoke with González about listening for gravitational waves, the start of Advanced LIGO, and the possibility of seeing gravitational waves for the first time. An edited and condensed version of the interview follows.
QUANTA MAGAZINE: What will gravitational waves tell us?
GABRIELA GONZÁLEZ: Well, first of all and most exciting to me, they’re telling us that space-time is wiggling around. That is just mind-boggling.
Is that what led you to work at LIGO?
When I started college in Argentina, where I am from, the theory of relativity was something that to me was so beautiful. There was a very strong group in theoretical relativity at the University of Córdoba. I started doing some theoretical research. My husband got a Ph.D. about the same time I finished my undergraduate degree. Syracuse University had a strong group in relativity and in quantum gravity, and that’s what my husband wanted to do. So we went to Syracuse. We arrived in February, in the middle of a snowstorm. Talk about a geographical, climatic shock.
When I was in my third year in graduate school, a new professor, Peter Saulson, joined Syracuse University, and he talked about LIGO and how you could measure these distortions in space-time. And putting that together — the measurement of things that are happening here on Earth, next to you, that are a ripple in space-time and that bring you information from black holes millions of light-years away — it was irresistible. I said, that’s what I want to do. So that’s what I did. The fact that we can measure this distortion that is so fundamental to nature, that is what inspires me all the time.
What types of objects are you looking for?
Neutron stars are the most compact stars other than black holes. We know that they exist in pairs and that they spin closer and closer together. They lose energy in the form of gravitational waves, and when they merge, they form a black hole. We also think that there could be lots of medium-size black holes that do this dance and coalesce to form big black holes. So those binary systems are the sources we are most likely to detect first.
We also look for a stochastic background [a noiselike hiss of gravitational waves] that we believe to have formed shortly after the Big Bang. This gravitational-wave background exists at all frequencies. It’s probably too small for us to see, but we’ll look for that kind of background, too.
There have been rumors that Advanced LIGO has already detected gravitational waves.
There’s really not anything we can say because it takes months to understand how the detector is performing. But we have only been operating the detector for a month now. There’s no way to know anything yet about the data with any confidence.
How does Advanced LIGO look for gravitational waves?
To me, that’s the most amazing thing. LIGO is such a complex but precise instrument. It’s an interferometer, which means that we have a laser source that we split in two with a beam splitter. We have two beams that travel four kilometers down perpendicular arms of the machine, find mirrors at the end, get reflected back, and meet again. We can tell the slight difference in distance that these light beams traveled.
The idea is that gravitational waves are distorting space-time so that one arm is getting shorter while the other is longer. That would produce a phase difference in the beams as they come back to our detector. We see it as a change in the amount of light. If there are no gravitational waves, when the beams come back they are exactly out of phase and you don’t detect light. But if there is a gravitational wave, then you will detect some light.
And, just to give the scale of this, these light beams travel four kilometers and we can detect a difference of less than a proton’s diameter in that distance. In fact, at our best we are doing one part in 10,000 of a proton diameter.
William Widmer for Quanta Magazine
Video: Gabriela González explains how to measure black-hole collisions using gravitational waves.
How is it possible to detect a length of less than one-ten-thousandth of the diameter of a proton?
The way I described it, it sounds simple. It’s a beam splitter, a laser and two mirrors. But there are thousands and thousands of things that need to be controlled. The distance is always changing because the mirrors are moving. We need a lot of tricks to make sure the atomic motion, the Brownian motion, of these mirrors does not limit us too much. This all has to be done in a vacuum. You are counting photons and there is a quantum uncertainty in the number of photons. The very hard work in the field has been reducing those sources of noise to get the sensitivity that we have now.
Is that the difference between the initial LIGO and Advanced LIGO?
It’s amazing, because if you look at it from the outside, it looks the same. It’s the same observatory, the same vacuum chambers. But on the inside, everything is different. We have a laser that’s 10 times as powerful, with more stabilization and more features. In initial LIGO, we had mirrors hanging from a single suspension, which in turn was hanging from “shock absorbers” to reduce seismic noise. In Advanced LIGO, we have mirrors in a quadruple suspension hanging from an active seismic isolation system. It’s a system that has lots of sensors and pushers — we call them actuators — that cancel the noise. So they measure the way the ground is moving and then they cancel it.
How much of the universe will Advanced LIGO be able to observe?
We refer to the sensitivity of our detectors by how sensitive they are to binary neutron star systems. With initial LIGO we could detect these systems up to 20 megaparsecs away. [A megaparsec is about 3.3 million light-years.] Now we can see 60, 70, sometimes even 80 megaparsecs away. But we think that Advanced LIGO detectors should, with all the bells and whistles, reach 200 megaparsecs.
And will this sensitivity allow Advanced LIGO to detect gravitational waves?
With a sensitivity of 200 megaparsecs in two detectors, we expect a rate of tens of events per year. If we are at 150 megaparsecs, then we might see a few a year. But that’s already more than one!
The first Advanced LIGO run is scheduled for only a few months, right?
That’s right, because we want to improve the detectors quite a bit more. There are some [astronomical] models that predict that we will see things very quickly, so I’m hoping they’re right. Next year we are planning to take six months of data. And then every year we will have better sensitivity, longer runs, until we see something.
There will be a lot of analysis once you have a detection.
Yes, it will take a lot of work and a lot of time. Over the last few months we have been trying to think ahead to the steps we will go through if we have a hint of a detection. How do we validate it? How do we prepare? How do we discuss it in a large collaboration? We have 900 people. We have a detection procedure, and we try to estimate how long it would take. Our optimistic estimate is three months.
What are the long-term goals for gravitational-wave astrophysics?
I think in the next few years we will have detections. But there will only be a few, and in astronomy, people are used to much bigger numbers. So in 10 to 15 years, I see bigger facilities that probably would need several countries collaborating to build. I see myself very involved in working in those.
There’s a space-based project that the European Space Agency has assigned to launch in 2034. I’m really hoping that with the discovery of gravitational waves from ground-based detectors, that project can progress a lot faster. Of course, space projects always take time. From that point on, I think there will be an explosion in all the different wavelengths of gravitational-wave astronomy. It’ll be like light. It’s going to be a spectrum of gravitational waves.
There’s a poetic notion that once gravitational waves are observed, they will open a new window into the universe.
This is definitely opening a new window, but it’s not just poetry, it’s science. For example, one of the things that we expect to see — it probably will not be the first or maybe even the 10th, but it will come eventually — is gravitational waves from the merging of two neutron stars, or a neutron star and a black hole, which we also detect as a gamma-ray burst. Gamma-ray bursts are detected very often, and there are two kinds: short and long. The short ones are from these mergers. These are two very different windows: gamma rays and gravitational waves.
What will the first gravitational-wave detection mean to you?
There are so many expectations riding on that first event. Once we have one, others will follow. And the real science — knowing how many black holes there are, how many neutron stars, what the composition of neutron stars is — all that science will come from many detections, not the first one. I am really looking forward to going past that first event.
This article was reprinted on Wired.com.