An Explorer of Quantum Borderlands
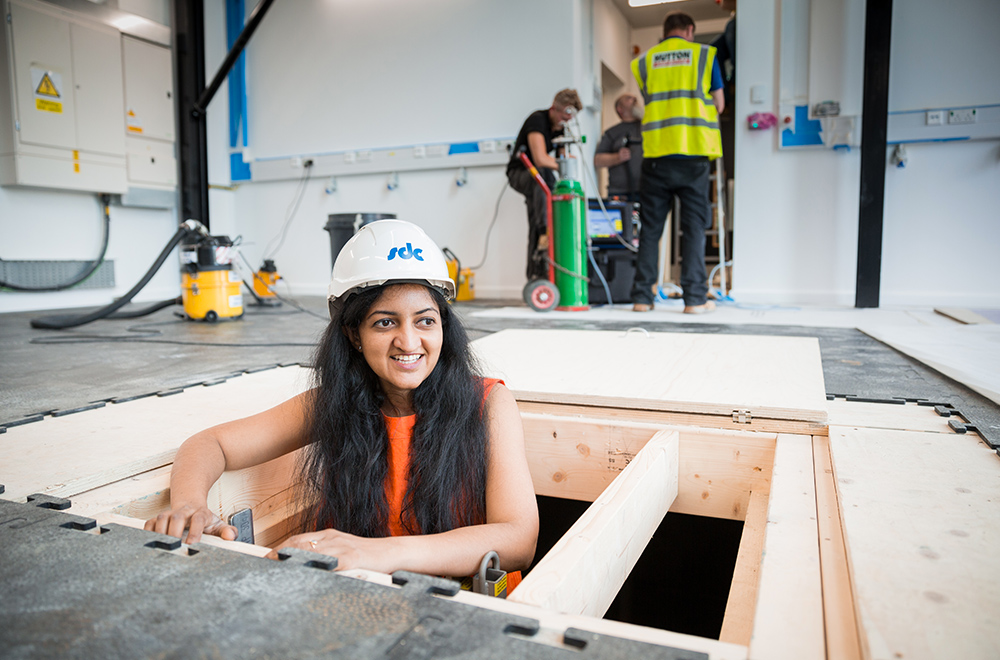
Suchitra Sebastian is a fringe physicist. Not a crackpot — she lectures at the University of Cambridge and has published a string of papers in Science and Nature. But she likes to venture into the borderlands between forms of matter that other physicists have already explored. There, in the liminal space where the particles in a material begin to change from one configuration to another, new quantum effects appear. “A lot of it is really exciting phenomena that emerges before it’s theoretically predicted,” Sebastian said with delight.
Last year, she and her colleagues discovered what appeared to be electrons looping their way through an insulator, a type of material that by definition prevents such movement. The observation, in a substance called samarium hexaboride, is still not understood. But Sebastian says one possibility is that what was looping was not electrons but an entirely new kind of subatomic building block.
Interactions between electrons create wavelike disturbances — known as quasiparticles — that serve as the basic components of almost every complex material. The known quasiparticles tend to act like heavier versions of electrons, but not so in this case. “In samarium hexaboride, the possibility is that the electron itself has broken apart,” said Sebastian. “So instead of thinking of the electron as the building block, we would need to think of fractional parts of the electron as building blocks.” These fractional quasiparticles would create an entirely new way to understand the universe of materials.
Sebastian herself moves between very different worlds. Before delving into science, she worked as a management consultant, and now she performs in experimental theater pieces when she’s not in the lab. “I kind of intensely do different things,” she says. “If I spend too much time doing the analytical physics side, I’m, like, gasping for oxygen.” Research, she said, “is not about drawing within the lines. It’s about discovery and creativity.”
Quanta Magazine spoke with Sebastian about her research and her unconventional path to science. An edited and condensed version of the interview follows.
QUANTA MAGAZINE: You search for quantum effects that are entirely new to science. How do you go about looking for them?
SUCHITRA SEBASTIAN: One option is to look at many, many, many, materials. You can say, “I’m trying to find the one that does something very different.” You may never find it. What I realized is you can use external conditions — pressures or temperatures or magnetic fields — to manipulate a material and move it into a region where it does something really interesting and where new quantum properties emerge.
Where is that magical region?
Water can be water, or it can be ice, or it can be steam. These are the same material but in different phases. In the quantum world you can also have different phases. You can have the same material and the same electrons, but the interactions can result either in the substance organizing into one kind of material — so under certain conditions, you can have a magnet — or you exert pressure on the material — you press it — and then it quantum configures in a slightly different way and the magnet transforms into a superconductor. The region I’m excited about is the region between these phases, which is a quantum critical region. Between one phase and another phase you get this intermediate region, where it’s unknown what might happen, and you can have completely new forms of matter emerging.
What gives rise to the quantum effects?
Simple materials with weak electron interactions can be modeled just in terms of the electrons’ propensity to hop around, which can be averaged over the entire material. But in more strongly interacting materials, the repulsive force due to interactions between each of the trillions and trillions of electrons is stronger than their propensity to hop around. In this case, the resulting collective effects are almost impossible to predict and can be dramatically different from individual electron behavior.
Last year, you found an unexpected quantum effect in a material called samarium hexaboride. What was so surprising?
We think of metals as carrying electricity, and insulators as not. Electrons in metals are traveling long distances, and they’re carrying charge — it’s how electricity flows. In insulators, electrons are largely stuck in one position. That’s why electricity is not being transported in insulators. Samarium hexaboride was predicted to be in a class of materials known as topological insulators, where current flows only on the surface and not in the bulk [the material’s interior].
We were really, really shocked when the magnetization we measured in the sample started showing wiggles that are characteristic of an electron executing orbits. We see these electrons that travel really long orbits, and they’re coming from the bulk. But the bulk is insulating; those electrons are barely moving. How is it conceivable they’re traveling in these large orbits?
What might be going on?
We assume it’s the electrons that are traveling in these orbits, but at the same time, the electrons can’t possibly be moving because there’s no charge moving.
Maybe what’s happening is that when the electrons come together in this quantum ensemble, we cannot describe the physics in terms of the individual electrons anymore. The possibility is that the electron itself has broken apart. So instead of thinking of the electron as the building block, we would need to think of fractional parts of the electron as building blocks.
One new finding in support of this is that we do have heat transport through this material, but there are no charges being transported. So maybe you need to think about particles that carry [the quantum property of] spin — which carry heat — and not charge. One possibility is a type of neutral quasiparticle known as a spinon, which carries spin and not charge.
If it turned out that the electron was no longer the basic building block, that would be very shocking. You can count on the fingers of one hand how many such cases there are.
Why would it be so shocking?
It’s always shocking when we discover the fundamental building block of matter isn’t what we thought it was. Originally we thought the fundamental building block was the atom. Then subatomic particles — neutrons, protons and electrons — were discovered.
When we describe complex materials, we think of the fundamental building block as the electron. One of the overarching quests in condensed matter physics is to find a material that behaves radically differently, as though the electron has broken down, which means we need a new description — not in terms of electronlike quasiparticles but in terms of quasiparticles based on fractional components of an electron.
One of the clearest examples is the fractional quantum Hall effect, in which instead of an electron that carries charge as the building block, one observes the fundamental building block to be fragments of an electron that carry fractional charges.
Philipp Ammon for Quanta Magazine
You have also created a superconductor — a material that transmits electricity without any resistance — by squeezing a magnet with a diamond anvil, a process you call “quantum alchemy.” What strategies do you use to search for new types of superconductors?
Thus far, if you look at existing superconductors, they’re often close to being magnetic, and they’re often close to being insulating. Another promising feature is layered materials, so that rather than being cubic in their crystal structure, the materials are quite two-dimensional — their crystal structure is stretched out.
What’s important is to take materials with these promising properties, then apply pressure and go from a non-superconductor into a superconductor. You’re probably not going to start with an optimal high-temperature superconductor by doing this. But if we can make several superconductors from different families of materials, then you’re going to be able to start making a road map: “Now I know these properties of this material gave me a superconductor, but it wasn’t as good as this other one where I started with a material with these other properties.” When you start finding patterns, that will give you a good bridge to then pursue the ultimate optimization of superconductivity.
What would this optimization lead to, practically?
One of the big implications of a room-temperature superconductor would be that you could transport energy over very long distances — from anywhere in the world to anywhere else — without any loss. Renewables will be a big part of the future for sustainable energy. What’s not always recognized is that we also need to transport the renewable energy. Solar energy will be abundant in the Sahara, but the most populous cities are, for instance, in New York. How are you going to transport energy over thousands of miles? If you wave a magic wand and get to ideal superconductors, a really long cable would take energy from where it’s created to where it’s most needed. We could start thinking of renewable energy in a more holistic way. You could start thinking more of a worldwide grid.
Is science doing enough to find more superconductors?
I don’t think there is a very big effort to search for new superconductors. There are these two questions: How do we understand copper oxide superconductors, which are the one known family of materials that superconduct above liquid-nitrogen temperatures at ambient conditions? And how do we create new superconductors? My impression is about 90 percent of the field’s effort is focused on how to understand copper oxide superconductors. That is a really interesting and important area, but I think there is way too little effort and interest in how to make new superconductors. Personally I feel that scientists should be partnering with industry, or partnering with organizations that are familiar with the idea of assembly-line processes, to accelerate this process of discovery. It’s such an important problem.
You have some experience working with industry. After studying physics in college, you went on to do an MBA and work as a management consultant for several years before returning to physics. Why did you leave science for a while?
As much as I love physics and I’m passionate about it, I think the field itself is quite insular, and it does tend to be not very diverse. I didn’t identify with the kind of people I saw doing physics. They didn’t seem very fun or interesting. It seemed like they were really locked into these little worlds, and they knew a lot about what they did, but I felt like they were totally out of touch with the rest of the world. I really need to be engaged with everything around me in different ways. How does the world work, how does economics work, how do governments run? I’m interested in the social implications of what we do. I actually applied to an MBA, I applied to physics, I applied to engineering, and I applied to literature. I interviewed for all of them, and the MBA interview — it was quite an interesting interview. This is how I made my decision.
But you didn’t enjoy consulting in the end?
They sell it to you as out-of-the-box thinking and meeting all these interesting people in different fields. But doing it I realized it is not that out-of-the-box thinking. The longer you stay in it, the less it becomes about discovering solutions and the more it becomes about trying to network and market the same old solutions.
But I will say that I think that is why I recognize how important communication is and how important it is to get your audience on board. I think scientists are sometimes quite complacent in thinking they know a lot about something very specific, and really, if someone doesn’t understand, that’s their problem. But if you’re making a pitch and your client doesn’t buy it, that is your problem. It’s up to you to learn to make a compelling case so you bring people on board. The difference really was, in my consulting work, I wasn’t passionate enough about the solution I was pitching to want to do it the rest of my life. It’s nice, but does it change the world? No. But with physics, I’m passionate about it. It has the potential to revolutionize the world.
So did you find fulfillment with physics?
I think it took time to find my own way. I think I needed all those experiences. When I came back to physics, now I’m able to keep all the different parts of me and develop them. Now I can be a more balanced person and recognize that I can do physics without being that stereotypical physicist.
Physics isn’t just about writing equations on a board and sitting in front of a computer. Science is about exploring new worlds. There are lots of people who would approach the world in that way, but they don’t recognize that physics is about that. We need to attract more of these people to physics.
You have said, “Who I am is at the heart of the science I do.” Can you explain that?
I think often people have this impression that the science you do is removed from the person you are. It’s almost like people are swappable. I feel like in physics they don’t really recognize that the person you are is integral to the science you do. The way different people do physics is completely different. I choose to do extremely exploratory physics where I deliberately choose problems where I don’t know what the answer is going to be. But I recognize that for other people, they need to do the kind of physics where you have to be incredibly careful and take years setting something up because you’re looking for the 10th digit to prove a hypothesis. In order to learn more about the world around us, you need to come at it in different ways.
How have you tried to draw more people to science?
I’ve done things called soapbox talks before. We go into public spaces — it’s like science busking. It’s literally upturned wooden boxes, and a few people engage with pedestrians walking by, saying, “Do you want to learn about some cool science?” People are really excited about it.
In your free time, you do theater, and you just spearheaded an art exhibit to celebrate the opening of a new science building at the University of Cambridge. Do you plan to do more art-science events?
Engaging through the arts is really new for me. But I’m really excited about how well it worked the first time. We are having conversations about taking it forward. Creativity happens when you bring together disparate worlds. If you just do the one thing, and you meet people very similar to you, I think this often reinforces certain ways of thinking and deepens ruts. But where does creativity come from? It comes when you have different approaches intersecting. I think physical spaces are really, really important for this — where people come together, where you have chance encounters. I just really think it’s important to have these interfaces and porous boundaries to break down any kind of siloing.
This article was reprinted on Wired.com.