A Missing Genetic Link in Human Evolution
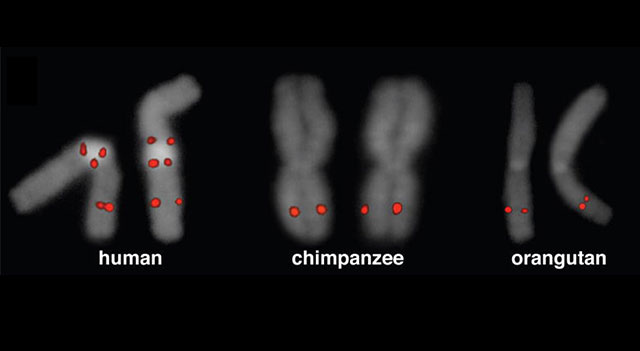
About 8 million to 12 million years ago, the ancestor of great apes, including humans, underwent a dramatic genetic change. Small pieces of DNA replicated and spread across their resident chromosomes like dandelions across a lawn. But as these “dandelion seeds” dispersed, they carried some grass and daisy seeds — additional segments of DNA — along for the ride. This unusual pattern, repeated in different parts of the genome, is found only in great apes — bonobos, chimpanzees, gorillas and humans.
“I think it’s a missing piece of human evolution,” said Evan Eichler, a geneticist at the University of Washington, in Seattle. “My feeling is that these duplication blocks have been the substrate for the birth of new genes.”
Over the past few years, scientists have begun to uncover the function of a handful of genes that reside in these regions; they seem to play an important role in the brain, linked to the growth of new cells, as well as brain size and development. In September, Eichler’s team published a new technique for analyzing how these genes vary from person to person, which could shed more light on their function.
Much about the duplication process — and its implications — remains a mystery. Eichler and others don’t know what spurred the initial rounds of duplications or how these regions, dubbed “core duplicons,” reproduced and moved around the genome.
Despite the duplication-linked genes’ potential importance in human evolution, most have not been extensively analyzed. The repetitive structure of the duplicated regions makes them particularly difficult to study using standard genetic approaches — the most efficient methods for sequencing DNA start by chopping up the genome, reading the sequence of the small chunks and then assembling those sections like one would a puzzle. Trying to assemble repetitive sections is like trying to put together a puzzle made of pieces with almost the same pattern.
“Because these regions are so complex, they are often ignored by conventional genome studies, and some regions still haven’t been fully sequenced,” said James Sikela, a geneticist at the University of Colorado School of Medicine in Aurora. “So not only are they important, they are unfortunately unexamined.”
A Genetic Burst
In 2007, Eichler and his collaborators took on what seemed like a herculean task — looking comprehensively at the repetitive stretches of the human genome. Previous studies had characterized individual regions, but Eichler’s team employed new computational techniques and comparative genomics — comparing DNA sequences from different species — to examine the entire genome. Mathematical analysis published in Nature Genetics that year revealed a set of “core duplicons” — stretches of DNA that appear over and over on a specific chromosome.
The core duplicon anchors an architecturally complex stretch of DNA, acting as the focal point for a larger block of duplications. Although scientists aren’t sure how, the core seems to sweep up neighboring segments of DNA, duplicating the entire stretch and inserting the new copy into a new location on the chromosome. “Then it picks up again and duplicates some of the sequence around it and moves to another new location,” Eichler said. “It seems to be an extremely unstable genetic element that provides a template for evolutionary change.”
It is this process that appears to create new genes: When new duplications are inserted into the genome, they bring together two previously foreign pieces of DNA, which can lead to new functional components, such as proteins. This chaotic mix-and-match approach is different from the traditional model for the creation of a gene, in which an existing gene is duplicated and the copy is free to develop new functions.
“This mechanism appears to be seminal in our evolution,” said Philip Hastings, a geneticist at Baylor College of Medicine, in Houston. “It’s possible that we are the way we are largely because of this mechanism that generates dramatic episodes of chromosomal structural change.”
The pattern that the duplicons create seems to be unique to great apes, suggesting the mechanism itself is also unique to these species. In other animals, duplicated regions are lined up next to one another rather than dispersed along the chromosome.
The duplicated regions in great apes tend to be very active, meaning that their genes are turned on more often than genes in other areas and that they are producing more RNAs and proteins. That suggests these regions are functionally important.
Eichler and others have so far characterized the structure of only about half of the roughly dozen duplicon regions, each of which is unique to their resident chromosome. Most of the analysis to date has focused on the region’s evolutionary history, including where the genes came from, how quickly they are evolving and how they are related to one another. Eichler said his team has had a more difficult time understanding what they do, although he and others have managed to study the function of a handful of the duplication-linked genes.
What the scientists do know is that the genes appear to be important in evolution. According to Eichler, about a third of the gene families linked to core duplicons show signs of positive selection — meaning that they boost survival of their bearers and are passed on to the next generation, contributing to evolution — compared to about 5 percent of genes overall. Indeed, a gene on one of the cores, first described more than 10 years ago, appears to be the fastest-evolving human gene. However, Eichler cautioned, it’s tricky to measure positive selection in these human or ape-specific genes because scientists have little to compare them to. To measure selection, scientists typically compare a gene in different species to examine how much it has changed.
Much like the rings of a tree trunk, the outer regions of core duplicons are the newest, arising from the latest round of replications. These regions also tend to be the most variable from person to person. They may therefore contribute to disease — extra or deleted copies of important genes or DNA segments are likely to affect how well cells or organs function. Eichler’s team aims to use the new approaches described in their September paper in Nature Methods to track variation in duplicated regions, which could give insight into what these genes do. The researchers will look for variations in 30 human-specific genes within these zones in children with developmental disorders such as intellectual disability and epilepsy. If changes in a certain gene or region are reliably linked to specific traits, like a change in brain size, it gives hints to the gene’s function.
Duplication-linked genes studied to date “seem to be important for cell proliferation, either speeding it up or slowing it down,” Eichler said. “They are expressed in many tissues but highly in the brain, often in neurons, and often in areas of rapid cell division.” In fact, some of the genes have been linked to cancer when they become overactive.
Bigger Brains
About 3.4 million years ago, a core duplicon on what is now called chromosome 1 in human descendants made one of its characteristic jumps, taking with it a copy of a gene known as SRGAP2. A million or so years later, it jumped again, creating a granddaughter of the original. No other mammals whose genomes have been examined to date have multiple copies of the gene, and the jumps coincide with a pivotal point in human evolution: As Australopithecus evolved into Homo habilis 2 to 3 million years ago, hominid brains were on their way to doubling in size.
The granddaughter gene, known as SRGAP2C, may be particularly important for the human brain. In 2012, Eichler’s team and a group from the Scripps Research Institute near San Diego showed that SRGAP2C can influence how neurons migrate in a developing brain. By expressing the human version of the gene in mice, the Scripps team showed that SRGAP2C slows the maturation of certain brain cells and triggers the development of a denser array of neuronal structures called spines, which help form connections between brain cells. “I’m not saying it’s responsible for the expansion of the human brain, but it might play a role in getting neural precursors [cells that give birth to neurons] to the right place,” Eichler said.
Deciphering Duplications
Figuring out the function of human-specific genes will help uncover how they have contributed to our evolution. But this research presents a challenge. One of the standard approaches to deciphering what a gene does is to remove it in model organisms — mice, flies or worms — and see what goes wrong. But that’s impossible if the animals don’t have the gene in the first place. Genetically engineering a human version into mice, as researchers did for their experiment with the duplicated gene SRGAP2C, provides one alternative. But given that these genes are probably involved in brain development, it might be impossible to study their full repertoire in mice, which lack the wrinkled cortex characteristic of the human brain or the rich range of human behavior.
Evan Eichler, a geneticist at the University of Washington, in Seattle, and collaborators say one way around this issue is to study the genes in humans, looking for natural variation in the population and determining whether these differences are linked to specific disorders or cognitive abilities. In an unpublished study of SRGAP2C in nearly 3,000 people with intellectual disability, the researchers found five people who had lost it completely. Two of them have a smaller version of part of the brain called the orbitofrontal cortex, which is involved in functions such as decision-making. The numbers in the study aren’t yet statistically significant, “but it’s encouraging that we now have the ability to systematically probe these regions,” Eichler said.
The SRGAP2 findings show how a human-specific genetic change led to changes in neurons. “That’s what has been missing from the field,” said Genevieve Konopka, a neuroscientist at the University of Texas Southwestern Medical Center, in Dallas. “People have identified unique changes in the human lineage, but they haven’t really followed up on them in any functional manner.”
Konopka said studies such as the SRGAP2 paper, which delve into the function of a gene, can help to clarify the role that human-specific genetic changes play in our development as a species. “Anytime you can show something is unique in the human genome and how it modifies the biology, that is a unique and important thing to do,” she said.
Double-Edged DNA
Core duplicons represent something of an evolutionary gamble. The same genetic instability that enables the creation of new genes may also destroy or delete existing ones or create too many copies, perhaps explaining some of our susceptibility to disease. Parts of the duplicated blocks have been tied to a number of brain disorders, including intellectual disability, schizophrenia and epilepsy.
When researchers searched for genetic regions that had been duplicated more often in humans than other great apes, a short stretch of DNA called DUF1220 caught their attention. DUF1220 has duplicated in humans more rapidly than any other protein-coding region of the genome and has been linked to brain size, suggesting it contributed to the evolution of the human brain, and disease, suggesting that either too little or too much of this gene segment can be harmful.
DUF1220 is not a gene itself, but rather a genetic component found in a family of genes — individual genes in the family carry 5 to 50 copies. Overall, humans have more than 250 copies of DUF1220, other great apes 90 to 125, monkeys about 30, and non-primates fewer than 10. In 2012, Sikela and collaborators used special tools to count the number of copies in healthy people and discovered that the more copies someone has, the larger their gray matter, the portion of the brain made up of nerve cells.
Although DUF repeats appear to provide an evolutionary advantage in terms of brain size, they may have harmful effects as well. DUF duplications are concentrated in an unstable region of chromosome 1 known as 1q21. Deletion or duplication of this region has been linked to a number of disorders, including autism, schizophrenia, heart disease and microcephaly or macrocephaly, when someone’s brain is abnormally small or large, respectively. Sikela’s team found that of all the sequences in the 1q21 region, the number of DUF1220 repeats is the most tightly linked to brain size in people with microcephaly. “The big increase in copy number in the human lineage has come at severe cost,” Sikela said.
According to Sikela, the additional copies render the region unstable, making it more likely that genes in the area can be further deleted or duplicated. “It’s the price we have to pay for the benefit of DUF, a trade-off given to us by evolution,” he said.
Uncharted Territory
Human Variation
Human-specific genes are both the newest additions to the duplicon blocks and the most variable. For example, humans carry different numbers of copies of a gene known as TBC1D3. Europeans tend to have few copies, and Africans from certain areas have many, with a tenfold difference among human populations. By altering gene activity in human cell lines, Philip Stahl, a cell biologist at the Washington University School of Medicine, and his collaborators have shown that TBC1D3 is involved in cell growth and proliferation. “Now we know the rough outlines of what [TBC1D3] is doing, but we don’t know why it’s so variable,” Stahl said. “Does it relate to nutrition? Reproduction? Brain development? If we can figure out what it’s doing, it would be a very interesting evolutionary story.”
While evidence that core duplicons are a driving force behind human evolution is growing, many questions remain. For example, it’s unclear what triggered the creation of these cores or how they spread. One popular theory points to a class of viruses known as retroviruses, which can insert DNA into their host’s genome that is then passed from generation to generation. Perhaps a retrovirus was responsible for the initial core duplicons. A significant portion of our genome is known to arise from viruses that have left the imprint of their DNA but are no longer active in our cells. “My favorite hypothesis is that at a key point in great ape evolution, there was a burst in retroviral activity,” said Edward Hollox, a geneticist at the University of Leicester, in Great Britain.
Intriguingly, the core duplicons once so active in our genomes seem to have slowed or stopped hopping. Despite evidence for several spurts in the great ape evolutionary history, scientists have yet to find duplications that occurred in the past few million years. Eichler’s team has searched for such cases, finding some younger duplications that the scientists think are specific to humans and distinct from Neanderthals. “But they are the exception rather than the rule,” he said.
It’s not yet clear how big a role core duplicons played in the formation of our species. “It’s very difficult to provide an overarching theory of great ape evolution,” Hollox said. “Undoubtedly, the core duplicon hypothesis is part of it. To what extent it contributes, the jury is still out.”
Research suggests other factors such as gene regulation — when and where specific genes are turned on — play a part as well. But changes in gene regulation are probably not sufficient to explain all the differences between primates and humans. “I know there are going to be multiple paths to brain evolution,” Konopka said, adding that core duplicons are “probably one of the main players.”
This article was reprinted on ScientificAmerican.com.