A New Spin on the Quantum Brain
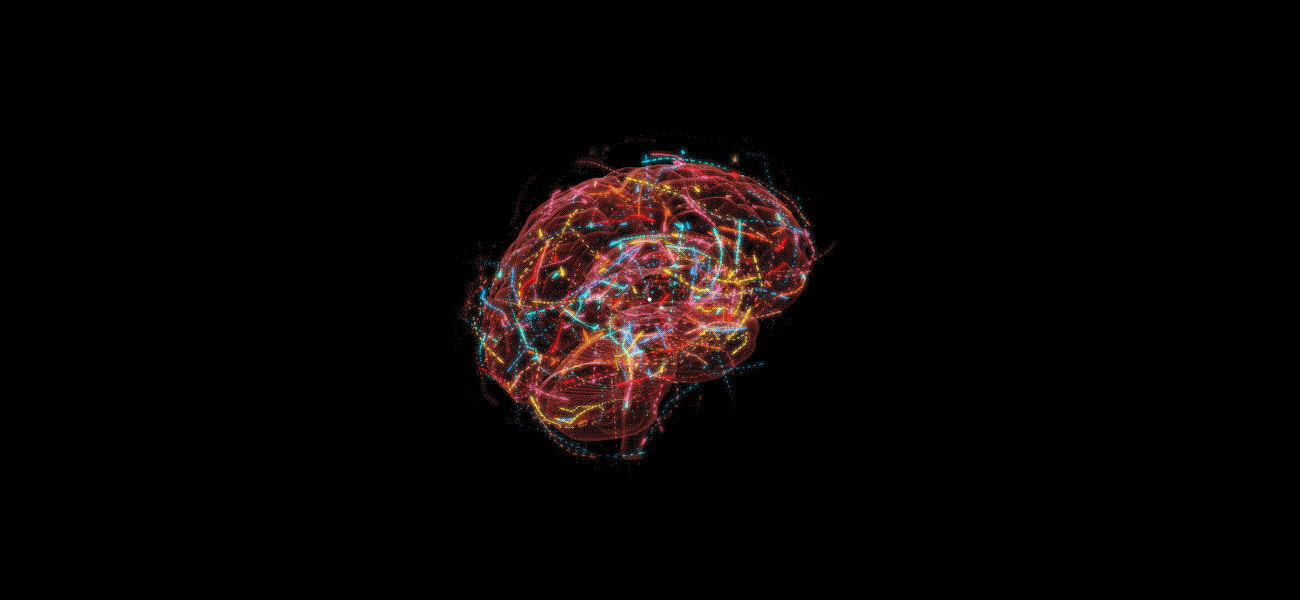
davidope for Quanta Magazine
Introduction
The mere mention of “quantum consciousness” makes most physicists cringe, as the phrase seems to evoke the vague, insipid musings of a New Age guru. But if a new hypothesis proves to be correct, quantum effects might indeed play some role in human cognition. Matthew Fisher, a physicist at the University of California, Santa Barbara, raised eyebrows late last year when he published a paper in Annals of Physics proposing that the nuclear spins of phosphorus atoms could serve as rudimentary “qubits” in the brain — which would essentially enable the brain to function like a quantum computer.
As recently as 10 years ago, Fisher’s hypothesis would have been dismissed by many as nonsense. Physicists have been burned by this sort of thing before, most notably in 1989, when Roger Penrose proposed that mysterious protein structures called “microtubules” played a role in human consciousness by exploiting quantum effects. Few researchers believe such a hypothesis plausible. Patricia Churchland, a neurophilosopher at the University of California, San Diego, memorably opined that one might as well invoke “pixie dust in the synapses” to explain human cognition.
Fisher’s hypothesis faces the same daunting obstacle that has plagued microtubules: a phenomenon called quantum decoherence. To build an operating quantum computer, you need to connect qubits — quantum bits of information — in a process called entanglement. But entangled qubits exist in a fragile state. They must be carefully shielded from any noise in the surrounding environment. Just one photon bumping into your qubit would be enough to make the entire system “decohere,” destroying the entanglement and wiping out the quantum properties of the system. It’s challenging enough to do quantum processing in a carefully controlled laboratory environment, never mind the warm, wet, complicated mess that is human biology, where maintaining coherence for sufficiently long periods of time is well nigh impossible.
Over the past decade, however, growing evidence suggests that certain biological systems might employ quantum mechanics. In photosynthesis, for example, quantum effects help plants turn sunlight into fuel. Scientists have also proposed that migratory birds have a “quantum compass” enabling them to exploit Earth’s magnetic fields for navigation, or that the human sense of smell could be rooted in quantum mechanics.
Fisher’s notion of quantum processing in the brain broadly fits into this emerging field of quantum biology. Call it quantum neuroscience. He has developed a complicated hypothesis, incorporating nuclear and quantum physics, organic chemistry, neuroscience and biology. While his ideas have met with plenty of justifiable skepticism, some researchers are starting to pay attention. “Those who read his paper (as I hope many will) are bound to conclude: This old guy’s not so crazy,” wrote John Preskill, a physicist at the California Institute of Technology, after Fisher gave a talk there. “He may be on to something. At least he’s raising some very interesting questions.”
Senthil Todadri, a physicist at the Massachusetts Institute of Technology and Fisher’s longtime friend and colleague, is skeptical, but he thinks that Fisher has rephrased the central question — is quantum processing happening in the brain? — in such a way that it lays out a road map to test the hypothesis rigorously. “The general assumption has been that of course there is no quantum information processing that’s possible in the brain,” Todadri said. “He makes the case that there’s precisely one loophole. So the next step is to see if that loophole can be closed.” Indeed, Fisher has begun to bring together a team to do laboratory tests to answer this question once and for all.
Finding the Spin
Fisher belongs to something of a physics dynasty: His father, Michael E. Fisher, is a prominent physicist at the University of Maryland, College Park, whose work in statistical physics has garnered numerous honors and awards over the course of his career. His brother, Daniel Fisher, is an applied physicist at Stanford University who specializes in evolutionary dynamics. Matthew Fisher has followed in their footsteps, carving out a highly successful physics career. He shared the prestigious Oliver E. Buckley Prize in 2015 for his research on quantum phase transitions.
So what drove him to move away from mainstream physics and toward the controversial and notoriously messy interface of biology, chemistry, neuroscience and quantum physics? His own struggles with clinical depression.
Fisher vividly remembers that February 1986 day when he woke up feeling numb and jet-lagged, as if he hadn’t slept in a week. “I felt like I had been drugged,” he said. Extra sleep didn’t help. Adjusting his diet and exercise regime proved futile, and blood tests showed nothing amiss. But his condition persisted for two full years. “It felt like a migraine headache over my entire body every waking minute,” he said. It got so bad he contemplated suicide, although the birth of his first daughter gave him a reason to keep fighting through the fog of depression.
Eventually he found a psychiatrist who prescribed a tricyclic antidepressant, and within three weeks his mental state started to lift. “The metaphorical fog that had so enshrouded me that I couldn’t even see the sun — that cloud was a little less dense, and I saw there was a light behind it,” Fisher said. Within nine months he felt reborn, despite some significant side effects from the medication, including soaring blood pressure. He later switched to Prozac and has continuously monitored and tweaked his specific drug regimen ever since.
His experience convinced him that the drugs worked. But Fisher was surprised to discover that neuroscientists understand little about the precise mechanisms behind how they work. That aroused his curiosity, and given his expertise in quantum mechanics, he found himself pondering the possibility of quantum processing in the brain. Five years ago he threw himself into learning more about the subject, drawing on his own experience with antidepressants as a starting point.
Since nearly all psychiatric medications are complicated molecules, he focused on one of the most simple, lithium, which is just one atom — a spherical cow, so to speak, that would be an easier model to study than Prozac, for instance. The analogy is particularly appropriate because a lithium atom is a sphere of electrons surrounding the nucleus, Fisher said. He zeroed in on the fact that the lithium available by prescription from your local pharmacy is mostly a common isotope called lithium-7. Would a different isotope, like the much more rare lithium-6, produce the same results? In theory it should, since the two isotopes are chemically identical. They differ only in the number of neutrons in the nucleus.
When Fisher searched the literature, he found that an experiment comparing the effects of lithium-6 and lithium-7 had been done. In 1986, scientists at Cornell University examined the effects of the two isotopes on the behavior of rats. Pregnant rats were separated into three groups: One group was given lithium-7, one group was given the isotope lithium-6, and the third served as the control group. Once the pups were born, the mother rats that received lithium-6 showed much stronger maternal behaviors, such as grooming, nursing and nest-building, than the rats in either the lithium-7 or control groups.
This floored Fisher. Not only should the chemistry of the two isotopes be the same, the slight difference in atomic mass largely washes out in the watery environment of the body. So what could account for the differences in behavior those researchers observed?
Fisher believes the secret might lie in the nuclear spin, which is a quantum property that affects how long each atom can remain coherent — that is, isolated from its environment. The lower the spin, the less the nucleus interacts with electric and magnetic fields, and the less quickly it decoheres.
Because lithium-7 and lithium-6 have different numbers of neutrons, they also have different spins. As a result, lithium-7 decoheres too quickly for the purposes of quantum cognition, while lithium-6 can remain entangled longer.
Fisher had found two substances, alike in all important respects save for quantum spin, and found that they could have very different effects on behavior. For Fisher, this was a tantalizing hint that quantum processes might indeed play a functional role in cognitive processing.
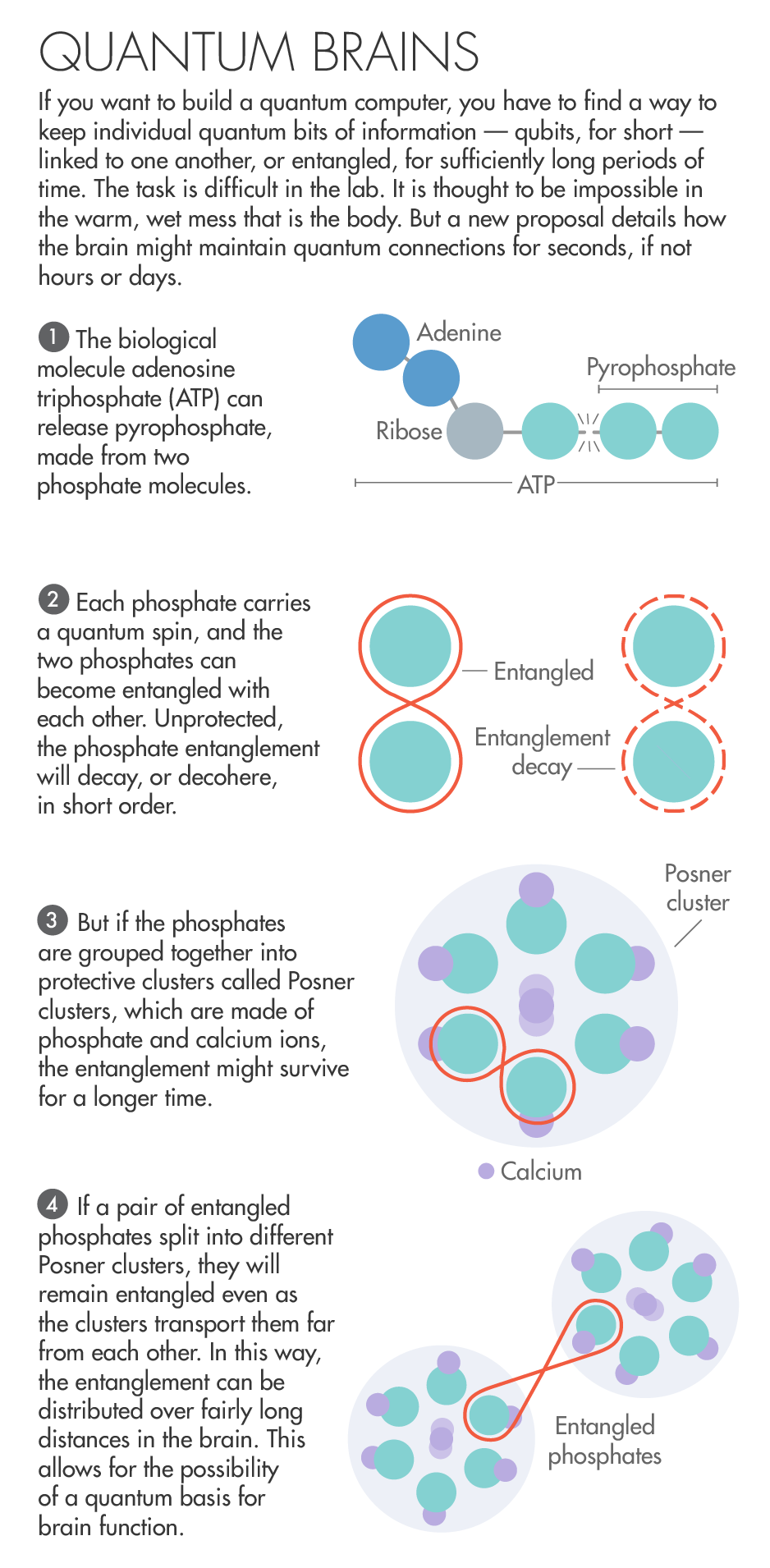
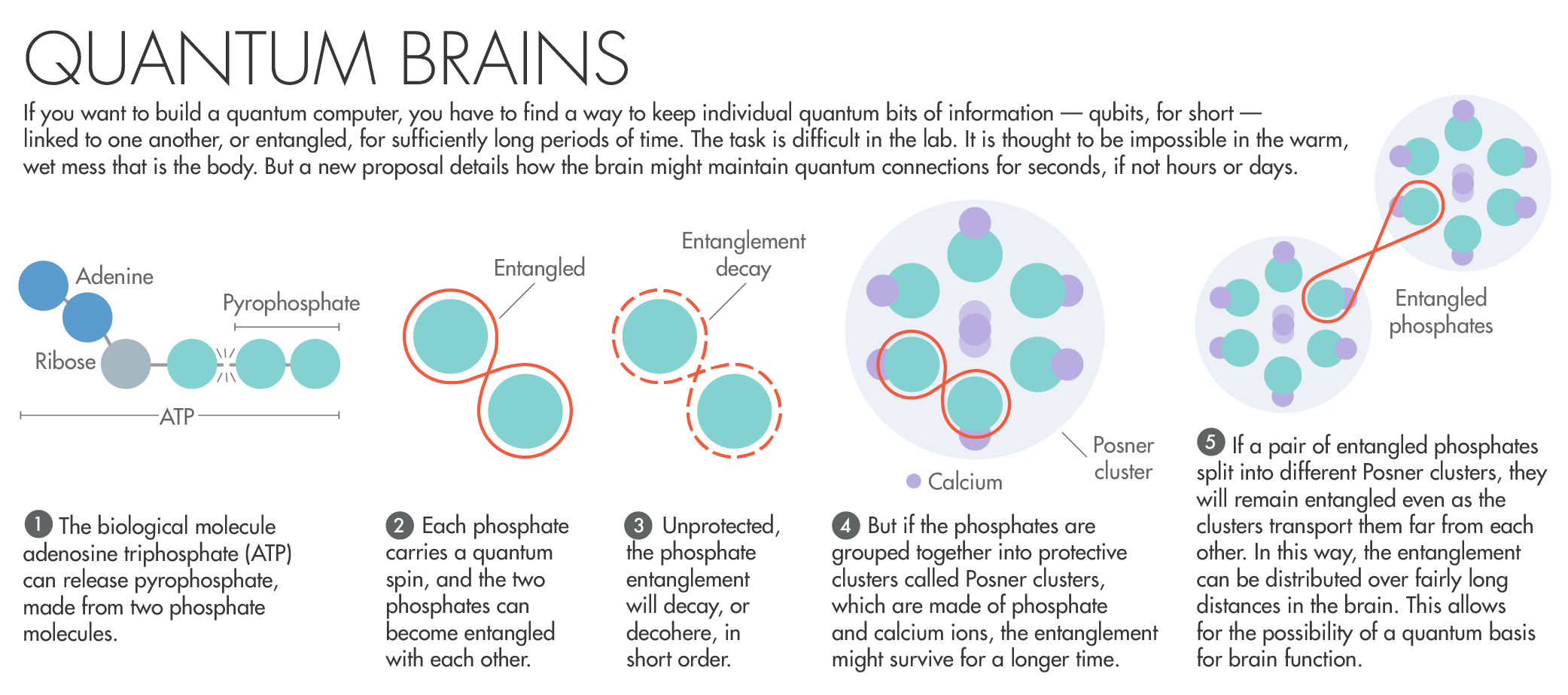
Lucy Reading-Ikkanda for Quanta Magazine
Quantum Protection Scheme
That said, going from an intriguing hypothesis to actually demonstrating that quantum processing plays a role in the brain is a daunting challenge. The brain would need some mechanism for storing quantum information in qubits for sufficiently long times. There must be a mechanism for entangling multiple qubits, and that entanglement must then have some chemically feasible means of influencing how neurons fire in some way. There must also be some means of transporting quantum information stored in the qubits throughout the brain.
This is a tall order. Over the course of his five-year quest, Fisher has identified just one credible candidate for storing quantum information in the brain: phosphorus atoms, which are the only common biological element other than hydrogen with a spin of one-half, a low number that makes possible longer coherence times. Phosphorus can’t make a stable qubit on its own, but its coherence time can be extended further, according to Fisher, if you bind phosphorus with calcium ions to form clusters.
In 1975, Aaron Posner, a Cornell University scientist, noticed an odd clustering of calcium and phosphorous atoms in his X-rays of bone. He made drawings of the structure of those clusters: nine calcium atoms and six phosphorous atoms, later called “Posner molecules” in his honor. The clusters popped up again in the 2000s, when scientists simulating bone growth in artificial fluid noticed them floating in the fluid. Subsequent experiments found evidence of the clusters in the body. Fisher thinks that Posner molecules could serve as a natural qubit in the brain as well.
That’s the big picture scenario, but the devil is in the details that Fisher has spent the past few years hammering out. The process starts in the cell with a chemical compound called pyrophosphate. It is made of two phosphates bonded together — each composed of a phosphorus atom surrounded by multiple oxygen atoms with zero spin. The interaction between the spins of the phosphates causes them to become entangled. They can pair up in four different ways: Three of the configurations add up to a total spin of one (a “triplet” state that is only weakly entangled), but the fourth possibility produces a zero spin, or “singlet” state of maximum entanglement, which is crucial for quantum computing.
Next, enzymes break apart the entangled phosphates into two free phosphate ions. Crucially, these remain entangled even as they move apart. This process happens much more quickly, Fisher argues, with the singlet state. These ions can then combine in turn with calcium ions and oxygen atoms to become Posner molecules. Neither the calcium nor the oxygen atoms have a nuclear spin, preserving the one-half total spin crucial for lengthening coherence times. So those clusters protect the entangled pairs from outside interference so that they can maintain coherence for much longer periods of time — Fisher roughly estimates it might last for hours, days or even weeks.
In this way, the entanglement can be distributed over fairly long distances in the brain, influencing the release of neurotransmitters and the firing of synapses between neurons — spooky action at work in the brain.
Testing the Theory
Researchers who work in quantum biology are cautiously intrigued by Fisher’s proposal. Alexandra Olaya-Castro, a physicist at University College London who has worked on quantum photosynthesis, calls it “a well-thought hypothesis. It doesn’t give answers, it opens questions that might then lead to how we could test particular steps in the hypothesis.”
University of Oxford chemist Peter Hore, who investigates whether migratory birds’ navigational systems make use of quantum effects, concurs. “Here’s a theoretical physicist who is proposing specific molecules, specific mechanics, all the way through to how this could affect brain activity,” he said. “That opens up the possibility of experimental testing.”
Experimental testing is precisely what Fisher is now trying to do. He just spent a sabbatical at Stanford University working with researchers there to replicate the 1986 study with pregnant rats. He acknowledged the preliminary results were disappointing, in that the data didn’t provide much information, but thinks if it’s repeated with a protocol closer to the original 1986 experiment, the results might be more conclusive.
Fisher has applied for funding to conduct further in-depth quantum chemistry experiments. He has cobbled together a small group of scientists from various disciplines at UCSB and the University of California, San Francisco, as collaborators. First and foremost, he would like to investigate whether calcium phosphate really does form stable Posner molecules, and whether the phosphorus nuclear spins of these molecules can be entangled for sufficiently long periods of time.
Even Hore and Olaya-Castro are skeptical of the latter, particularly Fisher’s rough estimate that the coherence could last a day or more. “I think it’s very unlikely, to be honest,” Olaya-Castro said. “The longest time scale relevant for the biochemical activity that’s happening here is the scale of seconds, and that’s too long.” (Neurons can store information for microseconds.) Hore calls the prospect “remote,” pegging the limit at one second at best. “That doesn’t invalidate the whole idea, but I think he would need a different molecule to get long coherence times,” he said. “I don’t think the Posner molecule is it. But I’m looking forward to hearing how it goes.”
Others see no need to invoke quantum processing to explain brain function. “The evidence is building up that we can explain everything interesting about the mind in terms of interactions of neurons,” said Paul Thagard, a neurophilosopher at the University of Waterloo in Ontario, Canada, to New Scientist. (Thagard declined our request to comment further.)
Plenty of other aspects of Fisher’s hypothesis also require deeper examination, and he hopes to be able to conduct the experiments to do so. Is the Posner molecule’s structure symmetrical? And how isolated are the nuclear spins?
Most important, what if all those experiments ultimately prove his hypothesis wrong? It might be time to give up on the notion of quantum cognition altogether. “I believe that if phosphorus nuclear spin is not being used for quantum processing, then quantum mechanics is not operative in longtime scales in cognition,” Fisher said. “Ruling that out is important scientifically. It would be good for science to know.”