Ancient Genes for Symbiosis Hint at Mitochondria’s Origins
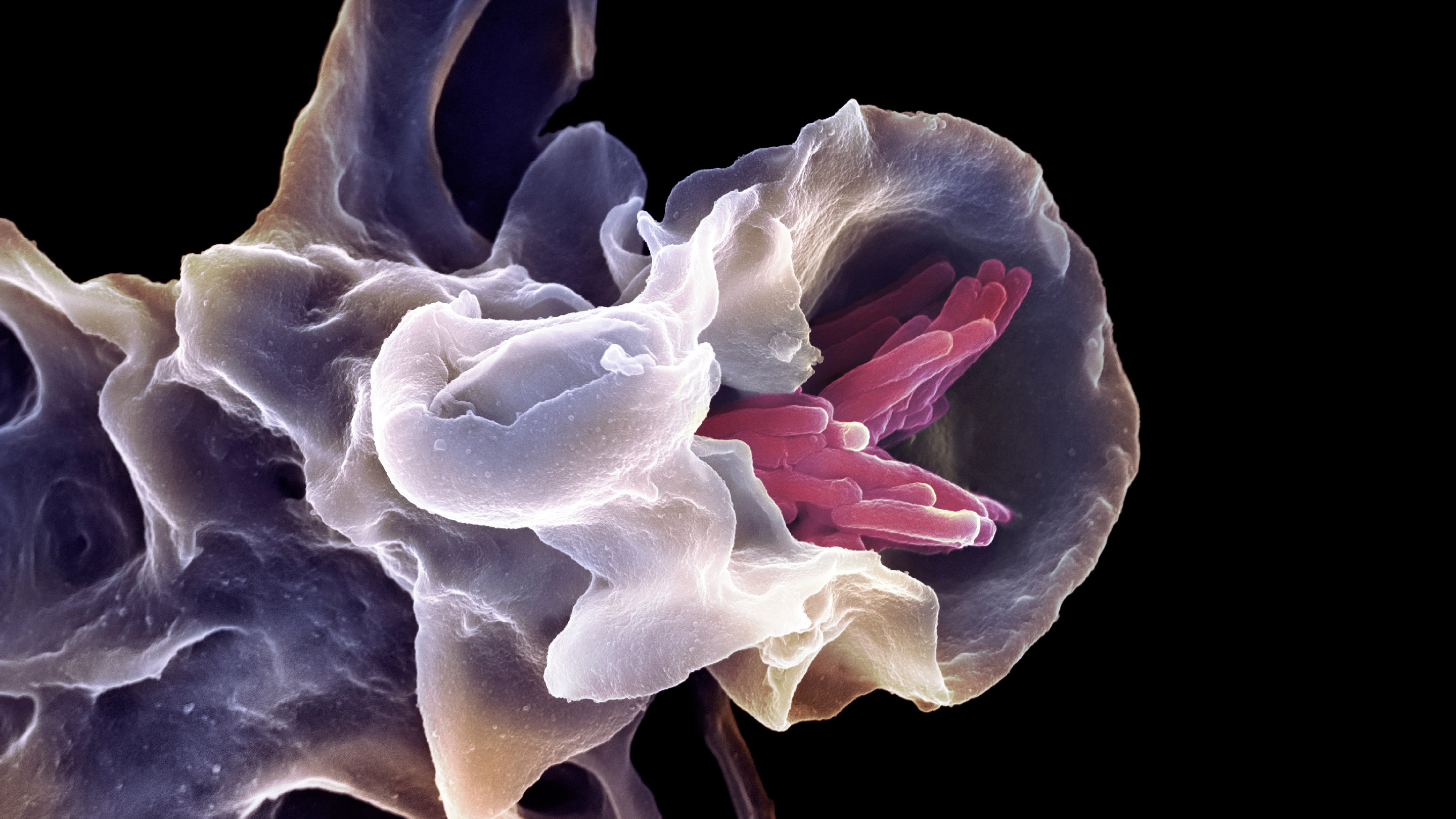
A white blood cell ingests rod-shaped bacteria (pink) in this micrograph. Some scientists are using the apparent age of this process, known as phagocytosis, to deduce when complex cells might have acquired mitochondria.
SPL/Science Source
Introduction
Once, long ago, the only players in the grand drama of life, predation and death were invisibly small and simple cells. Archaea and bacteria jigged and whirled through seas and ponds, assembled themselves into fortresses a few microns wide, and devoured films of organic matter. Then some of them began to change, and eventually the first eukaryote — the first organism to keep its genes locked away in a nucleus, to line its interior with ramifying compartments, and, crucially, to use mitochondria to make energy — appeared on the scene. We and all the rest of life visible to the naked eye are the descendants of that cell, the last common ancestor of all eukaryotes.
Scientists still understand relatively little about what happened during that transformation. One of the central conundrums is how and when our eukaryotic ancestor acquired its mitochondria, the powerhouse organelles that generate the cell’s energy. The mitochondrion was clearly once an independent bacterium, until some host cell (an archaeon or descendant of one, from all the evidence) engulfed it and turned it into a permanent symbiotic partner.
But the way eukaryotic cells engulf bacteria is energetically costly; it involves extensive and rapid remodeling of the cytoskeleton, the protein scaffolding beneath the cell membrane. A cell almost needs to possess mitochondria to do it, since mitochondria can wring about 18 times as much energy from a molecule of glucose as glycolysis and fermentation, the alternative metabolic processes. So scientists debate which came first: the mitochondrion or the engulfment process, known as phagocytosis.
The two options suggest vastly different origin stories for eukaryotes: Was the mitochondrion an afterthought, a late arrival in the evolution of the first eukaryote? Or did it come early, with its spectacular energy-generating powers, and drive the changes in our ancestor?
A recent paper in Molecular Biology and Evolution provides an intriguing peek into what might have happened when this chicken-or-egg dilemma played out more than 1.5 billion years ago. The researchers sequenced the DNA of more than 30 species of parasitic and symbiotic bacteria that, when engulfed by eukaryotic cells, avoid being digested and live off the resources of their hosts. The ability to dwell within eukaryotic cells, the scientists realized, seemed strikingly older than expected. It suggests — with some important caveats — that some version of phagocytosis predated mitochondria, setting the stage for the revolution to come.
Lionel Guy, an evolutionary microbiologist at Uppsala University in Sweden and an author of the new paper, began sequencing bacteria of the order Legionellales some years ago because it contains important pathogens, such as the one that causes Legionnaires’ disease, but genetic details for the group were scarce. “I knew there was more to them, and I wanted to get a comprehensive view of the order,” he said.
He was particularly interested in the genes that influence the interactions between the bacteria and their hosts. These genes code for proteins that target eukaryotic molecular systems to interfere with the host’s ability to sense the invader, among other benefits to the parasite.
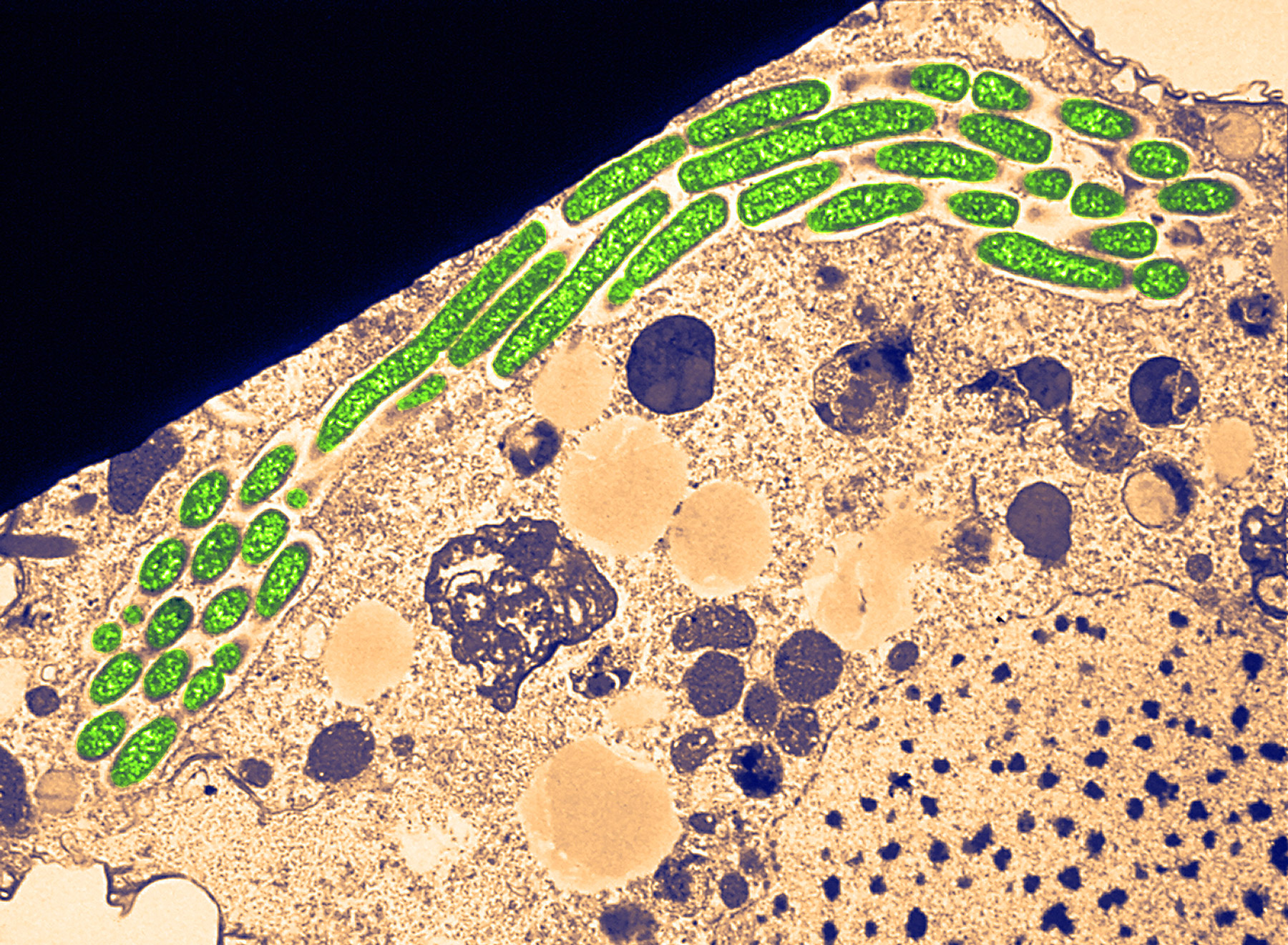
A chain of Legionella bacteria (green) move inside a protozoan they have infected. Bacteria in the Legionellales order exploit phagocytosis to get inside eukaryotic host cells.
Science Source
Guy and his colleagues sequenced the genes of members of Legionellales ranging from the Legionnaires’ disease pathogen to bacteria discovered by expeditions trawling the ocean for exotic microbes. Unsurprisingly, the proteins that the parasites used to shroud their presence and extract nutrients from their hosts varied substantially. But the researchers also found that nearly all the Legionellales deployed these proteins with fundamentally the same molecular machinery, called the Type IVB secretion system, which they seemingly inherited from a shared ancestor countless generations ago.
“Then, at a late stage, I got the million-dollar question” from a colleague, Guy recalls. “How old exactly are those Legionellales?” The answer, he realized, could illuminate the nature of the host cells.
Assigning a date to the origin of a bacterial group is a complicated process, and often an unsuccessful one. When biologists build a family tree for organisms with ancestors in the fossil record, they can calculate how long ago the rock formations holding those fossils arose. With bacteria, it’s different. Scientists can infer family relationships from the genes, but they usually can’t pinpoint how many millions of years ago one group branched off from another. Still, scientists have a handful of ways to determine the age of bacteria, primarily by checking for chemical markers in rocks for which the bacteria are the only known source.
Guy had read a paper from 2008 about one of these markers, a substance called okenone that certain purple sulfur bacteria make. Traces of it had been found in a rock formation in Australia dated to 1.64 billion years ago. Using a series of inferences based on the relatedness of the purple sulfur bacteria and Legionellales, Guy and his colleagues tentatively dated the origin of the Legionellales group to 1.9 billion years ago.
That’s a provocative number. If the group is that old and it inherited its machinery for hijacking eukaryotic cells from its earliest shared ancestor, this suggests that there were eukaryotic cells capable of phagocytosis to infect.
Merrill Sherman/Quanta Magazine
But many current estimates, based on fossils and chemical evidence, place the first appearance of cells with mitochondria nearly half a billion years later. The timing lends credence, the researchers suggest, to the theory that mitochondria were a late addition to evolving eukaryotes.
It is a clever take on a thorny problem, said Berend Snel, an evolutionary biologist at Utrecht University who has explored the early evolution of the eukaryotes using genetics. He and his colleagues have also suggested that when the mitochondrion arrived, it moved into a host cell that already had some rudiments of complexity. From the analysis of Legionellales, Snel thinks that Guy and his colleagues make “a convincing case” that this order of bacteria live in eukaryotes because their common ancestor had a way to live inside a phagocytic cell. “And that is, I think, a very smart and original complementary way of looking at the same problem,” he said.
However, it does not end the debate about mitochondrial origins, for at least two reasons.
Guy’s conclusion that the ancestors of Legionellales might have infected a proto-eukaryote before the arrival of mitochondria hinges on the dating of the okenone. Molecular clock calculations of this sort are notoriously fuzzy, with wide margins of error.
Such timings are therefore best treated as one part of a puzzle rather than as a statement of fact, said Toni Gabaldón, an evolutionary biologist and geneticist at the Institute for Research in Biomedicine in Barcelona and the Barcelona Supercomputing Center. “I always consider this with a grain of salt. It’s not bulletproof data, it’s an inference, a very difficult inference, because we are talking about a lot of time,” he said.
There are also mechanisms other than phagocytosis that might have brought the bacterial ancestor of mitochondria into the ancestor of eukaryotes. William Martin, a microbiologist at Heinrich Heine University Düsseldorf who has written extensively about the origins of mitochondria, is adamant that mitochondria were essential to the evolution of phagocytosis, not vice versa. In a landmark 1998 paper, Martin and his colleague Miklós Müller of Rockefeller University laid out how an archaeon that consumed hydrogen and a bacterium that produced it could have built a cozy symbiosis side by side. Over time, protrusions from the archaeon could have surrounded the bacterium and engulfed it.
If that’s a more plausible way for mitochondria to have entered a cell, then any evidence that the ancestor of Legionellales might have been infecting proto-eukaryotes isn’t relevant to the debate because phagocytosis is not a prerequisite for the arrival of the mitochondrion.
The frustrating challenge for any theory about the origin of mitochondria is that there are many details to account for, and all of them are important, said Snel. “It’s not solving the problem, because the problem is just too big,” he said of the new paper. The crucial events happened during an immensely long dark age, with no surviving intermediate forms left to help tell the story. The information we must draw on to understand this picture lies scattered through the genomes of the organisms that emerged from that obscurity.
“The history of life is written in genomes; we just have to learn how to read it,” said Martin.
To understand how this fundamental transition happened, scientists may have to look at the problem from new angles. For instance, says Snel, it’s commonly thought that the first big change in the evolution of eukaryotes, whatever it was, set the stage for everything else.
But the last addition to our ancestors’ toolkit may prove even more essential to understanding eukaryotes. “Whatever the final step was, it caused the extinction of all the other competitors. Maybe that was the most important,” said Snel.
“Scientists like a good mystery,” he said. “I just want to know what happened.”