Astronomers Find Black Holes Stirring Up the Biggest Galaxies
Introduction
When the Hitomi space telescope successfully reached orbit in early 2016, astronomers thought their string of bad luck was over. A predecessor, launched in 2000, had crashed into the ocean. A follow-up mission leaked helium; full operations lasted only a few weeks. Hitomi, an X-ray satellite with the unique ability to sort some of the universe’s most energetic photons by energy, represented astrophysicists’ latest chance to demystify the behavior of colossal galaxies. The moment it became operational, the spacecraft trained its eye on a cluster of galaxies in the constellation Perseus.
Scientists had long wondered why these energetically “active” galaxies — filled with gas measuring millions of degrees — don’t churn out stars at a more ferocious rate. As their hot clouds blast X-rays into space, the gas should cool, like a hot poker losing its glow. In time, the gas should settle and clump together, forming stars. “There should be huge galaxy building going on in the middle of these systems,” said Mark Voit, an astronomer at Michigan State University. But “we see nothing of the sort.” Neither do the galaxies heat up. Something appears to be keeping them Goldilocks warm.
Astrophysicists had long assumed that the supermassive black hole at the heart of each of the galaxies was micromanaging the thermostat. But how could such a tiny speck, comparable in size to our own solar system, drive the behavior of a galaxy hundreds of thousands of light-years across?
Researchers suspected that vast jets of energy, expelled from either side of the black hole, could stir up turbulence in the clouds to keep everything warm. Hitomi was launched in part to spot that turbulence.
Yet when Hitomi turned to Perseus, the gas clouds seemed too quiet, putting stress on the idea that turbulence was enough to do the job. Data collected in just the first few days of observations challenged a leading idea in astrophysics.
Then disaster struck.
Before researchers could turn Hitomi toward other galaxies for confirmation, the spacecraft tumbled out of control and spun itself into pieces, a victim of bad luck and ruinous engineering. A brief observation of Perseus was the main result to come out of the $300 million mission. “It’s a sad story,” said Voit. “We’ve been eager to have this capability to do good X-ray spectroscopy for decades.”
Now a new study has challenged Hitomi’s conclusions. By sidestepping the hard-to-get X-ray data, it finds that turbulence might still be responsible for the Goldilocks nature of these active galaxies.
Follow the Bubbles
If you want to study X-ray-spewing gas, a dedicated X-ray satellite is a nice thing to have. But researchers have come up with a way to probe for turbulence without the use of X-rays.
Yuan Li, a theoretical astrophysicist at the University of California, Berkeley, had originally planned to study special bubbles that lurk near the central supermassive black holes of massive galaxies. She wanted to check if the bubbles’ shapes matched her theories. Since the bubbles are cooler than the surrounding gas, they shine in visible light, which means you don’t need a fancy X-ray satellite to see these denser “filaments.” She searched the existing data and found observations of filaments at the center of three nearby galaxy clusters — Perseus, Abell 2597 and Virgo.
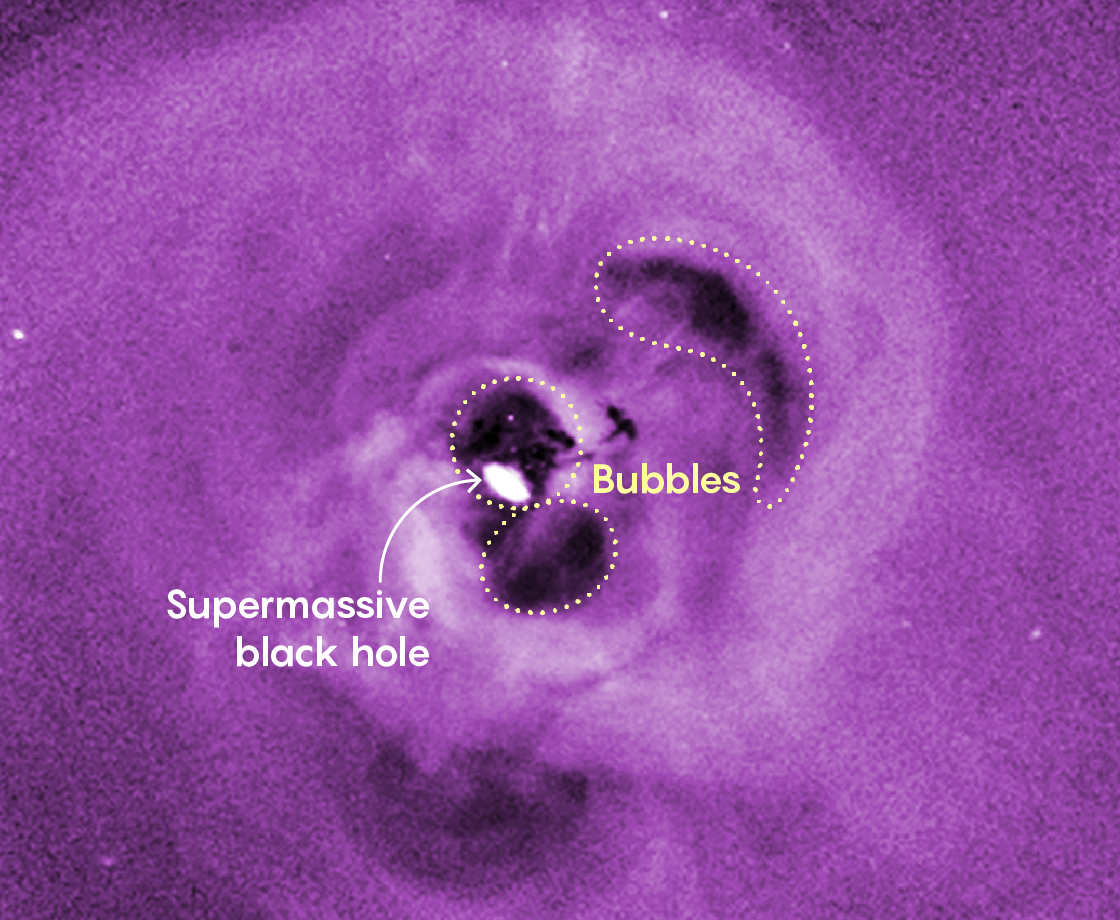
Bubbles surround the supermassive black hole at the center of the Perseus cluster.
When she got the data, she realized there was also information about which parts of the filaments were moving in which directions. She believed that if she tracked how the bubbles move, she could discern what was happening in the invisible gas — a strategy akin to using fluttering leaves to map the wind. “It’s a whole new way of looking at this issue,” Voit said.
The major question was turbulence, a specific pattern of movement where big bursts of energy break up into smaller whorls, which then split into smaller eddies, distributing energy all the way down to the scale of individual particles. Turbulence could explain how energy was getting from the black hole’s jets — enormous fountains of particles, thousands of light-years long — into particle-level jitters. Turbulence could be how the black hole kept the galaxy warm.
Li and her colleagues searched for the fingerprints of turbulence by coming up with a long list of pairs of locations in the filaments. Some of the pairs were close together, others farther apart. For each pair, they compared the relative speed of the gas at each point to the distance between the points.
If a filament was all moving in a uniform direction — falling toward the black hole, for instance — then points at all distances apart would appear to be moving at the same speed. But if points distant from one another moved relatively quickly, while nearby points moved slowly, then the filaments would be moving in complicated ways, with eddies of all sizes.
That’s exactly what the team found, as they describe in research to be published in The Astrophysical Journal Letters. The more distant two spots are, the faster they move.
Li marveled at how it was precisely the kind of intricate swirling we might expect from turbulent gas clouds pushing the filaments around. “It was almost a little disturbing how small the uncertainties were,” she said. “The data itself is truly beautiful.”
The existence of movements ranging from small to large represents, according to Li, a smoking gun indicating that turbulence is responsible for taking galactic-level energy and grinding it down to the particle level. “This [research] is telling us that black holes can drive turbulence,” she said.
Yet others would like to see more detailed modeling of the cascade process before they agree with the conclusions. “It’s interesting,” said Brian McNamara, an astrophysicist at the University of Waterloo in Canada, “but I think they need to go deeper.”
Li strengthens her case by tracing this energy flow back to the central black hole. The largest swirls of filament (and presumably the largest gas swirls) measure a few thousand light-years in size, matching the lengthy jets. If some other, larger cosmic spoon had stirred up the turbulence instead, the team would have found larger eddies.
As for the precise role of turbulence, debate continues. In textbook turbulence, energy cascades from large to small in a particular way — eddies of a given size generate whorls of a certain type. In Li’s filaments, however, the smaller eddies don’t turn quite quickly enough to satisfy the classic definition. Energy fails to reach the small parts of the filaments as efficiently as it should if turbulence alone is doing the distributing.
One turbulence-saving explanation lies in the poorly understood nature of the gas clouds. The clouds are made of a plasma of charged particles so thin that any one particle might drift for dozens of light-years without encountering another. In such wispy veils, energy may well disappear into heat earlier than theory predicts.
Or the appearance of turbulence could be an illusion. Just because the galaxies have large-scale and small-scale motion, said Christopher Reynolds, an astrophysicist at the University of Cambridge, that doesn’t guarantee that turbulence is turning the first into the second. The slower, finer motion could represent thumping sound waves from the black hole — shock waves produced when the jets slam into the surrounding gas.
Li, for her part, believes that true turbulence generates at least some heat. “The real picture is more complicated than what we had previously thought,” she said. While classical turbulence comes from incessant stirring, she pointed out, black hole jets switch on and off, which could also explain the unconventional energy cascade.
Regardless of how much heat comes down to turbulence, researchers hope to further study filaments for their role in tying the whole galactic cycle together. Jets feed the hot gas, which condenses into the cooler clumps, which may eventually fall into the black hole to power future jets. How each step of this cycle really works remains anyone’s guess, but the filaments are likely one of the keys to how, in galaxy after galaxy, the central black hole puts out just enough energy to avoid cooling, but not so much as to trigger runaway heating.
Nailing down the details of that cycle will take both computer simulations and wider observations of filaments. In addition, X-ray images of the invisible gas itself should directly reveal what’s going on. For that, astrophysicists will have to wait for Hitomi’s replacement, currently scheduled for launch in 2021. Fingers crossed.
Editor’s note: Li has received funding from the Simons Foundation, which also funds this editorially independent magazine.
This article was reprinted in Spanish at Investigacionyciencia.es.