Beating the Odds for Lucky Mutations
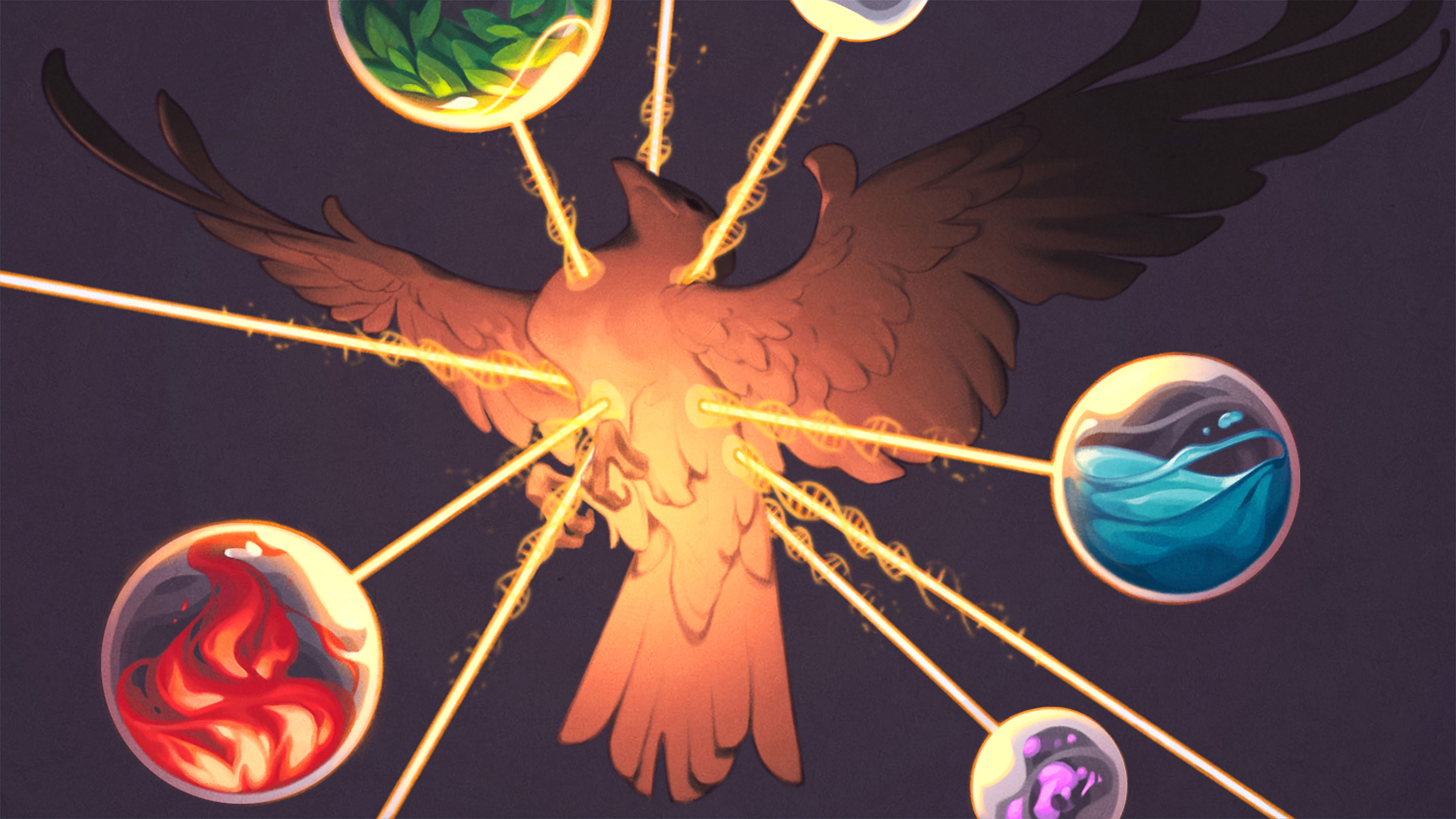
Ashley Mackenzie for Quanta Magazine
Introduction
In 1944, a Columbia University doctoral student in genetics named Evelyn Witkin made a fortuitous mistake. During her first experiment in a laboratory at Cold Spring Harbor, in New York, she accidentally irradiated millions of E. coli with a lethal dose of ultraviolet light. When she returned the following day to check on the samples, they were all dead — except for one, in which four bacterial cells had survived and continued to grow. Somehow, those cells were resistant to UV radiation. To Witkin, it seemed like a remarkably lucky coincidence that any cells in the culture had emerged with precisely the mutation they needed to survive — so much so that she questioned whether it was a coincidence at all.
For the next two decades, Witkin sought to understand how and why these mutants had emerged. Her research led her to what is now known as the SOS response, a DNA repair mechanism that bacteria employ when their genomes are damaged, during which dozens of genes become active and the rate of mutation goes up. Those extra mutations are more often detrimental than beneficial, but they enable adaptations, such as the development of resistance to UV or antibiotics.
The question that has tormented some evolutionary biologists ever since is whether nature favored this arrangement. Is the upsurge in mutations merely a secondary consequence of a repair process inherently prone to error? Or, as some researchers claim, is the increase in the mutation rate itself an evolved adaptation, one that helps bacteria evolve advantageous traits more quickly in stressful environments?
The scientific challenge has not just been to demonstrate convincingly that harsh environments cause nonrandom mutations. It has also been to find a plausible mechanism consistent with the rest of molecular biology that could make lucky mutations more likely. Waves of studies in bacteria and more complex organisms have sought those answers for decades.
The latest and perhaps best answer — for explaining some kinds of mutations, anyway — has emerged from studies of yeast, as reported in June in PLOS Biology. A team led by Jonathan Houseley, a specialist in molecular biology and genetics at the Babraham Institute in Cambridge, proposed a mechanism that drives more mutation specifically in regions of the yeast genome where it could be most adaptive.
“It’s a totally new way that the environment can have an impact on the genome to allow adaptation in response to need. It is one of the most directed processes we’ve seen yet,” said Philip Hastings, professor of molecular and human genetics at Baylor College of Medicine, who was not involved in the Houseley group’s experiments. Other scientists contacted for this story also praised the work, though most cautioned that much about the controversial idea was still speculative and needed more support.
Increasing Variety in the Genome
“Rather than asking very broad questions like ‘are mutations always random?’ I wanted to take a more mechanistic approach,” Houseley said. He and his colleagues directed their attention to a specific kind of mutation called copy number variation. DNA often contains multiple copies of extended sequences of base pairs or even whole genes. The exact number can vary among individuals because, when cells are duplicating their DNA before cell division, certain mistakes can insert or delete copies of gene sequences. In humans, for instance, 5 to 10 percent of the genome shows copy number variation from person to person — and some of these variations have been linked to cancer, diabetes, autism and a host of genetic disorders. Houseley suspected that in at least some cases, this variation in the number of gene copies might be a response to stresses or hazards in the environment.
In 2015, Houseley and his colleagues described a mechanism by which yeast cells seemed to be driving extra copy number variation in genes associated with ribosomes, the parts of a cell that synthesize proteins. However, they did not prove that this increase was a purposefully adaptive response to a change or constraint in the cellular environment. Nevertheless, to them it seemed that the yeast was making more copies of the ribosomal genes when nutrients were abundant and the demand for making protein might be higher.
Houseley therefore decided to test whether similar mechanisms might act on genes more directly activated by hazardous changes in the environment. In their 2017 paper, he and his team focused on CUP1, a gene that helps yeast resist the toxic effects of environmental copper. They found that when yeast was exposed to copper, the variation in the number of copies of CUP1 in the cells increased. On average, most cells had fewer copies of the gene, but the yeast cells that gained more copies — about 10 percent of the total population — became more resistant to copper and flourished. “The small number of cells that did the right thing,” Houseley said, “were at such an advantage that they were able to outcompete everything else.”
But that change did not in itself mean much: If the environmental copper was causing mutations, then the change in CUP1 copy number variation might have been no more than a meaningless consequence of the higher mutation rate. To rule out that possibility, the researchers cleverly re-engineered the CUP1 gene so that it would respond to a harmless, nonmutagenic sugar, galactose, instead of copper. When these altered yeast cells were exposed to galactose, the variation in their number of copies of the gene changed, too.
The cells seemed to be directing greater variation to the exact place in their genome where it would be useful. After more work, the researchers identified elements of the biological mechanism behind this phenomenon. It was already known that when cells replicate their DNA, the replication mechanism sometimes stalls. Usually the mechanism can restart and pick up where it left off. When it can’t, the cell can go back to the beginning of the replication process, but in doing so, it sometimes accidentally deletes a gene sequence or makes extra copies of it. That is what causes normal copy number variation. But Houseley and his team made the case that a combination of factors makes these copying errors especially likely to hit genes that are actively responding to environmental stresses, which means that they are more likely to show copy number variation.
The key point is that these effects center on genes responding to the environment, and that they could give natural selection extra opportunities to fine-tune which levels of gene expression might be optimal against certain challenges. The results seem to present experimental evidence that a challenging environment could galvanize cells into controlling those genetic changes that would best improve their fitness. They may also seem reminiscent of the outmoded, pre-Darwinian ideas of the French naturalist Jean-Baptiste Lamarck, who believed that organisms evolved by passing their environmentally acquired characteristics along to their offspring. Houseley maintains, however, that this similarity is only superficial.
“What we have defined is a mechanism that has arisen entirely through Darwinian selection of random mutations to give a process that stimulates nonrandom mutations at useful sites,” Houseley said. “It is not Lamarckian adaptation. It just achieves some of the same ends without the problems involved with Lamarckian adaptation.”
The Adaptive Mutation Debate
Ever since 1943, when the microbiologist Salvador Luria and the biophysicist Max Delbrück showed with Nobel prize-winning experiments that mutations in E. coli occur randomly, observations like the bacterial SOS response have made some biologists wonder whether there might be important loopholes to that rule. For example, in a controversial paper published in Nature in 1988, John Cairns of Harvard and his team found that when they placed bacteria that could not digest the milk sugar lactose in an environment where that sugar was the sole food source, the cells soon evolved the ability to convert the lactose into energy. Cairns argued that this result showed that cells had mechanisms to make certain mutations preferentially when they would be beneficial.
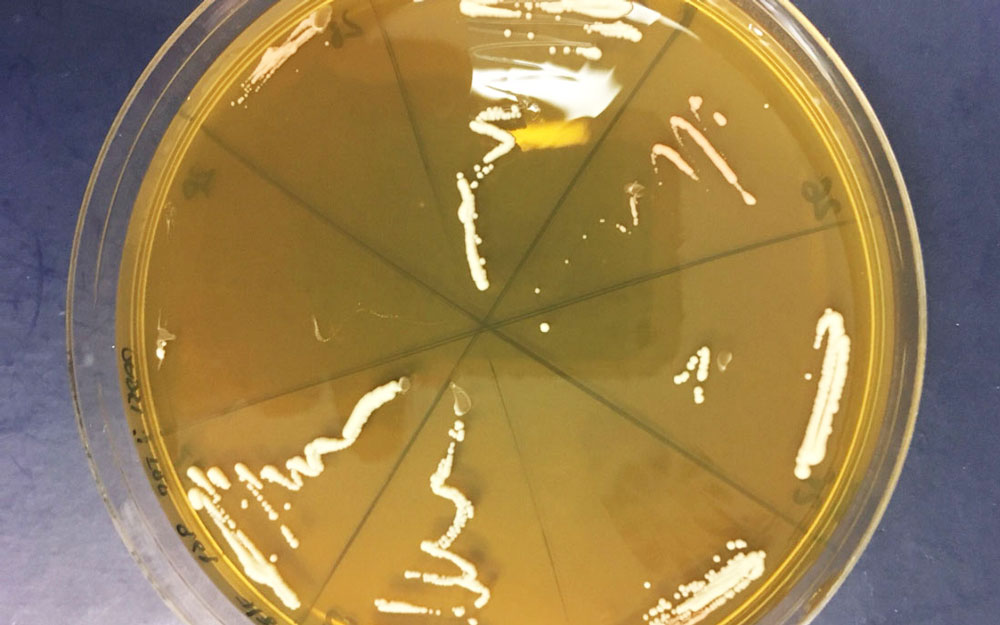
Budding yeast (S. cerevisiae) grow as colonies on this agar plate. If certain recent research is correct, a mechanism that helps to repair DNA damage in these cells may also promote more adaptive mutations, which could help the cells to evolve more quickly under harsh circumstances.
Jon Houseley
Experimental support for that specific idea eventually proved lacking, but some biologists were inspired to become proponents of a broader theory that has come to be known as adaptive mutation. They believe that even if cells can’t direct the precise mutation needed in a certain environment, they can adapt by elevating their mutation rate to promote genetic change.
The work of the Houseley team seems to bolster the case for that position. In the yeast mechanism “there’s not selection for a mechanism that actually says, ‘This is the gene I should mutate to solve the problem,’” said Patricia Foster, a biologist at Indiana University. “It shows that evolution can get speeded up.”
Hastings at Baylor agreed, and praised the fact that Houseley’s mechanism explains why the extra mutations don’t happen throughout the genome. “You need to be transcribing a gene for it to happen,” he said.
Adaptive mutation theory, however, finds little acceptance among most biologists, and many of them view the original experiments by Cairns and the new ones by Houseley skeptically. They argue that even if higher mutation rates yield adaptations to environmental stress, proving that the higher mutation rates are themselves an adaptation to stress remains difficult to demonstrate convincingly. “The interpretation is intuitively attractive,” said John Roth, a geneticist and microbiologist at the University of California, Davis, “but I don’t think it’s right. I don’t believe any of these examples of stress-induced mutagenesis are correct. There may be some other non-obvious explanation for the phenomenon.”
“I think [Houseley’s work] is beautiful and relevant to the adaptive mutation debate,” said Paul Sniegowski, a biologist at the University of Pennsylvania. “But in the end, it still represents a hypothesis.” To validate it more certainly, he added, “they’d have to test it in the way an evolutionary biologist would” — by creating a theoretical model and determining whether this adaptive mutability could evolve within a reasonable period, and then by challenging populations of organisms in the lab to evolve a mechanism like this.
Notwithstanding the doubters, Houseley and his team are persevering with their research to understand its relevance to cancer and other biomedical problems. “The emergence of chemotherapy-resistant cancers is commonplace and forms a major barrier to curing the disease,” Houseley said. He thinks that chemotherapy drugs and other stresses on tumors may encourage malignant cells to mutate further, including mutations for resistance to the drugs. If that resistance is facilitated by the kind of mechanism he explored in his work on yeast, it could very well present a new drug target. Cancer patients might be treated both with normal courses of chemotherapy and with agents that would inhibit the biochemical modifications that make resistance mutations possible.
“We are actively working on that,” Houseley said, “but it’s still in the early days.”
This article was reprinted on Wired.com.