To Learn More Quickly, Brain Cells Break Their DNA
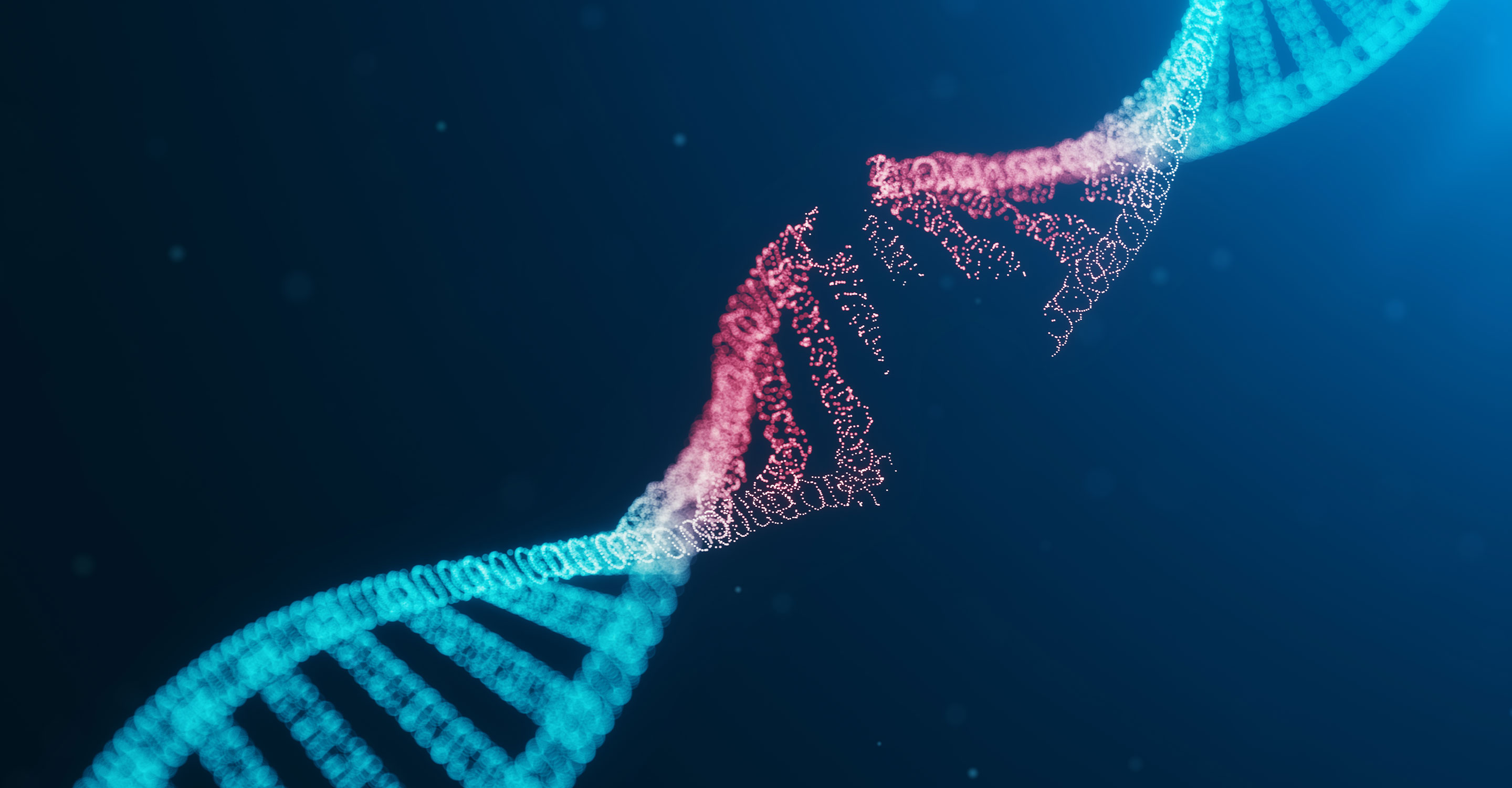
Double-strand breaks in DNA, usually viewed as a dangerous form of genetic damage, can also play a crucial role in normal cellular processes.
Rost9/Shutterstock
Introduction
Faced with a threat, the brain has to act fast, its neurons making new connections to learn what might spell the difference between life and death. But in its response, the brain also raises the stakes: As an unsettling recent discovery shows, to express learning and memory genes more quickly, brain cells snap their DNA into pieces at many key points, and then rebuild their fractured genome later.
The finding doesn’t just provide insights into the nature of the brain’s plasticity. It also demonstrates that DNA breakage may be a routine and important part of normal cellular processes — which has implications for how scientists think about aging and disease, and how they approach genomic events they’ve typically written off as merely bad luck.
The discovery is all the more surprising because DNA double-strand breaks, in which both rails of the helical ladder get cut at the same position along the genome, are a particularly dangerous kind of genetic damage associated with cancer, neurodegeneration and aging. It’s more difficult for cells to repair double-strand breaks than other kinds of DNA damage because there isn’t an intact “template” left to guide the reattachment of the strands.
Yet it’s also long been recognized that DNA breakage sometimes plays a constructive role, too. When cells are dividing, double-strand breaks allow for the normal process of genetic recombination between chromosomes. In the developing immune system, they enable pieces of DNA to recombine and generate a diverse repertoire of antibodies. Double-strand breaks have also been implicated in neuronal development and in helping turn certain genes on. Still, those functions have seemed like exceptions to the rule that double-strand breaks are accidental and unwelcome.
But a turning point came in 2015. Li-Huei Tsai, a neuroscientist and director of the Picower Institute for Learning and Memory at the Massachusetts Institute of Technology, and her colleagues were following up on previous work that had linked Alzheimer’s disease with the accumulation of double-strand breaks in neurons. To their surprise, the researchers found that stimulating cultured neurons triggered double-strand breaks in their DNA, and the breaks quickly increased the expression of a dozen fast-acting genes associated with synaptic activity in learning and memory.
The double-strand breaks seemed to be essential for regulating gene activity important to the neurons’ function. Tsai and her collaborators hypothesized that the breaks essentially released enzymes that were stuck along twisted pieces of DNA, freeing them to transcribe relevant nearby genes quickly. But the idea “was met with a lot of skepticism,” Tsai said. “People simply have a hard time imagining that double-strand breaks can actually be physiologically important.”
Nevertheless, Paul Marshall, a postdoctoral researcher at the University of Queensland in Australia, and his colleagues decided to follow up on the finding. Their work, which appeared in 2019, both confirmed and extended the observations by Tsai’s team. It showed that the DNA breakage touched off two waves of enhanced gene transcription, one immediate and one several hours later.
Marshall and his colleagues proposed a two-step mechanism to explain the phenomenon: When the DNA breaks, some enzyme molecules are freed for transcription (as Tsai’s group suggested) and the site of the break is also chemically flagged with a methyl group, a so-called epigenetic marker. Later, when repair of the broken DNA begins, the marker is removed — and in the process, still more enzymes can spill free, starting the second round of transcription.
“Not only is the double-strand break involved as a trigger,” Marshall said, “it then becomes a marker, and that marker itself is functional in terms of regulating and guiding machinery to that location.”
Since then, other studies have demonstrated something similar. One, published last year, associated double-strand breaks not just with the formation of a fear memory, but with its recollection.
Now, in a study last month in PLOS ONE, Tsai and her colleagues have shown that this counterintuitive mechanism of gene expression might be prevalent in the brain. This time, instead of using cultured neurons, they looked at cells in the brains of living mice that were learning to associate an environment with an electric shock. When the team mapped genes undergoing double-strand breaks in the prefrontal cortex and hippocampus of mice that had been shocked, they found breaks occurring near hundreds of genes, many of which were involved in synaptic processes related to memory.
Equally interesting, however, was that some double-strand breaks were also occurring in the neurons of mice that had not been shocked. “These breaks are occurring just normally in the brain,” said Timothy Jarome, a neuroscientist at Virginia Polytechnic Institute and State University who did not participate in the study but has done related work. “I think that’s the most surprising aspect from this, because it suggests that it’s occurring all the time.”
In further support of that conclusion, the scientists also observed double-strand breaks in non-neuronal brain cells called glia, in which they regulate a different assortment of genes. The finding implies a role for glia in the formation and storage of memories, and it hints that DNA breakage might be a regulatory mechanism in many other cell types. “It’s probably a broader mechanism than we think it is,” Jarome said.
But even if breaking DNA is a particularly fast way to induce crucial gene expression, whether for memory consolidation or for other cellular functions, it’s also risky. If the double-strand breaks occur at the same locations over and over again and aren’t properly repaired, genetic information could be lost. Moreover, “this type of gene regulation could render neurons vulnerable to genomic lesions, especially during aging and under neurotoxic conditions,” Tsai said.
“It is interesting that it’s used so intensively in the brain,” said Bruce Yankner, a neurologist and geneticist at Harvard Medical School who was not involved in the new work, “and that the cells can get away with it without incurring damage that’s devastating.”
That’s probably because the repair process is efficient and effective — but with age, that could change. Tsai, Marshall and others are studying whether and how this could become a mechanism of neurodegeneration in conditions such as Alzheimer’s disease. Yankner says that it could also potentially contribute to glial cancers or post-traumatic stress disorder. And if double-strand breaks regulate gene activity in cells outside the nervous system, the breakdown of that mechanism could also lead to, say, muscle loss or heart disease.
As the details and uses of this mechanism in the body become better understood, they could eventually guide the development of new medical treatments. At the very least, Marshall said, simply trying to prevent double-strand breaks might not be the right approach, given their importance in basic memory processes.
But the work also demonstrates a broader need to stop thinking about the genome in static terms, and to start envisioning it as something dynamic. “Whenever you’re utilizing that [DNA] template, you disturb the template, you change the template,” Marshall said. “And that’s not necessarily a bad thing.”
He and his colleagues have started to examine other types of DNA changes associated with dysregulation and negative consequences, including cancer. They have uncovered some crucial roles for these changes as well in regulating basic memory-related processes.
Marshall believes that many researchers still have trouble seeing the breaking of DNA as a fundamental regulatory mechanism of gene transcription. “It hasn’t really caught on yet,” he said. “People are still very switched on to the idea of it being DNA damage.” But he hopes his work and the new results from Tsai’s team “will open up the door for other people … to probe a bit deeper.”