Brainless Embryos Suggest Bioelectricity Guides Growth
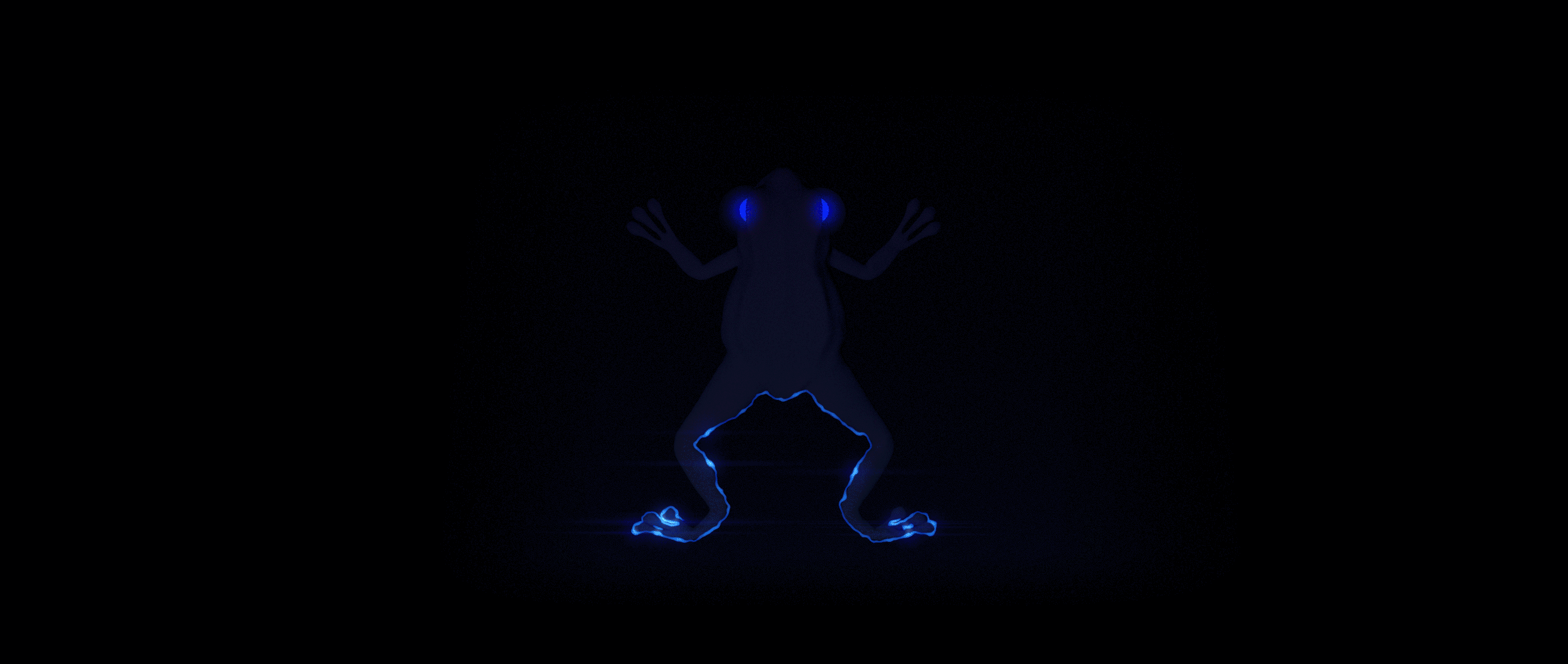
A variety of experiments suggest that embryonic tissues depend on bioelectric cues, as well as genetic information, to develop correctly.
Olena Shmahalo/Quanta Magazine
Introduction
The tiny tadpole embryo looked like a bean. One day old, it didn’t even have a heart yet. The researcher in a white coat and gloves who hovered over it made a precise surgical incision where its head would form. Moments later, the brain was gone, but the embryo was still alive.
The brief procedure took Celia Herrera-Rincon, a neuroscience postdoc at the Allen Discovery Center at Tufts University, back to the country house in Spain where she had grown up, in the mountains near Madrid. When she was 11 years old, while walking her dogs in the woods, she found a snake, Vipera latastei. It was beautiful but dead. “I realized I wanted to see what was inside the head,” she recalled. She performed her first “lab test” using kitchen knives and tweezers, and she has been fascinated by the many shapes and evolutionary morphologies of the brain ever since. Her collection now holds about 1,000 brains from all kinds of creatures.
This time, however, she was not interested in the brain itself, but in how an African clawed frog would develop without one. She and her supervisor, Michael Levin, a software engineer turned developmental biologist, are investigating whether the brain and nervous system play a crucial role in laying out the patterns that dictate the shapes and identities of emerging organs, limbs and other structures.
For the past 65 years, the focus of developmental biology has been on DNA as the carrier of biological information. Researchers have typically assumed that genetic expression patterns alone are enough to determine embryonic development.
To Levin, however, that explanation is unsatisfying. “Where does shape come from? What makes an elephant different from a snake?” he asked. DNA can make proteins inside cells, he said, but “there is nothing in the genome that directly specifies anatomy.” To develop properly, he maintains, tissues need spatial cues that must come from other sources in the embryo. At least some of that guidance, he and his team believe, is electrical.
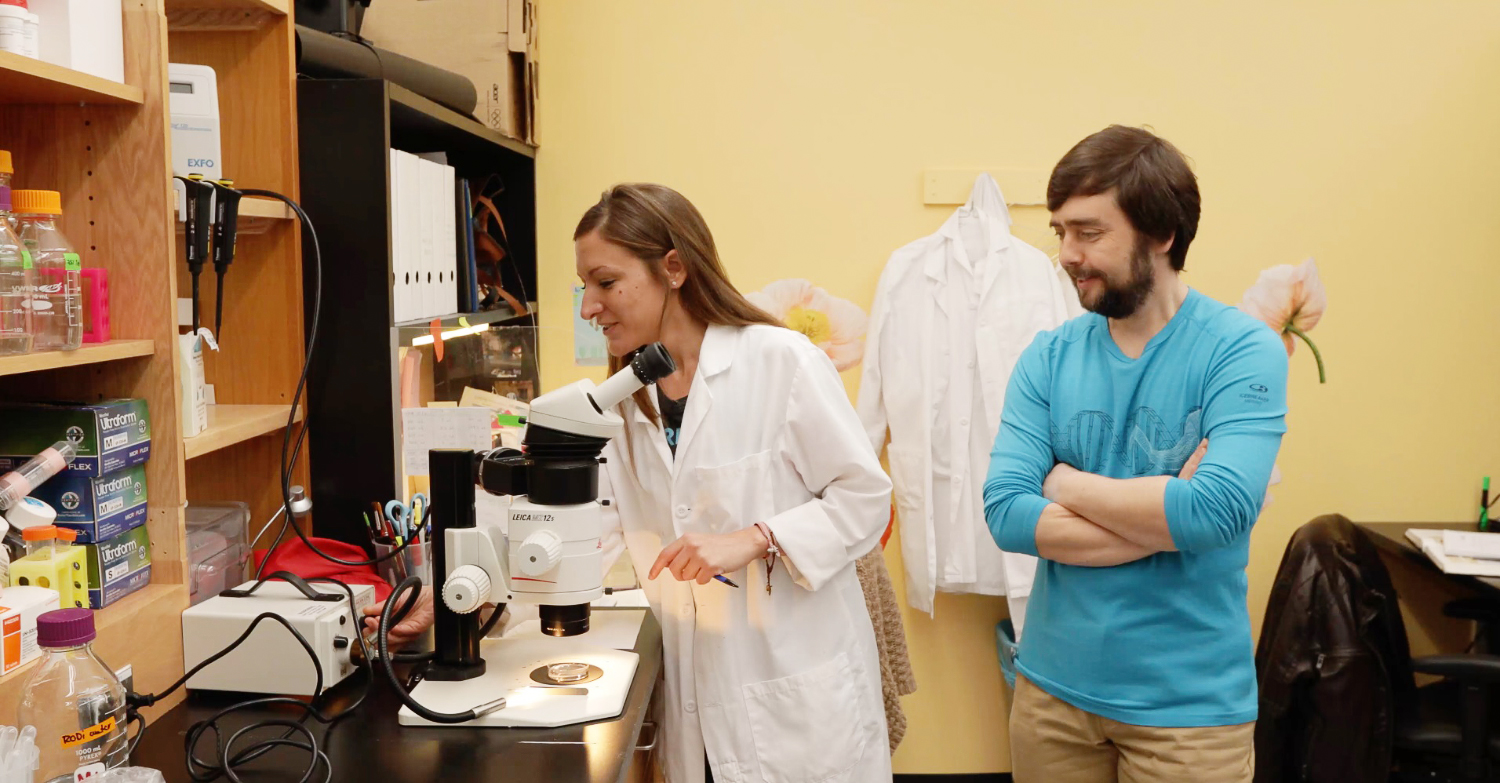
The developmental biologists Celia Herrera-Rincon and Michael Levin at the Allen Discovery Center at Tufts University found evidence that signals from the developing brain affect the form of distant tissue structures in tadpoles.
El Futuro es One/EL PAIS
In recent years, by working on tadpoles and other simple creatures, Levin’s laboratory has amassed evidence that the embryo is molded by bioelectrical signals, particularly ones that emanate from the young brain long before it is even a functional organ. Those results, if replicated in other organisms, may change our understanding of the roles of electrical phenomena and the nervous system in development, and perhaps more widely in biology.
“Levin’s findings will shake some rigid orthodoxy in the field,” said Sui Huang, a molecular biologist at the Institute for Systems Biology. If Levin’s work holds up, Huang continued, “I think many developmental biologists will be stunned to see that the construction of the body plan is not due to local regulation of cells … but is centrally orchestrated by the brain.”
Bioelectrical Influences in Development
The Spanish neuroscientist and Nobel laureate Santiago Ramón y Cajal once called the brain and neurons, the electrically active cells that process and transmit nerve signals, the “butterflies of the soul.” The brain is a center for information processing, memory, decision making and behavior, and electricity figures into its performance of all of those activities.
But it’s not just the brain that uses bioelectric signaling — the whole body does. All cell membranes have embedded ion channels, protein pores that act as pathways for charged molecules, or ions. Differences between the number of ions inside and outside a cell result in an electric gradient — the cell’s resting potential. Vary this potential by opening or blocking the ion channels, and you change the signals transmitted to, from and among the cells all around. Neurons do this as well, but even faster: To communicate among themselves, they use molecules called neurotransmitters that are released at synapses in response to voltage spikes, and they send ultra-rapid electrical pulses over long distances along their axons, encoding information in the pulses’ pattern, to control muscle activity.
Levin has thought about hacking networks of neurons since the mid-1980s, when he was a high school student in the suburbs near Boston, writing software for pocket money. One day, while browsing a small bookstore in Vancouver at Expo 86 with his father, he spotted a volume called The Body Electric, by Robert O. Becker and Gary Selden. He learned that scientists had been investigating bioelectricity for centuries, ever since Luigi Galvani discovered in the 1780s that nerves are animated by what he called “animal electricity.”
However, as Levin continued to read up on the subject, he realized that, even though the brain uses electricity for information processing, no one seemed to be seriously investigating the role of bioelectricity in carrying information about a body’s development. Wouldn’t it be cool, he thought, if we could comprehend “how the tissues process information and what tissues were ‘thinking about’ before they evolved nervous systems and brains?”
He started digging deeper and ended up getting a biology doctorate at Harvard University in morphogenesis — the study of the development of shapes in living things. He worked in the tradition of scientists like Emil du Bois-Reymond, a 19th-century German physician who discovered the action potential of nerves. In the 1930s and ’40s, the American biologists Harold Burr and Elmer Lund measured electric properties of various organisms during their embryonic development and studied connections between bioelectricity and the shapes animals take. They were not able to prove a link, but they were moving in the right direction, Levin said.
Before Genes Reigned Supreme
The work of Burr and Lund occurred during a time of widespread interest in embryology. Even the English mathematician Alan Turing, famed for cracking the Enigma code, was fascinated by embryology. In 1952 he published a paper suggesting that body patterns like pigmented spots and zebra stripes arise from the chemical reactions of diffusing substances, which he called morphogens.
But organic explanations like morphogens and bioelectricity didn’t stay in the limelight for long. In 1953, James Watson and Francis Crick published the double helical structure of DNA, and in the decades since “the focus of developmental biology has been on DNA as the carrier of biological information, with cells thought to follow their own internal genetic programs, prompted by cues from their local environment and neighboring cells,” Huang said.
The rationale, according to Richard Nuccitelli, chief science officer at Pulse Biosciences and a former professor of molecular biology at the University of California, Davis, was that “since DNA is what is inherited, information stored in the genes must specify all that is needed to develop.” Tissues are told how to develop at the local level by neighboring tissues, it was thought, and each region patterns itself from information in the genomes of its cells.
The extreme form of this view is “to explain everything by saying ‘it is in the genes,’ or DNA, and this trend has been reinforced by the increasingly powerful and affordable DNA sequencing technologies,” Huang said. “But we need to zoom out: Before molecular biology imposed our myopic tunnel vision, biologists were much more open to organism-level principles.”
The tide now seems to be turning, according to Herrera-Rincon and others. “It’s too simplistic to consider the genome as the only source of biological information,” she said. Researchers continue to study morphogens as a source of developmental information in the nervous system, for example. Last November, Levin and Chris Fields, an independent scientist who works in the area where biology, physics and computing overlap, published a paper arguing that cells’ cytoplasm, cytoskeleton and both internal and external membranes also encode important patterning data — and serve as systems of inheritance alongside DNA.
And, crucially, bioelectricity has made a comeback as well. In the 1980s and ’90s, Nuccitelli, along with the late Lionel Jaffe at the Marine Biological Laboratory, Colin McCaig at the University of Aberdeen, and others, used applied electric fields to show that many cells are sensitive to bioelectric signals and that electricity can induce limb regeneration in nonregenerative species.
According to Masayuki Yamashita of the International University of Health and Welfare in Japan, many researchers forget that every living cell, not just neurons, generates electric potentials across the cell membrane. “This electrical signal works as an environmental cue for intercellular communication, orchestrating cell behaviors during morphogenesis and regeneration,” he said.
However, no one was really sure why or how this bioelectric signaling worked, said Levin, and most still believe that the flow of information is very local. “Applied electricity in earlier experiments directly interacts with something in cells, triggering their responses,” he said. But what it was interacting with and how the responses were triggered were mysteries.
That’s what led Levin and his colleagues to start tinkering with the resting potential of cells. By changing the voltage of cells in flatworms, over the last few years they produced worms with two heads, or with tails in unexpected places. In tadpoles, they reprogrammed the identity of large groups of cells at the level of entire organs, making frogs with extra legs and changing gut tissue into eyes — simply by hacking the local bioelectric activity that provides patterning information.
And because the brain and nervous system are so conspicuously active electrically, the researchers also began to probe their involvement in long-distance patterns of bioelectric information affecting development. In 2015, Levin, his postdoc Vaibhav Pai, and other collaborators showed experimentally that bioelectric signals from the body shape the development and patterning of the brain in its earliest stages. By changing the resting potential in the cells of tadpoles as far from the head as the gut, they appeared to disrupt the body’s “blueprint” for brain development. The resulting tadpoles’ brains were smaller or even nonexistent, and brain tissue grew where it shouldn’t.
Unlike previous experiments with applied electricity that simply provided directional cues to cells, “in our work, we know what we have modified — resting potential — and we know how it triggers responses: by changing how small signaling molecules enter and leave cells,” Levin said. The right electrical potential lets neurotransmitters go in and out of voltage-powered gates (transporters) in the membrane. Once in, they can trigger specific receptors and initiate further cellular activity, allowing researchers to reprogram identity at the level of entire organs.
Lucy Reading-Ikkanda/Quanta Magazine
This work also showed that bioelectricity works over long distances, mediated by the neurotransmitter serotonin, Levin said. (Later experiments implicated the neurotransmitter butyrate as well.) The researchers started by altering the voltage of cells near the brain, but then they went farther and farther out, “because our data from the prior papers showed that tumors could be controlled by electric properties of cells very far away,” he said. “We showed that cells at a distance mattered for brain development too.”
Then Levin and his colleagues decided to flip the experiment. Might the brain hold, if not an entire blueprint, then at least some patterning information for the rest of the body, Levin asked — and if so, might the nervous system disseminate this information bioelectrically during the earliest stages of a body’s development? He invited Herrera-Rincon to get her scalpel ready.
Making Up for a Missing Brain
Herrera-Rincon’s brainless Xenopus laevis tadpoles grew, but within just a few days they all developed highly characteristic defects — and not just near the brain, but as far away as the very end of their tails. Their muscle fibers were also shorter and their nervous systems, especially the peripheral nerves, were growing chaotically. It’s not surprising that nervous system abnormalities that impair movement can affect a developing body. But according to Levin, the changes seen in their experiment showed that the brain helps to shape the body’s development well before the nervous system is even fully developed, and long before any movement starts.
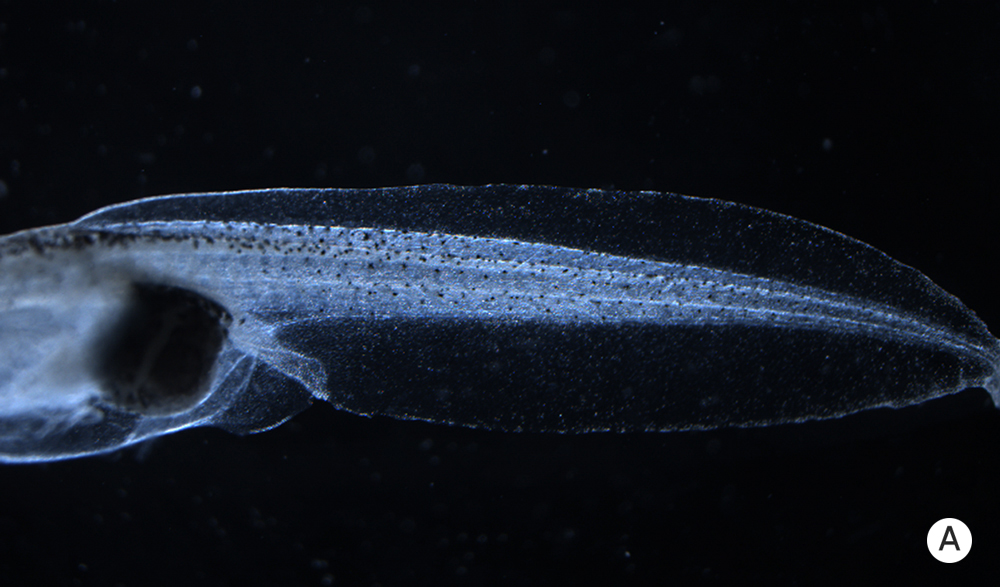
The body of a tadpole normally develops with a predictable structure.
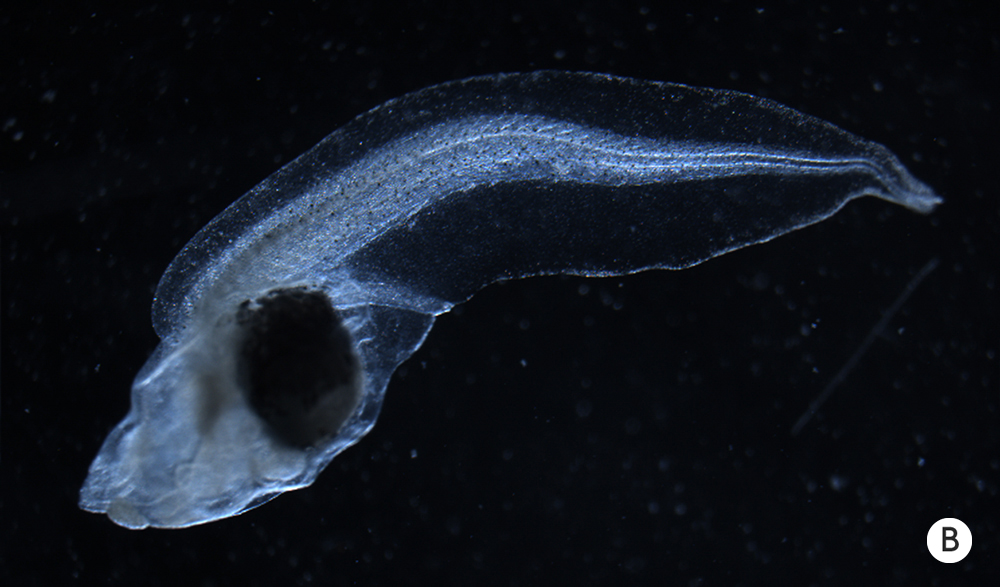
Removing a tadpole’s brain early in development, however, leads to abnormalities in tissues far from the head.
The body of a tadpole normally develops with a predictable structure (A). Removing a tadpole’s brain early in development, however, leads to abnormalities in tissues far from the head (B).
That such defects could be seen so early in the development of the tadpoles was intriguing, said Gil Carvalho, a neuroscientist at the University of Southern California. “An intense dialogue between the nervous system and the body is something we see very prominently post-development, of course,” he said. Yet the new data “show that this cross-talk starts from the very beginning. It’s a window into the inception of the brain-body dialogue, which is so central to most vertebrate life as we know it, and it’s quite beautiful.” The results also raise the possibility that these neurotransmitters may be acting at a distance, he added — by diffusing through the extracellular space, or going from cell to cell in relay fashion, after they have been triggered by a cell’s voltage changes.
Herrera-Rincon and the rest of the team didn’t stop there. They wanted to see whether they could “rescue” the developing body from these defects by using bioelectricity to mimic the effect of a brain. They decided to express a specific ion channel called HCN2, which acts differently in various cells but is sensitive to their resting potential. Levin likens the ion channel’s effect to a sharpening filter in photo-editing software, in that “it can strengthen voltage differences between adjacent tissues that help you maintain correct boundaries. It really strengthens the abilities of the embryos to set up the correct boundaries for where tissues are supposed to go.”
To make embryos express it, the researchers injected messenger RNA for HCN2 into some frog egg cells just a couple of hours after they were fertilized. A day later they removed the embryos’ brains, and over the next few days, the cells of the embryo acquired novel electrical activity from the HCN2 in their membranes.
The scientists found that this procedure rescued the brainless tadpoles from most of the usual defects. Because of the HCN2 it was as if the brain was still present, telling the body how to develop normally. It was amazing, Levin said, “to see how much rescue you can get just from very simple expression of this channel.” It was also, he added, the first clear evidence that the brain controls the development of the embryo via bioelectric cues.
As with Levin’s previous experiments with bioelectricity and regeneration, many biologists and neuroscientists hailed the findings, calling them “refreshing” and “novel.” “One cannot say that this is really a step forward because this work veers off the common path,” Huang said. But a single experiment with tadpoles’ brains is not enough, he added — it’s crucial to repeat the experiment in other organisms, including mammals, for the findings “to be considered an advance in a field and establish generality.” Still, the results open “an entire new domain of investigation and new of way of thinking,” he said.
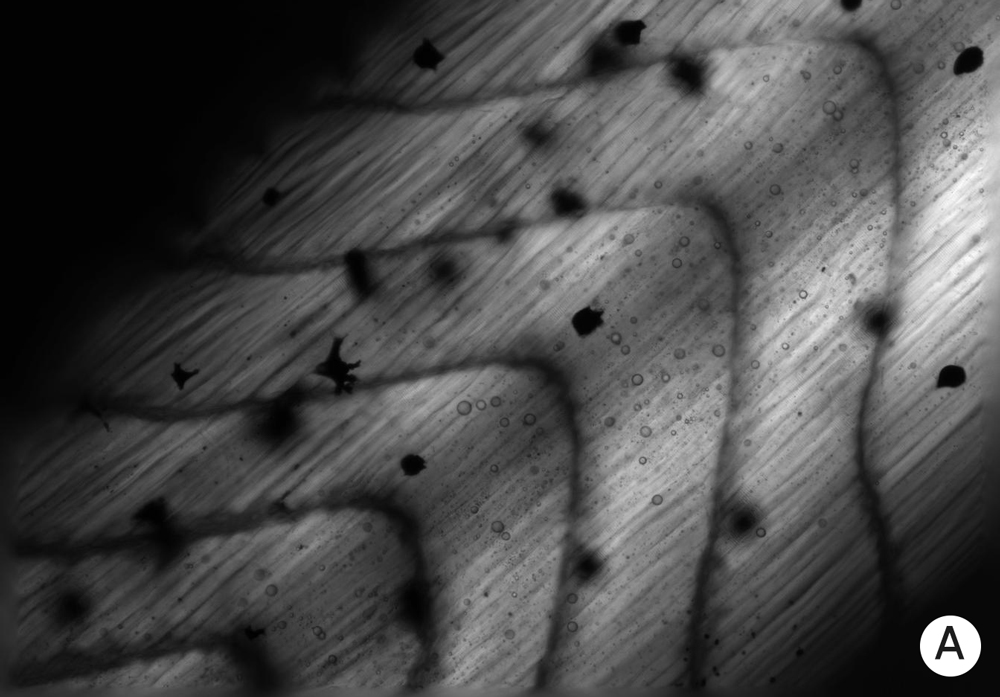
Experiments on tadpoles reveal the influence of the immature brain on other developing tissues, which appears to be electrical, according to Levin and his colleagues. This photo shows the appearance of normal muscle in young tadpoles.
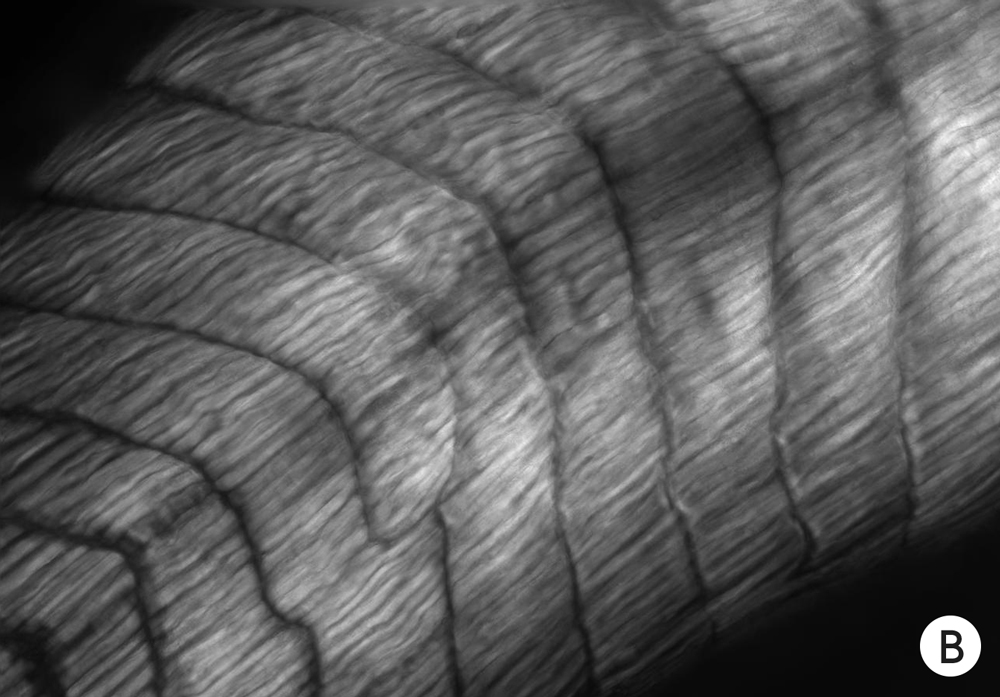
In tadpoles that lack brains, the muscles fail to develop the correct form.
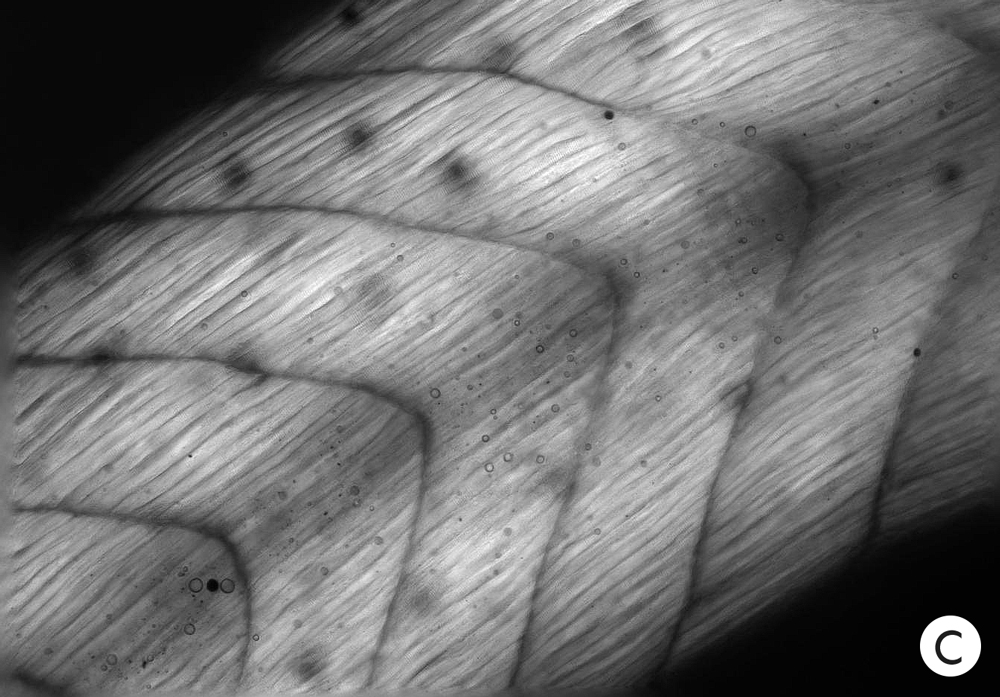
But if the cells of brainless tadpoles are made to express ion channels that can restore the right voltage to the cells, the muscles develop more normally.
Experiments on tadpoles reveal the influence of the immature brain on other developing tissues, which appears to be electrical, according to Levin and his colleagues. Photo A shows the appearance of normal muscle in young tadpoles. In tadpoles that lack brains, the muscles fail to develop the correct form (B). But if the cells of brainless tadpoles are made to express ion channels that can restore the right voltage to the cells, the muscles develop more normally (C).
Courtesy of Celia Herrera-Rincon and Michael Levin
Levin’s research demonstrates that the nervous system plays a much more important role in how organisms build themselves than previously thought, said Min Zhao, a biologist at the University of California, Davis, and an expert on the biomedical application and molecular biophysics of electric-field effects in living tissues. Despite earlier experimental and clinical evidence, “this paper is the first one to demonstrate convincingly that this also happens in [the] developing embryo.”
“The results of Mike’s lab abolish the frontier, by demonstrating that electrical signaling from the central nervous system shapes early development,” said Olivier Soriani of the Institut de Biologie de Valrose CNRS. “The bioelectrical activity can now be considered as a new type of input encoding organ patterning, allowing large range control from the central nervous system.”
Carvalho observed that the work has obvious implications for the treatment and prevention of developmental malformations and birth defects — especially since the findings suggest that interfering with the function of a single neurotransmitter may sometimes be enough to prevent developmental issues. “This indicates that a therapeutic approach to these defects may be, at least in some cases, simpler than anticipated,” he said.
Levin speculates that in the future, we may not need to micromanage multitudes of cell-signaling events; instead, we may be able to manipulate how cells communicate with each other electrically and let them fix various problems.
Another recent experiment hinted at just how significant the developing brain’s bioelectric signal might be. Herrera-Rincon soaked frog embryos in common drugs that are normally harmless and then removed their brains. The drugged, brainless embryos developed severe birth defects, such as crooked tails and spinal cords. According to Levin, these results show that the brain protects the developing body against drugs that otherwise might be dangerous teratogens (compounds that cause birth defects). “The paradigm of thinking about teratogens was that each chemical is either a teratogen or is not,” Levin said. “Now we know that this depends on how the brain is working.”
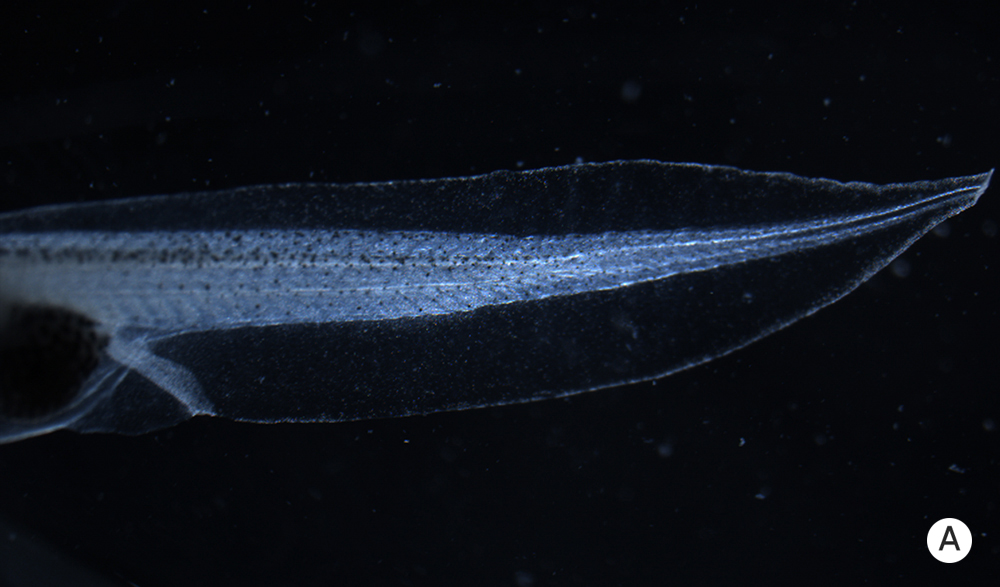
One surprising finding by Levin’s lab is that the brain somehow seems to help protect developing tissues against some environmental influences. Chemicals considered to be benign have no visible effect on intact tadpole development.
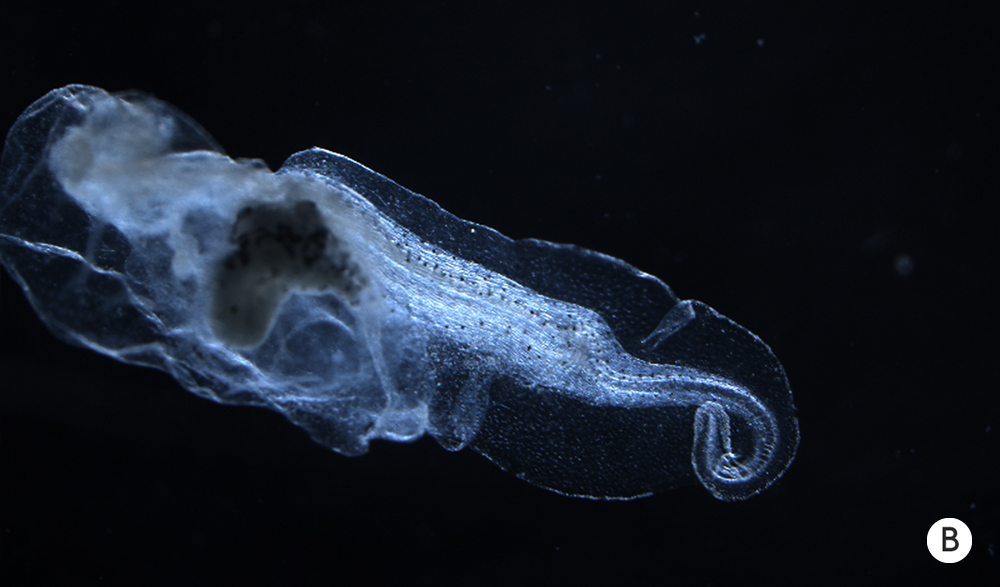
Yet they add to the abnormalities in tadpoles that lack a brain.
One surprising finding by Levin’s lab is that the brain somehow seems to help protect developing tissues against some environmental influences. Chemicals considered to be benign have no visible effect on intact tadpole development (A). Yet they add to the abnormalities in tadpoles that lack a brain (B).
These findings are impressive, but many questions remain, said Adam Cohen, a biophysicist at Harvard who studies bioelectrical signaling in bacteria. “It is still unclear precisely how the brain is affecting developmental patterning under normal conditions, meaning when the brain is intact.” To get those answers, researchers need to design more targeted experiments; for instance, they could silence specific neurons in the brain or block the release of specific neurotransmitters during development.
Although Levin’s work is gaining recognition, the emphasis he puts on electricity in development is far from universally accepted. Epigenetics and bioelectricity are important, but so are other layers of biology, Zhao said. “They work together to produce the biology we see.” More evidence is needed to shift the paradigm, he added. “We saw some amazing and mind-blowing results in this bioelectricity field, but the fundamental mechanisms are yet to be fully understood. I do not think we are there yet.”
But Nuccitelli says that for many biologists, Levin is on to something. For example, he said, Levin’s success in inducing the growth of misplaced eyes in tadpoles simply by altering the ion flux through the local tissues “is an amazing demonstration of the power of biophysics to control pattern formation.” The abundant citations of Levin’s more than 300 papers in the scientific literature — more than 10,000 times in almost 8,000 articles — is also “a great indicator that his work is making a difference.”
The passage of time and the efforts of others carrying on Levin’s work will help his cause, suggested David Stocum, a developmental biologist and dean emeritus at Indiana University-Purdue University Indianapolis. “In my view, his ideas will eventually be shown to be correct and generally accepted as an important part of the framework of developmental biology.”
“We have demonstrated a proof of principle,” Herrera-Rincon said as she finished preparing another petri dish full of beanlike embryos. “Now we are working on understanding the underlying mechanisms, especially the meaning: What is the information content of the brain-specific information, and how much morphogenetic guidance does it provide?” She washed off the scalpel and took off her gloves and lab coat. “I have a million experiments in my mind.”
This article was reprinted on Wired.com.