Brains May Teeter Near Their Tipping Point
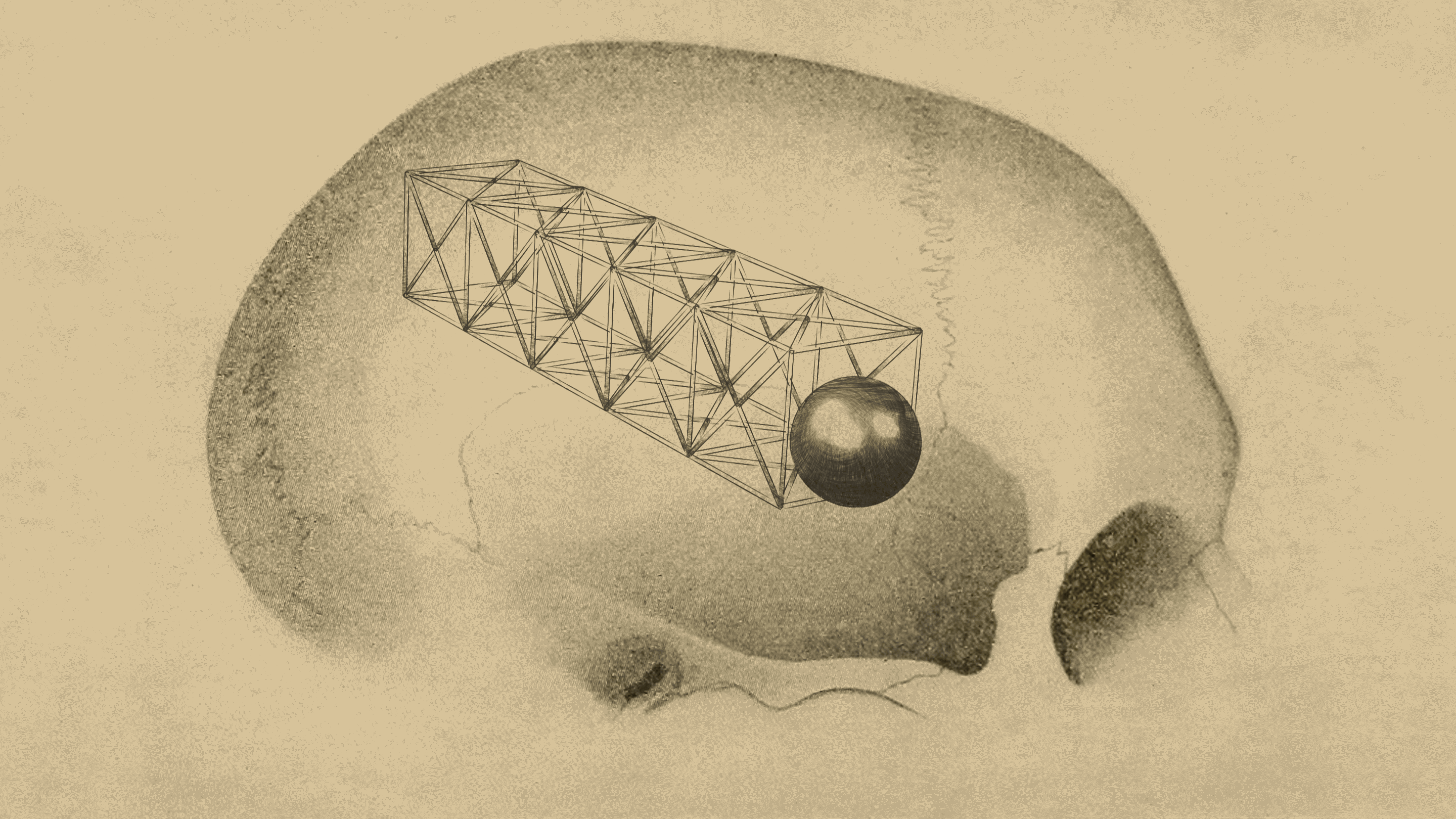
Bill Domonkos for Quanta Magazine
Introduction
Gerardo Ortiz remembers well the time in 2010 when he first heard his Indiana University colleague John Beggs talk about the hotly debated “critical brain” hypothesis, an attempt at a grand unified theory of how the brain works. Ortiz was intrigued by the notion that the brain might stay balanced at the “critical point” between two phases, like the freezing point where water turns into ice. A condensed matter physicist, Ortiz had studied critical phenomena in many different systems. He also had a brother with schizophrenia and a colleague who suffered from epilepsy, which gave him a personal interest in how the brain works, or doesn’t.
Ortiz promptly identified one of the knottier problems with the hypothesis: It’s very difficult to maintain a perfect tipping point in a messy biological system like the brain. The puzzle compelled him to join forces with Beggs to investigate further.
Ortiz’s criticism has beleaguered the theory ever since the late Danish physicist Per Bak proposed it in 1992. Bak suggested that the brain exhibits “self-organized criticality,” tuning to its critical point automatically. Its exquisitely ordered complexity and thinking ability arise spontaneously, he contended, from the disordered electrical activity of neurons.
Bak’s canonical example of a self-organized critical system is a simple sandpile. If you drop individual grains of sand on top of a sandpile one by one, each grain has a chance of causing an avalanche. Bak and colleagues showed that those avalanches will follow a “power law,” with smaller avalanches occurring proportionally more frequently than larger ones. So if there are 100 small avalanches in which 10 grains slide down the side of the sandpile during a given period, there will be 10 larger avalanches involving 100 grains in the same period, and just one large avalanche involving 1,000 grains. When a huge avalanche collapses the whole pile, the base widens, and the sand begins to pile up again until it returns to its critical point, where, again, avalanches of any size may occur. The sandpile is incredibly complex, with millions or billions of tiny elements, yet it maintains an overall stability.
The brain’s tens of billions of neurons form a highly complex, interconnected network. Bak hypothesized that, like a sandpile, the network balances at its critical point, with electrical activity following a power law. So when a neuron fires, this can trigger an “avalanche” of firing by connected neurons, and smaller avalanches occur more frequently than larger ones. In hundreds of papers over the past three decades advancing the idea, researchers have argued that operating at criticality would optimize the brain’s performance by maximizing information transfer and processing. The mystery is how such a noisy system as the brain can maintain such a finely tuned critical state, since another feature of criticality is that the system is most sensitive to any input that could cause it to alter its activity.
For Ortiz, as he listened to Beggs talk about the theory in a physics department conference room at Indiana, “it was obviously a fine-tuning problem,” he said. Criticality “is not something in nature you would find very easily. As soon as there is any perturbation that moves the system away from that fine-tuning, it’s not going to be critical.” Another strike against the critical brain hypothesis is that the textbook definition of criticality in statistical physics requires a system of infinite size. “So the fact that the brain is finite means true criticality is off the table,” said Beggs, who is a professor of biophysics at Indiana.
Yet compelling, if inconclusive, experimental evidence suggests that the brain’s neuronal activity does exhibit hallmarks of criticality. This has led several scientists to propose variations on Bak’s original theory. Ortiz and Beggs, along with graduate students Rashid Williams-García (now a postdoc at the University of Pittsburgh) and Mark Moore, have argued that perhaps the brain inhabits a “quasicritical state.” That is, rather than sitting at a precise critical point, it migrates around a broader but well-defined region, “a volume in phase space where the system can adapt to work efficiently and optimally,” Ortiz said.
Viola Priesemann of the Max Planck Institute for Dynamics and Self-Organization in Göttingen, Germany, has proposed a similar concept. She reasons that the brain could operate in the so-called “subcritical” regime, just below the tipping point. In both scenarios, the brain operates near the critical point, rather than being precariously poised there. This arrangement offers much-needed stability while still enabling highly efficient information transfer and processing.
The new proposals please one of the field’s earliest experimental pioneers, Dietmar Plenz of the National Institute of Mental Health, who has found evidence of power laws in the neuron firing patterns of monkeys. Whereas 15 years ago, criticality was not yet deemed a serious possibility, “now I think criticality is on the map,” Plenz said. “I think we are seeing that there’s a regime of cortical dynamics that is close to criticality. This is huge progress, because now we are not talking anymore about whether or not the brain is critical, but about in what specific aspect is it critical.”
Not Quite Critical
Scientists often use the same model for criticality as they do for nuclear chain reactions. In nuclear fission, a fission event gives off two particles, and they each give off two more, and so on, yielding a branching ratio (the expected number of descendants from a single event) of two. Such a system goes “supercritical” to produce an atomic bomb. Meanwhile in a “subcritical” system, the branching ratio is less than one, and so the chain reaction fizzles out. In a critical system, the branching ratio will be exactly one, setting off a sustained nuclear reaction capable (for example) of running a power plant indefinitely. Similarly, if the brain is truly critical, there will be a power-law distribution of avalanche sizes, but one neuron should, on average, activate one other neuron.
If the brain were extremely subcritical, according to Beggs, incoming signals would get damped and have no impact. “It would be like trying to talk to someone who is asleep or drunk,” he said. In a supercritical brain, incoming signals would get lost in a frenzy of electrical activity, and the effect would be like trying to talk to a seizure victim. Beggs and others argue that the neural network is most sensitive to incoming signals at the critical point. There, a chain of active neurons allows information to spread from one brain area to another without dying out prematurely or exploding.
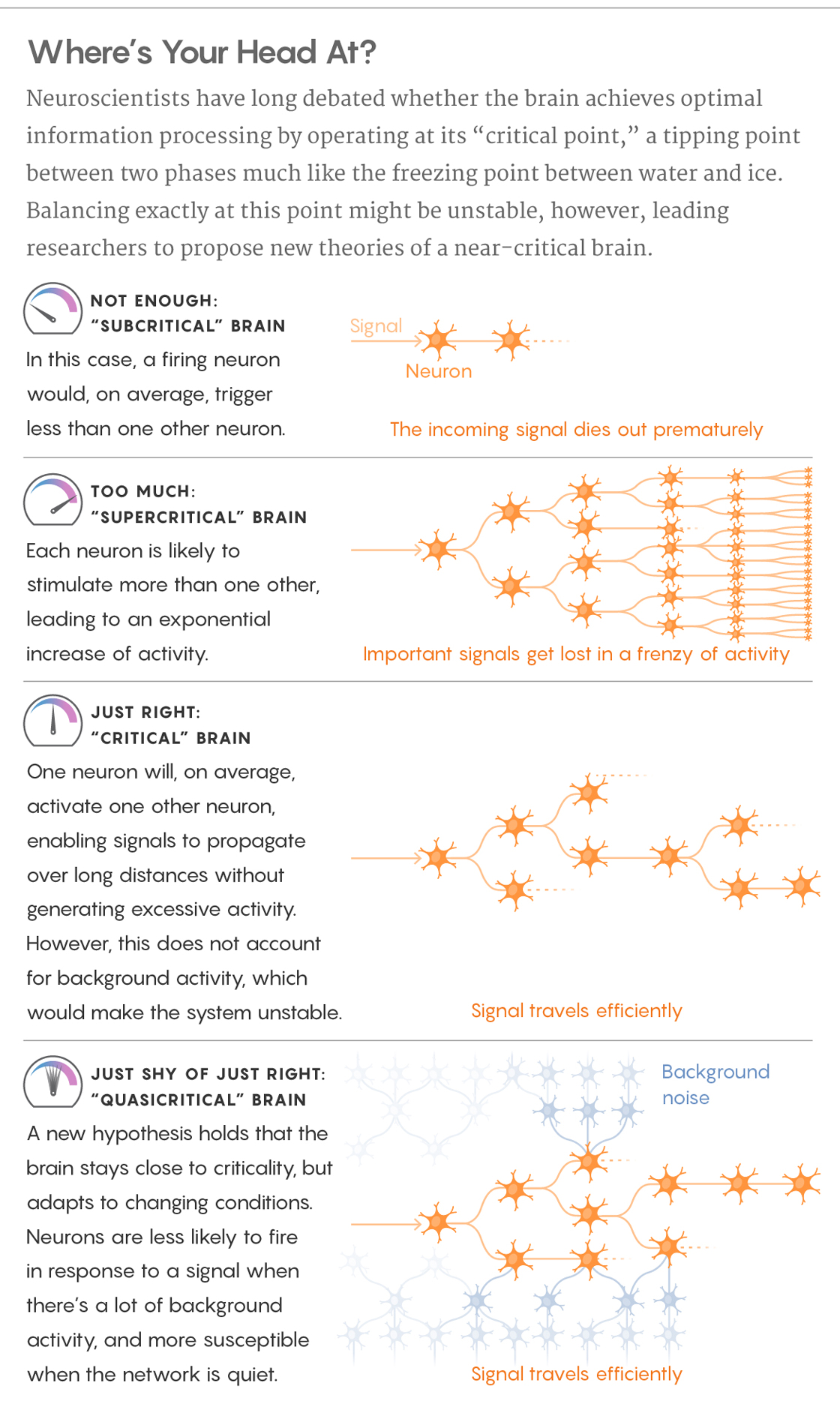
Lucy Reading-Ikkanda/Quanta Magazine
Priesemann suggests that the brain is slightly subcritical. Being right at the critical point comes with the risk of tipping over into the supercritical regime, so she sees subcriticality as a kind of safety zone for the brain, which can move toward, or away from, the critical point as needed to best adapt to the conditions at hand. For intense cognitive tasks that require integration of a lot of information, for example, the brain would benefit by being as close to criticality as possible. But if circumstances require a faster, more intuitive response, it’s better to be farther below the critical point. “Depending on whether you have to integrate information for a solution or should just quickly react, the ‘sweet spot’ can be very different,” Priesemann said.
In a paper that appeared this week in Nature Communications, she and a colleague formulated a new, more accurate method for estimating the branching ratio, verified by comparing its predictions to models with known ratios. When she applied this new method to cortical spike recordings from rats, cats and monkeys, she found that all turned out to have branching ratios of 0.98 or 0.99 — within 1 or 2 percent of true criticality.
While Priesemann offers this as evidence for the subcritical brain hypothesis, Beggs thinks it’s also compatible with his and his collaborators’ competing theory that the brain is quasicritical. Quasicriticality is similar to subcriticality in that the brain does not operate exactly at the critical point, but over a broader region near that point, enabling it to be more readily adaptable. But quasicriticality very precisely defines that region of space.
The starting point is the observation that the brain clearly doesn’t operate exactly like a sandpile or a nuclear chain reaction. In Bak’s sandpile model, when an avalanche occurs, it runs its course before the next grain is dropped onto the pile. This separation of timescales is needed for the traditional hallmarks of criticality, like power-law distributions, to occur. But the brain simply doesn’t work like that. This was the key insight that inspired Ortiz, Beggs and their students to devise their concept of quasicriticality.
“The cortex is not silent, just waiting to be driven by a stimulus; it is churning with activity,” Beggs said. “Each cortical region is getting inputs from multiple other regions.” Unlike in the sandpile model, neurons don’t fire one by one; neuronal avalanches of all sizes flow into one another, making it extremely difficult to differentiate them.
So rather than looking at power-law distributions in neuronal avalanches, Beggs and Ortiz characterize quasicriticality in terms of “susceptibility” — a function that describes how sensitive a given system is to an incoming stimulus, whether it takes the form of neuronal firings or grains of sand.
That sensitivity depends in large part on the extra spontaneous activity in the brain, which adds noise to the system and dampens susceptibility. When the brain is very active, it’s like trying to discern a signal in a room filled with background chatter. In a completely quiet room — akin to Per Bak’s sandpile, entirely driven by external signals — it’s easy to pick up the signal. When the network is quasicritical, Beggs and Ortiz argue, the balance is just right: An incoming signal will be detected and can spread throughout the cortex without being prematurely dampened or hopelessly distorted by noise.
What’s the Mechanism?
This all assumes there is some mechanism enabling the brain’s network of neurons to stay close to criticality. It’s not inconceivable that the brain would have a means of self-regulating, given that the body already employs various biological mechanisms to regulate blood pressure, breathing rate and pH levels, among other things. But the specifics of such a mechanism, if one exists, are still speculative.
There’s some experimental evidence that when connections between neurons in the brain become stronger via repeated firings, this is counterbalanced by the weakening of other connections. “When you have an excess of activity, the brain sets up molecular mechanisms that inhibit the activity to bring it back down,” said Lucilla de Arcangelis, a physicist at the University of Campania in Italy. “This is very well-accepted now, that the brain works close to the balance of excitation and inhibition.” That doesn’t necessarily mean the brain is critical, however.
Priesemann is developing her own theory about a possible mechanism for keeping the brain subcritical, known as “homeostatic plasticity.” The idea is that every neuron has a target firing rate, and the neuron adjusts its activity as necessary to maintain that target. For example, if the target firing rate is once per second, and a neuron fires less often than this for a long enough period, it will increase its excitability in response, meaning it will respond more strongly to input until its firing rate rises to the target rate. This would let the brain “tune” its immense network of neurons, moving closer to, or farther from, the critical point depending on the input strength.
Some researchers suspect that the brain isn’t critical at all, and that the signs scientists have been using to identify the phenomenon, like power-law distributions, can be mimicked by other processes. That’s the stance of Alain Destexhe, a theoretical neuroscientist at the National Center for Scientific Research (CNRS) in France and one of the most vocal critics of the hypothesis. There’s a running joke that physicists see power laws everywhere they look. Destexhe says power laws do indeed show up in nature, but “whether they stem from criticality — that’s another, very important question.”
Destexhe thinks the brain is more akin to an “asynchronous irregular” state, in which neurons fire with such weak correlations that they fire almost randomly. Last year, he found that artificial neural networks with just a few thousand neurons can automatically generate such activity, and the data from these networks resemble those collected from brain studies. Furthermore, the asynchronous irregular states showed power-law behavior when researchers measured the neuron activity in just the right way. “This was bizarre, since the system was not supposed to be critical,” he said.
When Destexhe and his colleagues replaced the neurons in their model with randomized neurons, the power-law behavior remained. For him, this is a strong argument that we cannot rely only on power laws and related phenomena as evidence of a critical or near-critical state. Some other kind of test is needed.
The quasicriticality hypothesis comes with a clear prediction that Beggs and colleagues are now experimentally testing. They are measuring susceptibility using high-density microelectrode arrays adapted from the silicon strip detectors used in particle accelerators like the Large Hadron Collider. Their version has roughly 500 electrodes simulating active neurons, enabling them to model the varying levels of spontaneous neural activity by artificially stimulating the arrays with increasing intensity. If, when they analyze the resulting data, the susceptibility decreases along the predicted line as the levels increase, that would be solid evidence that Beggs and Ortiz are on the right track. And if it doesn’t? “Then this is not true. Period,” Ortiz said.
“It seems a reasonable idea, but ultimately it’s an experimental question,” Beggs said. “It would break my heart, but there have been some really beautiful ideas that have just been wrong. This might be one of them.”