Can Darwinian Evolution Explain Lamarckism?
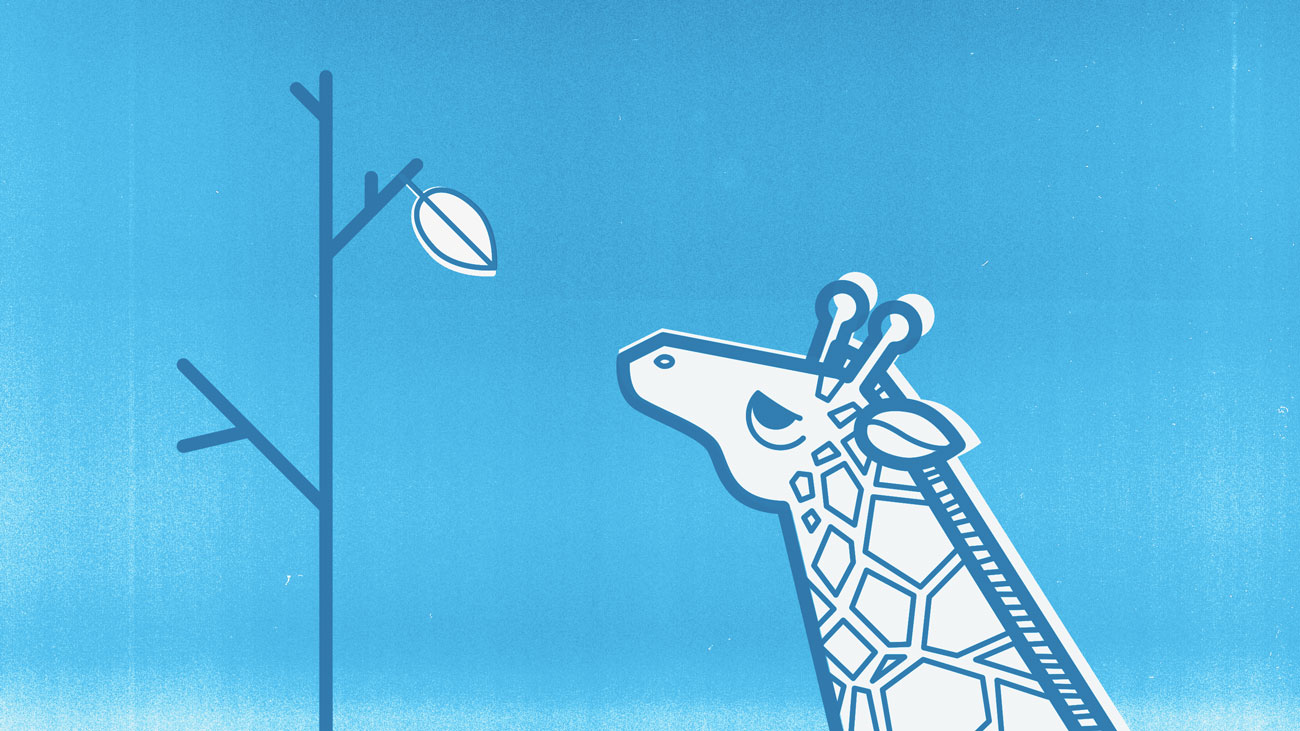
Olena Shmahalo/Quanta Magazine
Introduction
If you took a high school biology class, you’re probably familiar with Jean-Baptiste Lamarck’s theory of evolution and its emphasis on the “inheritance of acquired characteristics” — think giraffes stretching their necks longer to reach the leaves high in trees. In textbooks Lamarck’s theory is often presented as a rival to Charles Darwin’s theory of evolution by natural selection. The simplistic storyline is that the two theories battled it out in the 19th century and that Darwinism won, leading to Lamarckism’s demise and the rise of what biologists call the Modern Synthesis.
But recent discoveries have exhibited a remarkably Lamarckian flavor. One example is the CRISPR-Cas system, which enables bacteria to pass information about viruses they have encountered to their offspring. There are also clear-cut examples of transgenerational epigenetic inheritance, in which higher animals affected by environmental factors transmit favorable genetic changes to their offspring across generations. Such mechanisms make sense to us as designers: An animal should pass on, in its genes, information that it has gained about the environment. Such discoveries have sparked debate about the possibility of an update to the Modern Synthesis. Is there a role for Lamarckian mechanisms in modern evolutionary theory?
At the level of specific mechanisms, yes. At a deeper level of causes, though, the answer is a resounding “no” — natural selection reigns supreme. How can that be, you ask? The simplistic juxtaposition of Darwin and Lamarck in elementary biology courses is a false equivalence. If Lamarckian patterns of inheritance do exist, and are indeed beneficial to the organism (that is, they are evolutionary adaptations), then the only way that they could have arisen and been maintained over evolutionary time is by Darwinian natural selection. There’s simply no way around it. Our puzzles for this month explore a simple version of transgenerational epigenetic inheritance and show how natural selection across multiple generations can, under certain conditions, favor the individuals who possess such mechanisms.
First, let’s recap the differences between Lamarck’s and Darwin’s ideas by revisiting that frequently cited example: the giraffe’s long neck. According to Lamarck, the giraffe got its long neck because its ancestors stretched theirs to eat leaves that were just out of reach. This stretching of the neck was passed on to their offspring, over generations, until it reached its current length. On the other hand, the mainstream Darwinian view is that, within the giraffe’s ancestors, there was a variation in neck sizes, as there is in any population. The giraffes with longer necks were more successful in getting food and produced more longer-necked offspring. Long necks were thus “selected for” in every generation, gradually lengthening the giraffe’s neck over evolutionary time. The reason we no longer believe Lamarck’s version is that genetic material is transmitted to the next generation through germ cells, and most acquired changes simply do not influence germ cells; they are, to a large extent, isolated from the environment. If this were not true, mice whose tails are cut off for many generations, as the scientist August Weismann tried to do, would be born without tails. (They are not!) Furthermore, Jewish and Muslim males would be born without foreskins. (Despite many generations of circumcision, mohels can still make a living.)
But there is an obvious way that germ cells can be affected by the environment — when the changes are caused by ill health and are deleterious. Infections, toxins or just old age can affect germ cells and produce offspring that are less healthy in various ways. We don’t really think of this as unusual, or as anything to do with evolution. But what if the changes turned out to be good? A famous Swedish study of 300 people who were exposed to famines in the early 1900s, the Överkalix study, showed a remarkable result. The amount of food supply that a person’s grandparents were exposed to in their pre-pubertal years had a measurable effect on that person’s cardio-vascular risk two generations later. In one specific association, men who were were exposed to a poor food supply at a critical age were found, two generations later, to have conferred a measurably lower risk of cardiovascular death to their grandchildren. Apparently it helps to have had a paternal grandfather who starved between the ages of 9 and 12! Similar changes have been found in animal experiments. For instance, survivors of famines among nematode worms are smaller and less fertile than normal worms, but they acquire a toughness that lasts at least two generations. What’s more, scientists have also found that such transmission across generations does not happen through a change in DNA coding in the genes in the manner heredity usually works, but rather through “epigenetic” mechanisms such as the inactivation of certain genes by the attachment of methyl groups (DNA methylation) or through changes in the configuration of the protein that packages the DNA (histone modification). These non-standard “Lamarckian” mechanisms certainly do have the potential to confer good or adaptive changes on individuals, and they could spur evolution. But how could they have arisen, and how are they maintained? Well, it has to be by random variation and standard Darwinian natural selection, of course! Let’s explore this in our puzzle questions.
Question 1:
Imagine there exists an animal that has a new generation every year. Every normal individual has an average of 1.6 surviving offspring in a normal year, which can be defined as the animal’s fitness (let’s call it f), after which the animal dies. During a famine year, f falls to 1.3. Now suppose there are a bunch of smaller individuals whose f values are 1.5 in normal years but 1.35 in famine years: their smaller food requirement helps them survive famines better. How long would a famine have to last for the small individuals to do better than normal ones? How many famine years before small individuals make up 90 percent of the population?
Question 2:
Suppose there exists an initially normal mutant group of individuals called Epi2s, whose germ cells are affected by a year of famine in such a way that their progeny changes to the small type for two generations before they revert back to normal in the third generation, through epigenetic mechanisms. Consider a 13-year period that starts and ends with normal years but has a one-year famine, two two-year famines and a three-year famine in between. Which of the three groups (normal, small, Epi2s) will be most successful? Are there famine patterns in which Epi2s overwhelm the other two groups over the very long term?
Question 3:
Let’s add another type of animal to the above: the Epi1s, which like the Epi2s switch to small progeny after a famine, but in this case the progeny revert back to normal after just one generation. Over a period of 20 years, can you come up with a “famine-year schedule” such that all four types of animals (normal, small, Epi1s and Epi2s) exist in virtual equilibrium over this time period?
As these vignettes show, it does not matter to natural selection whether characteristics are controlled by genetic or epigenetic mechanisms. What matters is the fitness advantage that is selected by an organism’s environment. If the conditions that confer a fitness advantage on a given group last long enough and select that group across multiple generations, then that group will dominate the population, and the species characteristics will change. So if there is a sizable subset of a population that exhibits advantageous epigenetic inheritance, natural selection is very likely to maintain it. On the other hand, if epigenetic modifications in a population are deleterious, natural selection will eliminate it. There is no top-down, purposeful information passing across generations here, no matter how sensible that seems to us. Based on these considerations, can you speculate how the elegant information transfer across generations that is embodied by the CRISPR-Cas system in bacteria could have evolved?
So deep and so inexorable is the blind, bottom-up process of natural selection in evolution that there is no way to contain its potency, and no rival mechanism for creating adaptation. It is no wonder that the philosopher Daniel Dennett has likened natural selection to a “universal acid” that cannot be contained. Natural selection and its analogs in non-biological spheres may well be the major — or only — processes that create complex novelty at all levels of the universe. And that includes the complex novelty created by us.
Editor’s note: The reader who submits the most interesting, creative or insightful solution (as judged by the columnist) in the comments section will receive a Quanta Magazine T-shirt. And if you’d like to suggest a favorite puzzle for a future Insights column, submit it as a comment below, clearly marked “NEW PUZZLE SUGGESTION.” (It will not appear online, so solutions to the puzzle above should be submitted separately.)
Note that we may hold comments for the first day or two to allow for independent contributions by readers.
Update: The solution has been published here.