Gas Giants’ Energy Crisis Solved After 50 Years
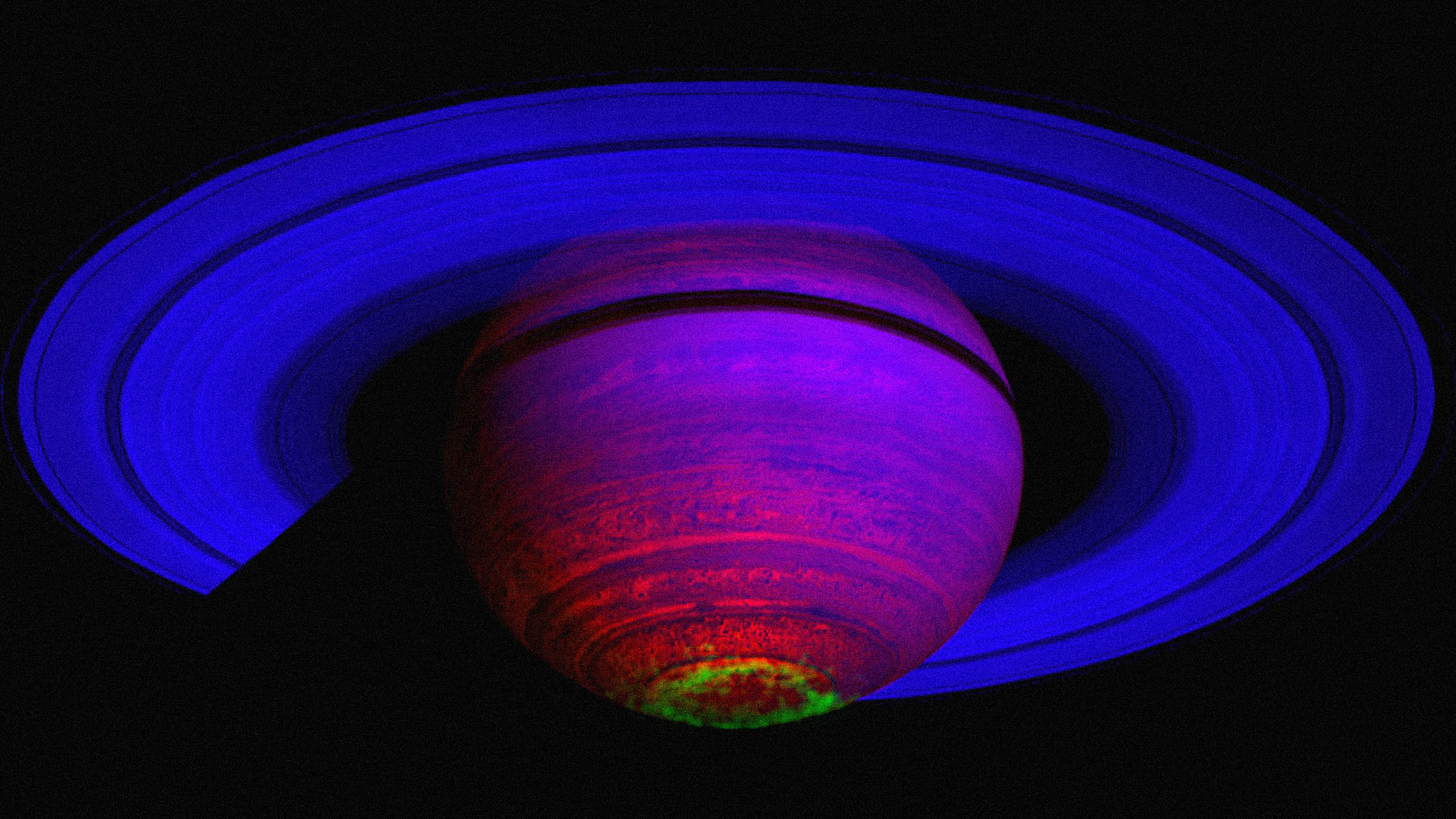
Saturn’s auroras are clearly visible in this composite image made by NASA’s Cassini spacecraft. Blue light corresponds to reflected sunlight, red to the planet’s own heat, and green to emissions from hydrogen ions in the aurora.
Introduction
Living as they do in the distant, sun-forsaken reaches of the solar system, Jupiter and Saturn, the gas giants, and Uranus and Neptune, the ice giants, were always expected to be frosty realms. But when NASA’s Voyager spacecraft sailed past them in the late 1970s and 1980s, scientists found that all four worlds were running planetary fevers — a revelation as jarring as finding a bonfire inside your freezer.
Follow-up observations by ground-based telescopes and the Galileo and Cassini spacecraft demonstrated that their planet-wide fevers have persisted through time. Their planetary pyrexias are acute: Jupiter’s lower latitudes, for example, should be a frigid −110 degrees Celsius. Instead, the atmosphere there cooks at 325 degrees. What incognito incinerator is behind this? And how is this unknown heat source warming not just a single spot on the planet, but the entire upper atmosphere?
Scientists have tried to explain this “energy crisis,” but have remained “confused for about 50 years,” said James O’Donoghue, a planetary astronomer at the Japan Aerospace Exploration Agency. Now two papers have conclusively revealed where all that heat is coming from: Jupiter and Saturn’s northern and southern lights — their auroras.
The results come from detailed measurements of both gas giants’ upper atmospheres. Saturn’s atmospheric temperature was taken by the Cassini spacecraft during the maneuvers that ultimately plunged it into the planet; Jupiter’s was stitched together using a telescope atop a giant Hawaiian volcano. Both show that the atmospheres are hottest near the auroral zones below both magnetic poles. As you approach the equator, the temperature drops off. Clearly, the aurora is bringing the heat — and, as with a radiator, that heat decreases with distance.
A solution to the energy crisis may have far-reaching ramifications. Planets — from those in our own solar system to those orbiting distant stars — don’t always keep their atmospheres. Many gassy envelopes are destroyed over time, in some cases turning giant worlds into tiny, uninhabitable husks. Researchers want to be able to distinguish these from habitable, Earth-like planets. If we hope to do so, said Zarah Brown, a researcher at the University of Arizona, “one of the major parameters that you would want to know is the temperature of the outer atmosphere, since that’s where gas is lost to space.”
Alien Auroras
Earth’s northern and southern lights aren’t yet completely understood, but the basics are clear.
The sun shoots volleys of magnetic fields and energetic particles into space. When these volleys — better known as the solar wind — reach our planet, they interact with Earth’s own magnetic bubble, which is known as the magnetosphere. The energetic particles then spiral down to the planet’s north and south magnetic poles. There, they ping off gas atoms and molecules in the upper atmosphere. Those impacts temporarily energize the gases, which emit visible flashes of light.
In general, auroras require three ingredients: a source of energetic particles, a magnetic field and an atmosphere. Jupiter and Saturn have all three, but neither planet’s auroras are quite like Earth’s.
Earth’s magnetic field comes from the churning of liquid nickel-iron alloys deep below our feet. But the gas giants don’t have liquid-iron cores. Instead, the planets’ immense gravity squeezes colossal volumes of liquid hydrogen in their outer cores so hard that the hydrogen’s electrons pop free. The process turns the hydrogen into a magnetism-generating metal.
Because these maelstroms of metal hydrogen are so immense, the gas giants’ magnetospheres make Earth’s look lilliputian. Jupiter’s magnetosphere “is the biggest structure in the solar system,” said O’Donoghue. “Its tail goes down to Saturn, and possibly beyond.”
The gas giants also can’t rely on a plentiful supply of energetic particles, or plasma, from the solar wind, which dissipates with increasing distance from the sun. Instead, they rely on acts of volcanic alchemy.
Jupiter gets most of its plasma from its moon Io, the most volcanic object known to science. Io’s near-constant magmatic eruptions jettison an abundance of volcanic material into space; there, it bathes in sunlight, becomes electrically excited, then showers down onto Jupiter. Most of Saturn’s plasma comes from Enceladus, a mirrorlike icy moon that fires spectacular jets of frigid watery matter into space.
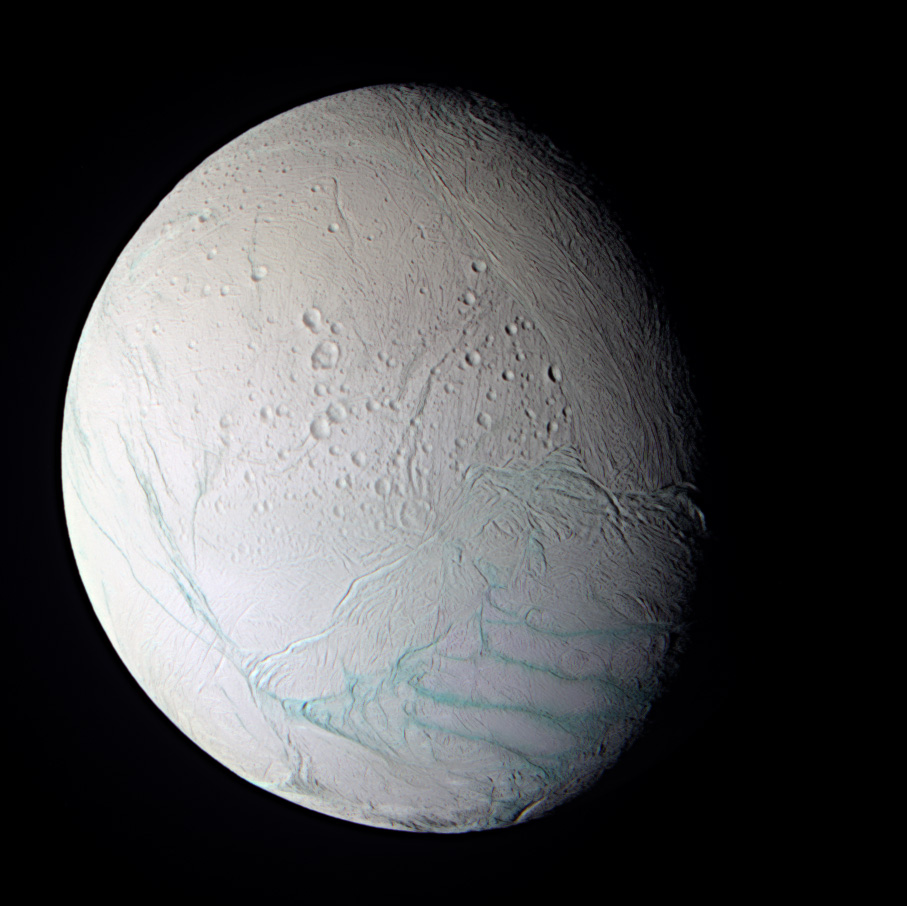
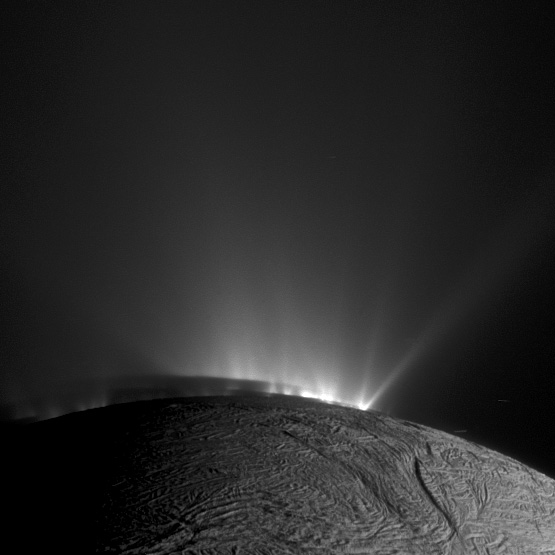
Saturn’s icy moon Enceladus hides a global ocean of liquid salty water beneath its crust. Its geysers spray water ice and vapor hundreds of kilometers into space.
These plasmas shoot into the planets’ expansive magnetospheres, which accelerate them into the poles. There, the charged particles in the plasma collide with gas molecules in the atmosphere.
Auroras on Saturn emit mostly ultraviolet light; on Jupiter, they’re in both ultraviolet and infrared wavelengths. But the processes that make light aren’t the same as those that make heat. In this case, “it’s all about friction,” said O’Donoghue.
Plasma flows to the planets’ magnetic poles via field lines — magnetized tendrils that stretch far into space. These tendrils and their streams rotate along with the planet. But they sometimes struggle to keep pace. Jupiter, for example, rotates once every 10 hours. When those streams of plasma lag behind the planet’s rotation, Jupiter’s powerful westerly winds push through them. The drag of these winds on the slow-moving plasma streams creates friction. And that friction makes heat — perhaps, in the case of Jupiter, 125 times more heat than the planet gets from the sun. “That’s kind of nuts,” said O’Donoghue.
It’s not surprising, then, that astronomers have been wondering if the auroras are the source of those planetary fevers. “For decades, it was obvious that there was plenty of energy in the aurora,” said Luke Moore, a senior research scientist at Boston University. But in order to navigate from suspicion to certainty, astronomers needed a map: specifically, a heat map of the gas and ice giants’ upper atmospheres. With it, they could see if the highest temperatures could be superimposed on the auroras, and if this heat was diffusing over the entire planet.
The first map came from a final act. In April 2017, after 13 years in orbit around Saturn, NASA’s Cassini spacecraft was commanded to do something remarkable: make 22 orbits of the planet while repeatedly diving between it and its rings. The so-called Grand Finale, which ended on September 15, 2017, when the spacecraft burned up in Saturn’s clouds, gave Cassini a close-up view of the world like no other.
As Cassini passed close to Saturn, it peered through the planet’s atmosphere at the bright stars beyond. The light from these stars appeared to change depending on the density of the atmosphere that the light passed through. A gas’s density and temperature are related, so researchers used dozens of these measurements, known as stellar occultations, to produce detailed heat maps for both the day and night sides of Saturn’s upper atmosphere.
Published last year in Nature Astronomy, the heat maps showed a thermal spike around the auroras, and a gentle drop-off in temperatures toward the equator.
It certainly seemed as if the auroras were responsible. But “if our theory of energy redistribution on Saturn is correct, it would have to work on Jupiter as well,” said Brown, who was the lead author on the Saturn study.
Now, due to the work by O’Donoghue and his colleagues, it appears that it does.
Attributing Jupiter’s upper atmospheric fever to its own auroras also required a heat map. But making such a map is far from easy. The planet’s chaotic upper atmosphere changes from week to week. You can’t just take a measurement near the poles on one night, then come back a few weeks later and compare it with a measurement near the equator. In time, the skies will shift significantly, and evidence of any heat flows will be lost.
What researchers needed was a global heat map made during a relatively brief moment in time — one that showed the flow of heat over several hours.
O’Donoghue, Moore and company turned to the Keck Observatory atop Hawai‘i’s dormant Mauna Kea volcano. They used it to observe Jupiter in infrared light over two nights — April 14, 2016, and January 25, 2017 — for five hours apiece. During the course of each night they created a high-resolution heat map of Jupiter’s day side. Both maps clearly showed temperatures peaking around the auroral zones, hitting a staggering 730 degrees Celsius. This thermal zenith gradually declined as you approached the equator, where the mercury still hit an impressive 325 degrees.
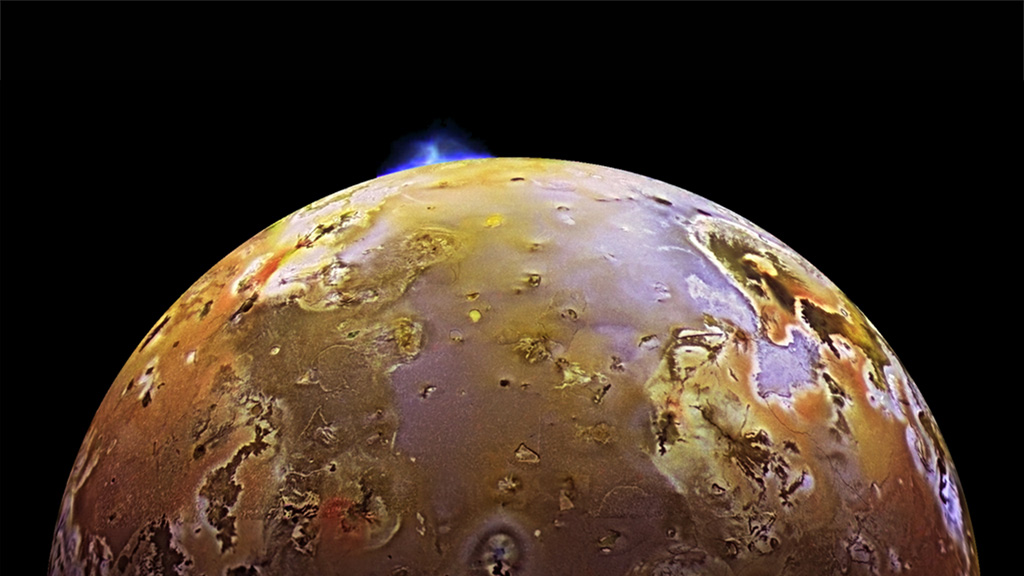
Jupiter’s moon Io is the most volcanically active place in the solar system. The constant gravitational tug of Jupiter and its planets distorts Io’s surface, leading to eruptions such as this one caught by NASA’s Galileo spacecraft.
NASA/JPL/DLR
Their results, currently available in a preprint that has been accepted to Nature, harmonize with what Cassini saw at Saturn. [Editor’s note: That paper has now been published.] The results have been taken as hard proof that the auroras can solve the energy crisis. “It’s a big step forward, seeing that it’s auroral heating,” said Rosie Johnson, a space physics researcher at Aberystwyth University in Wales who wasn’t involved with either paper.
Licia Ray, a space and planetary physics researcher at Lancaster University in England who is also not involved with either paper, praises the Saturn study’s rigorous data set. But she is less convinced by the Jupiter paper. “They’re only using two nights of data, and I find that to be an issue,” she said. But despite her misgivings, “I think the temperature gradient result [at Jupiter] probably will hold, because they’ve seen it at Saturn,” she said.
Having comparatively few observations is “a fair concern, because these are very dynamic places, these giant planets,” said Moore. Additional nights of Jupiter observations have been collected and are currently being processed.
In any event, most independent researchers seem convinced that the planet-wide fevers are down to the auroras. These papers provide “a really nice confirmation that what we suspected was happening is actually happening,” said Leigh Fletcher, a planetary scientist at the University of Leicester in England who was not involved with the work. “Energy is leaking from the auroral domain down into the lower latitudes.” The question is: How?
The Wicked Western Winds
The majority of atmospheric circulation models struggle to move heat from the aurora through Jupiter and Saturn’s screeching westward winds to the equator — at yet, their heat maps show that these tempestuous hurdles are somehow being overcome.
One potential solution was inspired by Cassini’s observations. Cassini discovered that, on occasion, a disturbance to a lower layer of Saturn’s atmosphere can cause that layer to migrate to the upper atmosphere. Such an inversion may disrupt and slow down the upper atmosphere’s powerful westward winds — perhaps enough to allow the auroral heat to leak through.
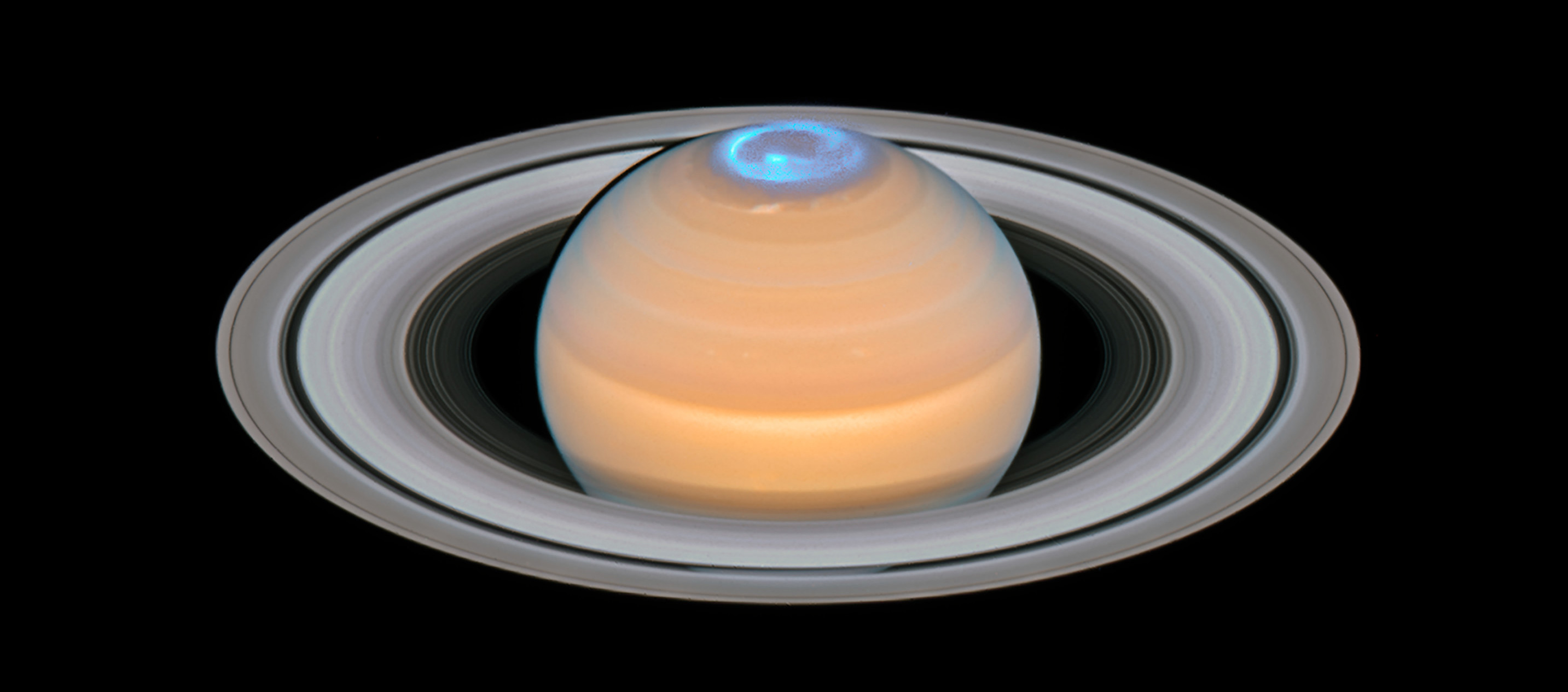
The aurora as seen over Saturn’s north pole.
In theory, this mechanism could apply to Jupiter too. But the upper atmospheres of gas giants lack clouds — clear markers of movement — which makes studying winds there “fiendishly challenging,” said Fletcher. For now, this part of the energy crisis remains a riddle without a resolution.
O’Donoghue’s team suspects that a second process might help distribute heat around Jupiter. Occasionally, intense solar wind activity will exert pressure on Jupiter’s magnetosphere, squeezing it. Prior work indicated that when this compression happens, Io’s plasma streams can get quickly pushed into the upper atmosphere. The additional plasma gives those powerful westward winds more to push through, which could produce a heating spike.
Such a spike may have been seen during the recent surveys. Around the time of the January 25, 2017, observation, when solar wind activity was relatively high, the already hot upper atmosphere spiked in temperature. The team simultaneously spotted a curious high-temperature structure moving from the auroral zones toward the equator. These phenomena were not seen during the April 14, 2016, observation, when the solar wind activity was relatively quiet.
The team speculates that a burst of solar wind activity in early 2017 may have pinched the planet’s magnetosphere. But other factors could also be in play. Ray speculates that an uptick in volcanic activity on Io may provide an alternative explanation. Without more observations, they can’t be certain one way or the other, said O’Donoghue.
Despite these lingering quandaries, the conclusive identification of the auroras as Jupiter and Saturn’s atmospheric arsonists has significantly bolstered our understanding of these worlds. Uranus and Neptune, however, remain shrouded in a thick fog of uncertainty. They have different atmospheres, magnetic fields and rotational behaviors — “they’re wacky,” said Brown — meaning what works for the gas giants may not work for the ice giants. They’re so far away that we struggle to see either in detail using Earth’s telescopes, and it looks as though they won’t be visited by another spacecraft for the foreseeable future. Until that day comes, these distant realms will remain strangers, both afflicted with planetary fevers that we have yet to fathom.
Update: August 4, 2021
When this article first appeared, the research describing Jupiter’s auroras was available only as a preprint. It has now been published in Nature.