Cells Talk in a Language That Looks Like Viruses
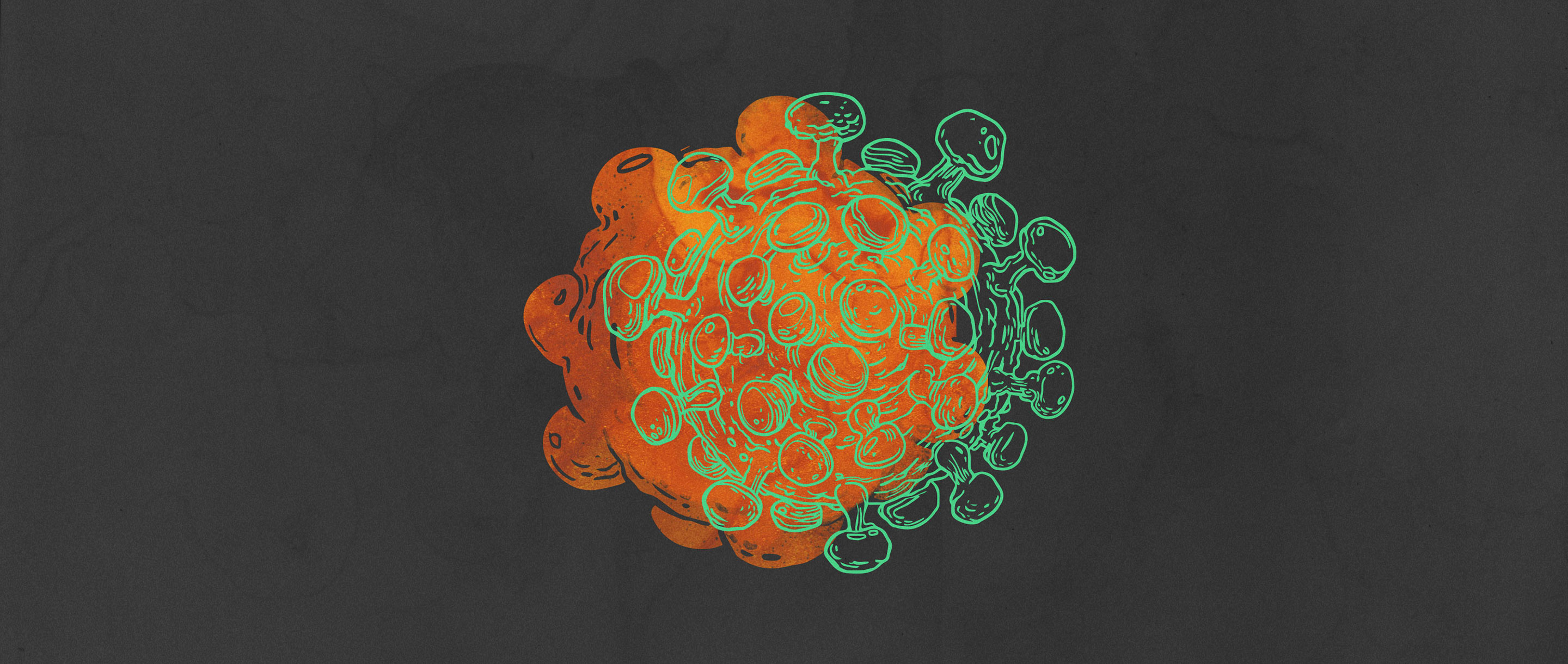
Is it a live virus? An extracellular vesicle that delivers information about a cell? An incomplete and defective virus particle? A vesicle carrying viral components? Classifying the closely related particles that cells release can be a challenge.
Olena Shmahalo/Quanta Magazine
Introduction
For cells, communication is a matter of life and death. The ability to tell other members of your species — or other parts of the body — that food supplies are running low or that an invading pathogen is near can be the difference between survival and extinction. Scientists have known for decades that cells can secrete chemicals into their surroundings, releasing a free-floating message for all to read. More recently, however, scientists discovered that cells could package their molecular information in what are known as extracellular vesicles. Like notes passed by children in class, the information packaged in an extracellular vesicle is folded and delivered to the recipient.
The past five years have seen an explosion of research into extracellular vesicles. As scientists uncovered the secrets about how the vesicles are made, how they package their information and how they’re released, it became clear that there are powerful similarities between vesicles and viruses.
A small group of researchers, led by Leonid Margolis, a Russian-born virologist at the National Institute of Child Health and Human Development (NICHD), and Robert Gallo, the HIV pioneer at the University of Maryland School of Medicine, has proposed that this similarity is more than mere coincidence. It’s not just that viruses appear to hijack the cellular pathways used to make extracellular vesicles for their own production — or that cells have also taken on some viral components to use in their vesicles. Extracellular vesicles and viruses, Margolis argues, are part of a continuum of membranous particles produced by cells. Between these two extremes are lipid-lined sacs filled with a variety of genetic material and proteins — some from hosts, some from viruses — that cells can use to send messages to one another.
“There are fundamental differences between viruses and vesicles: Viruses can replicate and vesicles cannot,” Margolis said. “But there are many variants in between. Where do viruses start, and where do extracellular vesicles start?”
Whether cells started using vesicles for communication first and viruses copied them, or cells stole the idea from viruses, or both evolved the strategy in tandem is currently impossible to determine: Sending information in extracellular vesicles must have first appeared billions of years ago because even bacteria do it. “This idea of using a membrane-bound sac of information to transport between cells has been around a long time,” said David Meckes, Jr., a virologist at Florida State University.
One of the most striking pieces of evidence supporting Margolis and Gallo’s hypothesis is the recent discovery, widely reported in January, that a mammalian protein called Arc, which is implicated in learning and memory, is actually a repurposed retroviral protein. More important, Arc appears to be secreted from the synapses of neurons in extracellular vesicles. “These vesicles may be acting like a viral envelope,” said Cedric Feschotte, a retrotransposon expert at Cornell University.
Now that humans are aware of this shared membranous medium for transporting information between cells, the idea is paving the way for new discoveries and the development of new therapeutics for cancer and viral diseases.
Bad News Wrapped in Protein Coats
When scientists first started gazing at cells under powerful light microscopes, they noticed a “dust” of minuscule particles surrounding the otherwise crisp edges of the cell’s plasma membrane. Researchers generally chalked up the debris to the cellular equivalent of dandruff and didn’t pay much attention. Over time, scientists noticed that these membranous flakes appeared in a wide range of cell cultures and body fluids, such as plasma and blood. Some formed by budding directly from the cell membrane itself and were first dubbed microvesicles, and later, extracellular vesicles. Other, typically smaller ones were assembled within the cell before being released through the plasma membrane and became known as exosomes. Extracellular vesicles and exosomes range tremendously in size, from 30 nanometers — approximately the diameter of a small virus — to as large as one micron.
The quantity of these vesicles is extraordinary: Every day, a cell produces the equivalent of its own plasma membrane in extracellular vesicles and exosomes, according to D. Michiel Pegtel, a vesicle expert at VU University Medical Center in Amsterdam.
The field took off in 2006–2007 when a Swedish team and a joint American-European group independently discovered that exosomes and extracellular vesicles could carry several types of RNA. These included the messenger RNAs (mRNAs) that are intermediaries in the translation of DNA into proteins, as well as the small molecules called microRNAs that affect gene expression. After the initial discovery of extracellular vesicles and exosomes in blood, scientists found them in nearly every type of body fluid they tested, including saliva, urine, amniotic fluid, breast milk and seminal fluid. Although researchers have begun to classify extracellular vesicles and exosomes into different subtypes, they struggle with finding ways to sort and identify those categories.
The realization that vesicles can carry RNAs also invites comparisons to viruses. Some of the vesicles that cells shed are similar in size to viruses, but their molecular cargo and their capabilities are of course different. “What inherently separates vesicles and exosomes from viruses is that exosomes are not infectious,” Pegtel said. Even so, the reasons for the similarities are significant.
The pioneering immunologist Peter Medawar once asserted that viruses are “bad news wrapped in a protein coat” — but retroviruses also drape a second layer over their protein shell by wrapping themselves in pieces of their host’s cell membrane. The host-derived membrane protects the virus from discovery by the immune system. When virologists probed the cellular pathways hijacked by these minuscule pathogens, they discovered that viruses get their envelopes by tapping into cells’ preexisting pathway for making exosomes and extracellular vesicles.
Not all the viruses encased in cell-derived envelopes are fully intact and functional. Many are the equivalent of lemons in a used car lot: secondhand and not operational. These viral trash heaps covered in membrane can’t infect other cells or perpetuate disease outbreaks. Yet in some cases, on the surface, these vesicles carrying viral junk look nearly identical to those carrying cellular RNA.
The similarity was so striking that Margolis realized that some viruses — like HIV and other small RNA viruses — and exosomes and extracellular vesicles fall on two different extremes of the same continuum. The defective viruses and viruslike particles extruded from infected cells form the vast middle ground on this field, Margolis says.
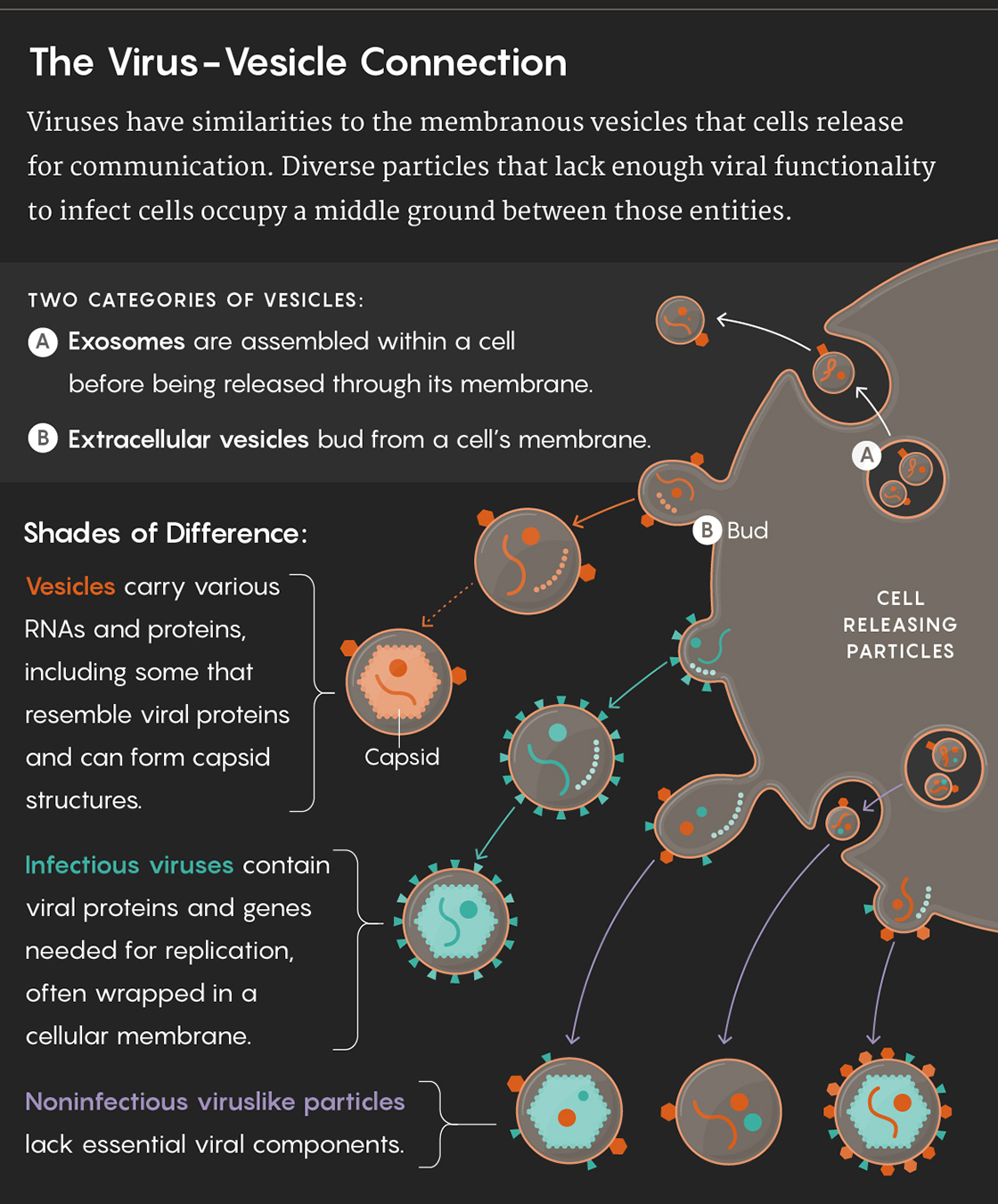
Lucy Reading-Ikkanda/Quanta Magazine
“Cell-cell communication is one of the most ancient mechanisms that makes us who we are,” Margolis said. “Since vesicles resemble viruses, the question of course is whether the first extracellular vesicles were primitive viruses and the viruses learned from extracellular vesicles or vice versa.”
Margolis and his colleagues at the NICHD and abroad weren’t the first to notice the similarities between vesicles and viruses, but their 2016 paper in the Proceedings of the National Academy of Sciences was the first to hypothesize that they were two extremes of the same phenomenon. The idea was provocative, said Dirk Dittmer, a virologist at the University of North Carolina at Chapel Hill, because people really weren’t thinking in that way. “But those are the kinds of things we like to debate late at night, and no one has an answer.”
What Margolis’s idea needed was more evidence supporting the close relationship between viruses and exosomes. This support eventually came from two independent labs that weren’t even studying this relationship.
Raw Materials for Evolution
When the neuroscientist Jason Shepherd and his postdoc Elissa Pastuzyn at the University of Utah began trying to decode the detailed structure of the Arc protein, they knew nothing about extracellular vesicles. What they did know was that mice lacking the Arc gene were unable to learn from scary situations—a deadly defect for an animal that’s a snack-size morsel for many predators. What’s more, another lab had already forged ahead with a less-detailed structure of the protein, and they were strongly motivated to publish a more detailed paper on Arc.
As Pastuzyn repeatedly tried to purify Arc, however, the single protein kept self-assembling into a more complex structure. At first, everyone thought it was a mistake. But when it kept happening, Shepherd and Pastuzyn took a peek under the electron microscope. The protein structure looked familiar.
“It looked like a virus,” she said. “It was a double-ringed structure, and the resemblance was uncanny. I had no idea that’s what it was.”
When Pastuzyn looked up the DNA sequence of Arc in GenBank (the NIH’s depository for all gene sequences), she discovered that the predicted structure of Arc most closely resembled that of Gag, a protein that forms a retrovirus’s capsid shell, which is subsequently encased in a host-derived lipid membrane.
Gag isn’t the only culprit, either. What researchers have come to realize is that sometime millions of years ago, part of a retrovirus genome inserted itself into its host’s DNA, and that sequence was then passed on to countless generations of offspring. Around 8 percent of the human genome is ultimately derived from viruses. Although some of this DNA is, in fact, “junk,” scientists are learning that much of it plays a role in our biology.
For the host, these viral genes provide a genetic junk drawer full of nuts and bolts for evolution to play with. Evolution, Shepherd says, is the ultimate MacGyver, referring to the 1980s TV hero who could defuse a bomb with bubble gum and a paper clip. It doesn’t invent things outright in an insomnia-fueled burst of creativity. Instead, evolution tinkers, cobbling together inventive solutions out of the spare parts at hand.
“Although these viruses aren’t good for individuals, they provide the raw materials for new genes,” Shepherd says. “They’re a potential gold mine.”
In the case of Arc, the Gag-derived viral gene gave mammals a ready-made delivery device that could be packaged in an extracellular vesicle. A retrovirus packages RNA and moves it out of the cell, Feschotte said. “Arc has preserved many of these same functions.”
About two thousand miles east of Shepherd’s lab in Salt Lake City, Vivian Budnik was also working on Arc in her lab at the University of Massachusetts Medical School. Unlike Shepherd, whose interest in memory and learning spurred his interest in Arc, Budnik became interested in the protein through her studies of extracellular vesicles at the synapse of neurons. In 2009, Budnik and her colleagues generated the first animal model that showed how fruit flies use extracellular vesicles to ferry a protein called Wnt across the synapse. When Budnik read a paper that showed extracellular vesicles could carry microRNA, it made her wonder if the vesicles could also carry messenger RNA. She began looking in the fly version of the Arc protein.
Then Travis Thomson arrived in her lab as a postdoc after completing another postdoc in a lab that studied the mobile genetic elements called transposons, many of which resemble viruses. As soon as he saw the mRNA from the Arc gene, he noted that it looked like RNA from a virus and wondered if it also behaved like a capsid.
Budnik presented her initial findings on Arc at a closed conference two years ago; Shepherd was sitting in the back and realized Budnik had independently reached the same conclusions about Arc. He approached her afterward and explained his identical findings from a different approach. Budnik and Shepherd soon determined that animals had repurposed a retroviral Gag protein twice: once in flies and once in mammals. In both groups of animals, Arc acts to move RNA across synapses.
“They look very similar. The mechanism on a molecular level is very similar, even though they come from different retrotransposons,” Feschotte said.
Shepherd and Budnik agreed to publish their papers in parallel, and did so in January 2018 in Cell. Budnik’s experience with Arc led her to look for other transposons and viral elements transported by extracellular vesicles. Thus far, she has found several, and one of them behaves like Arc. “We have viruslike sequences throughout our genome, but we have mostly no idea what they do,” Budnik said.
This work bolsters the close links between extracellular vesicles and viruses. Meanwhile, Shepherd and his colleagues have been scouring the human genome for other genes similar to Arc. Like Budnik, they’ve found several (their results, too, have yet to be published).
The recent explosion of research on extracellular vesicles — from 135 studies published in 2013 to 1,087 studies in 2017 — testifies to scientists’ new appreciation of their centrality to cellular functioning. Because extracellular vesicles and exosomes can pass information between cells, scientists have begun to implicate them in everything from cancer to viral infections to basic neural functioning. To Lynne Maquat, an expert on retrotransposons at the University of Rochester, this process shows how parts of the genome we used to think of as junk actually have important functions.
“You could say that the host domesticated a viral sequence for its own purposes,” Maquat said. “That’s the beauty of our complexity — [these elements] allow tinkering or fine-tuning of genes.”
Although it’s now clear that extracellular vesicles are far from simple cellular debris, and the viral genes littering our DNA aren’t exactly junk, researchers have only just begun to crack the mystery of what they can do.
Correction: The article was updated on May 4 to specify that Leonid Margolis is affiliated with the NICHD within the National Institutes of Health.
This article was reprinted on TheAtlantic.com.