‘Crazy’ Supernova Looks Like a New Kind of Star Death
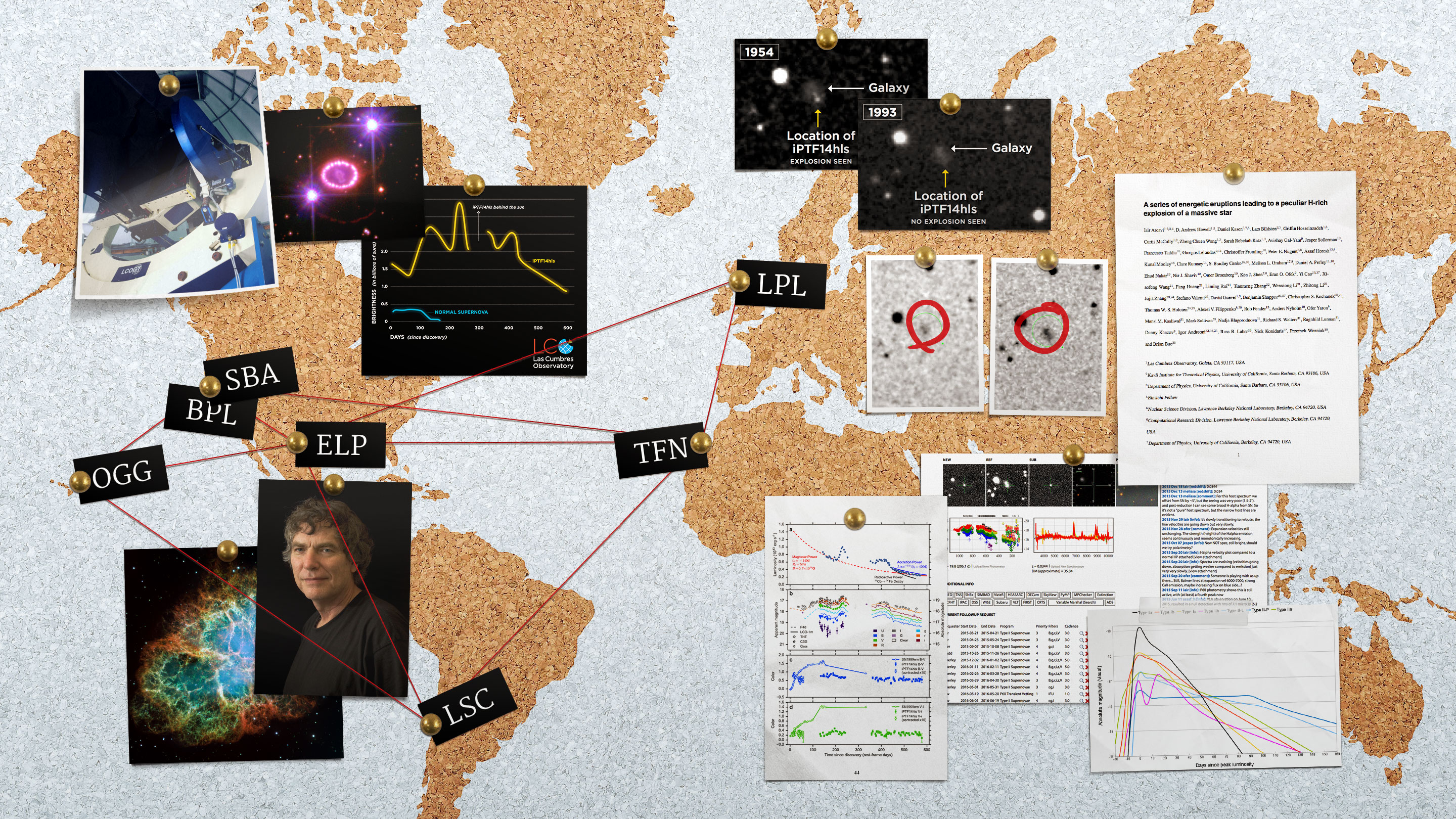
Photo illustration by Olena Shmahalo/Quanta Magazine
Introduction
In September 2014, astronomers saw a dimming point of light in a small galaxy half a billion light-years away. It looked like an ordinary supernova — a dying star that exploded and whose light was now petering out. But the following January, Zheng “Andrew” Wong, a student intern at Las Cumbres Observatory in Goleta, California, noticed that the light was getting brighter again.
When he showed this odd turnaround to his supervisor, the astronomer Iair Arcavi, “his eyes grew,” Wong recalled. Arcavi soon convinced himself that the light source, designated iPTF14hls, must not be a supernova after all but rather a nearby pulsating (or “variable”) star superimposed by coincidence on the distant galaxy. Arcavi was so sure, he said, “I would have bet my car that that was a variable star.” Still, he helped Wong take a spectrum of iPTF14hls, measuring its color to reveal its chemical makeup. To their astonishment, the spectrum came out to be exactly that of a Type II-P supernova — the most common and well-understood kind of exploding, dying, massive star. When a Type II-P supernova explodes, its brightness rises, plateaus (hence the “P”) for about 100 days and then declines until it’s over. “We had never seen such a supernova decline and go up again,” said Arcavi, who, along with 52 collaborators, reported the discovery of iPTF14hls today in the journal Nature. “That’s when we understood we had something very interesting.”
From that moment on, Las Cumbres’ global network of robotic telescopes constantly monitored iPTF14hls as it continued to brighten and dim. The scientists discovered another strange thing about the supernova: The measured speed of the exploding material, which normally decreases over time as slower stuff deeper in the supernova becomes visible, stayed mystifyingly high. “This is one crazy SN,” Arcavi typed in the comment log for iPTF14hls on May 16, 2015, more than 250 days after its discovery. “Too bad it will soon go behind the sun.”
Surely then, the scientists thought, it would be gone for good. But when September rolled around and iPTF14hls crept out from behind the sun, there was its twinkle. In fact, the light was brighter than before.
“Someone is playing with us up there,” logged Ofer Yaron, an astrophysicist at the Weizmann Institute of Science in Israel who is part of a global supernova project that includes the Las Cumbres Observatory team.
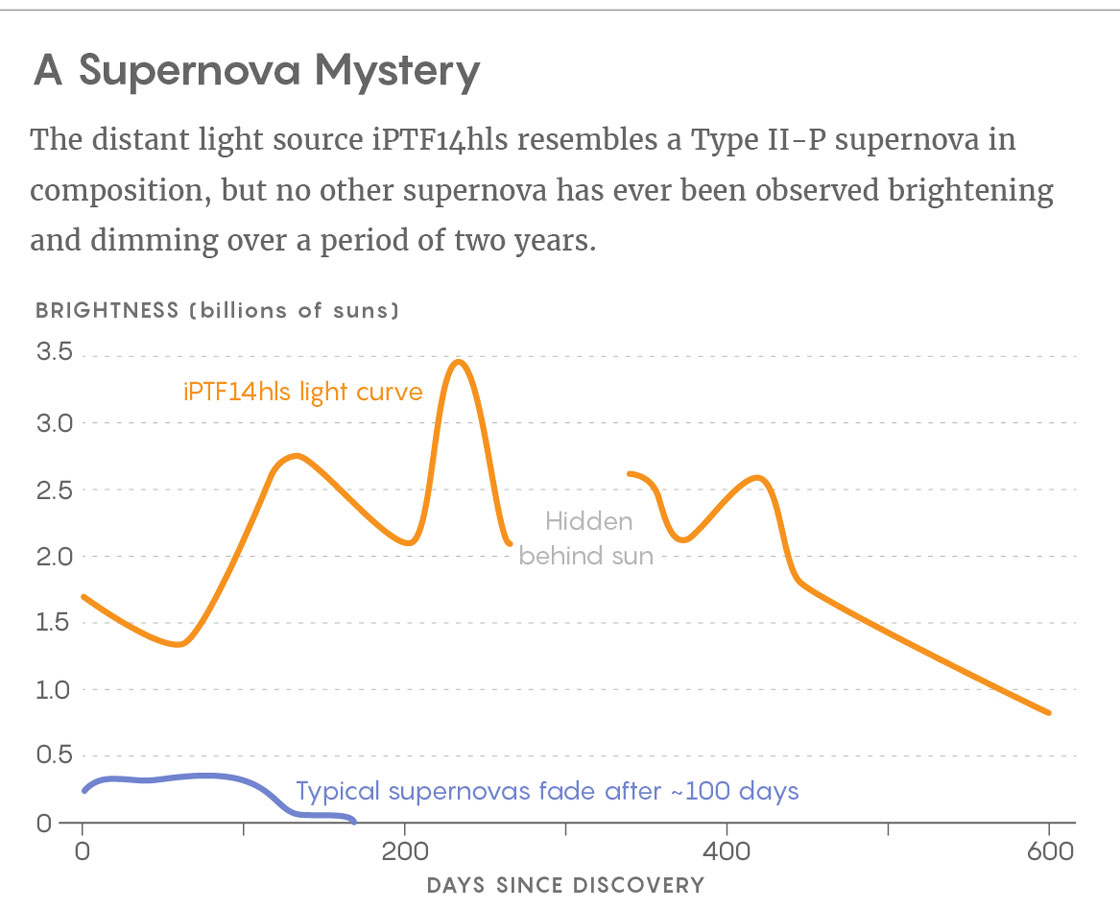
Lucy Reading-Ikkanda/Quanta Magazine; adapted from Arcavi et al. 2017
The Las Cumbres group was aiming to track 500 supernovas over three years as part of a broader mission by astronomers to develop a taxonomy of star deaths. The goal is “to synthesize some picture of what all the different stars of different masses and metallicities and rotational rates do when they die,” said Stan Woosley, a theoretical astrophysicist at the University of California, Santa Cruz. Whereas garden-variety stars like the sun blink in and out of existence relatively inconsequentially, massive stars — the kind that go supernova — are the engines of the cosmos, churning and processing matter in life and death. The explosions of these rare stars, which pack at least eight and as many as hundreds of solar masses, forge metals (what astronomers call all elements heavier than hydrogen and helium) and spew material into the surrounding galaxy, birthing the next, more metallic generation of stars. Meanwhile, their cores can collapse into black holes, super-dense neutron stars, magnetized neutron stars known as magnetars, or spinning magnetars called pulsars — but which paths arrive at which outcomes, and are there others? Knowing the full spectrum of stellar fates is crucial for understanding galactic evolution. Yet recent findings like the one from Las Cumbres suggest that stars die in more ways than anyone knew.
The researchers quietly monitored iPTF14hls for the next two years, discussing their strange sighting with a few select theorists in the hope that someone would have a clue about what they had found. Ideas emerged — the birth, during a supernova, of a magnetar giving off bright flashes called gamma ray bursts, perhaps, or a newly formed black hole swallowing the starry material around it — but nothing quite worked. “A theory could explain some stuff, but there’s a problem, and then another theory can explain this other thing, but then there’s a problem,” said Andy Howell, an astronomer at Las Cumbres Observatory and the University of California, Santa Barbara, who leads the Las Cumbres supernova team. “They don’t know a damn thing about it.”
What theorists do know is that massive stars typically go supernova when their cores run out of nuclear fuel. The drop in outward radiation pressure causes the core to gravitationally collapse (forming a black hole or neutron star), and the internal contraction creates an outgoing shockwave that thrusts the star’s outer shells of material into space, along with blinding radiation. With Type II-P supernovas, the “progenitor” star contains enough hydrogen in its outer shells to get ionized by the supernova shockwave and turn opaque. It steadily lets light out as it de-ionizes, resulting in the characteristic 100-day plateau in brightness. The hydrogen and iron detected in iPTF14hls’s spectrum exactly matched that of a II-P, and yet, Howell said, “What a II-P would look like at day 30, this weird one looks like at day 300.” Its progenitor star must have been unusually enormous, with never-before-seen levels of hydrogen, to shine for hundreds of days, but that still wouldn’t explain its mysterious brightening and dimming or the undiminishing speed of its exploding material. Whatever this was looked like a Type II-P supernova in composition but didn’t behave like one.
In September 2016, Howell presented the Las Cumbres findings at a supernova conference in Garching, Germany, to an audience that included Peter Nugent, an astronomer who helps operate the Intermediate Palomar Transient Factory sky survey that originally spotted iPTF14hls. As Nugent and the other supernova researchers absorbed the details about the incredibly long supernova, they thought of a well-known theory developed by Woosley, the Santa Cruz theorist, and others. Woosley hypothesizes that stars with initial masses in the range of 70 to 140 solar masses will die in stepwise explosions called “pulsational pair-instability supernovas” (PPISNs), due to a quantum phenomenon that acts on a gargantuan scale. Rather than undergoing core-collapse, these huge stars burn so hot that their radiation spontaneously converts into electron-positron pairs, calculations suggest. As radiation pressure is lost in the switch from light to matter, the star suddenly contracts; when the compression causes fuel in the outer shells of the star to ignite, the contraction reverses and the star explodes. It then contracts, then explodes, in a halting heave-ho.
PPISNs have never been definitively seen before, but theorists believe that they would play out in diverse ways, while always sharing one hallmark feature: The stars would erupt erratically over the course of days, months, decades, centuries or even millennia, before their cores have reduced enough in size to stop experiencing the quantum pair-instability, at which point, finally, they gravitationally collapse into black holes. (If Woosley’s theory is correct, there ought to be an absence of black holes over a certain mass range, since stars that start out in that range shrink through pulsational pair-instability explosions. Astronomers are looking for this black hole “mass gap” with the Laser Interferometer Gravitational-Wave Observatory.) In death by PPISN, each of the precursor explosions ejects many suns’ worth of mass — hiccups that create clouds of debris around the star and that are like mini supernovas themselves. As the ejected shells run into one another, the explosions appear to us as temporary brightening and dimming, as in the light signature of iPTF14hls.
In Garching, Nugent thought to check the historical record for evidence of precursor explosions from iPTF14hls’s progenitor star. He searched a vast archive of old photographic plates from the Palomar Optical Sky Survey that he knew had been digitized and put online. There was no sign of iPTF14hls in an image from Palomar’s 1993 survey, which Nugent checked first. But when he looked at an older, grainier photographic plate from the 1954 survey, amazingly, a point of light shone at its location. Supernovas are rare, and the chance that two different massive stars would explode within decades in the same small galaxy is slim. It’s difficult to estimate the statistical significance of a signal in a digitized image of a photographic plate, Nugent said. “But your eye doesn’t lie. You look at this and say, ‘OK, that looks exactly right.’”
The repeat eruptions suggest that iPTF14hls might indeed be a pulsational pair-instability supernova, the result of spontaneous quantum conversions, via Einstein’s E=mc2, from energy to matter, and back again. If so, the discovery would prove the bold PPISN hypothesis and add a major branch to the star-death taxonomy. But although Arcavi, Howell and their Las Cumbres colleagues offer the PPISN idea as the leading explanation for iPTF14hls in their paper, the theory is not without problems. “The explanation that we mention in the Nature paper doesn’t really work,” said Arcavi, referring to the current PPISN theory, “so we still don’t really know.”
For one thing, the immense energy released in the course of iPTF14hls’s known explosions (and there may have been more) already surpasses Woosley’s predictions for how much energy the pair-instability mechanism can muster. The theory also has trouble accounting for the considerable hydrogen in the strange supernova’s spectrum — earlier eruptions ought to have blown the star’s hydrogen envelope into space — not to mention the hydrogen’s bizarrely high speed, which shows no correlation with changes in brightness. (Woosley says he has “a notion” about how to explain the hydrogen measurements.)
The PPISN idea isn’t a perfect match for observations, but that might just mean that researchers need to improve the theory. Stars evolve in wildly different ways and are computationally demanding to model and predict. Often theorists can only do computer simulations of two-dimensional slices of stars and cautiously extrapolate to three dimensions. They might be underestimating the energy of PPISNs.
One piece of circumstantial evidence for the PPISN theory is iPTF14hls’s location in a “dwarf” galaxy; these consist of mostly hydrogen and helium and contain few of the heavier processed elements known as metals. The fresh raw ingredients in dwarf galaxies allow huge stars to form, like pumpkins in fertile soil. Stars can reach the mass-range required for death by pulsational pair-instability. The researchers point out that even if their super-long supernova isn’t a PPISN but something else not yet imagined, objects like it might have been more populous billions of years ago, when even galaxies like ours were hydrogen-rich and less metallic. “Probably in the early universe these were much more common, but it’s more or less extinct now,” Howell said. “So it’s a dinosaur or something.”
Now that iPTF14hls has been found and described, similar objects might start showing up. Their characteristics will gradually define a new supernova class, whether they’re PPISNs or something else. Continuous monitoring by Las Cumbres Observatory’s global telescope network has opened up a temporal view of supernovas, which could yield many more surprises.
After all, iPTF14hls is not the only odd supernova to have appeared in recent years. Astronomers have also spotted extremely bright so-called “superluminous” supernovas, whose cause and origin are also unknown. “Some of them are exceedingly luminous — 100 times brighter than ordinary supernovae,” Woosley said. “So they’re not just wrinkles on an old theme; they are beasts.”
One thousand days after its discovery, iPTF14hls is finally fading. Its light has attenuated to one-twentieth its peak brightness. But things could pick up again, the scientists said, as its shockwave expands and encounters shells of material from the star’s earlier eruptions. “The one we saw in 1954 — it could be another 20 years before it’s going to run into that shell,” Nugent said.
This article was reprinted on Wired.com and in Spanish at Investigacionyciencia.es.