Dark Matter Recipe Calls for One Part Superfluid
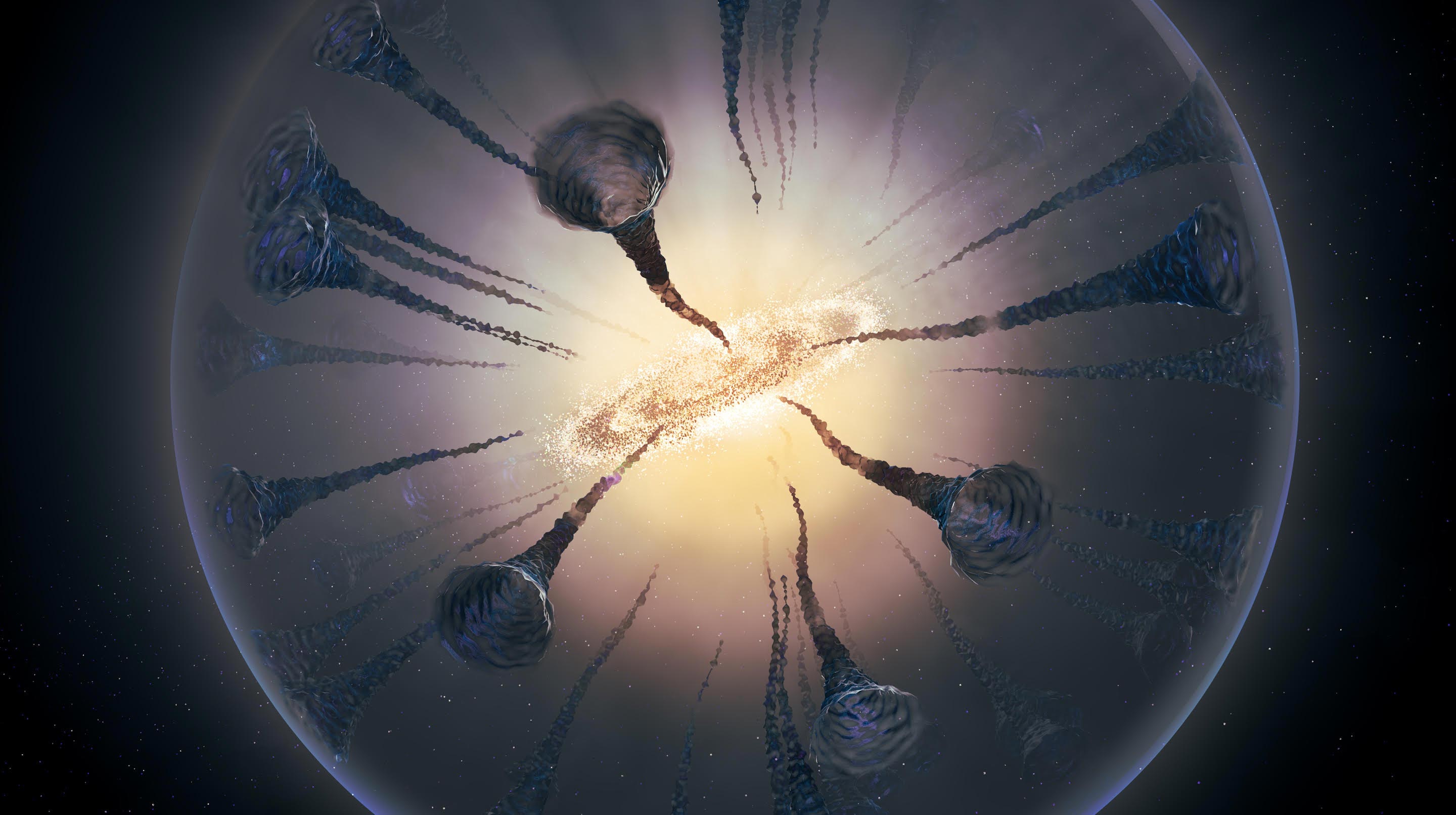
Markos Kay for Quanta Magazine
Introduction
For years, dark matter has been behaving badly. The term was first invoked nearly 80 years ago by the astronomer Fritz Zwicky, who realized that some unseen gravitational force was needed to stop individual galaxies from escaping giant galaxy clusters. Later, Vera Rubin and Kent Ford used unseen dark matter to explain why galaxies themselves don’t fly apart.
Yet even though we use the term “dark matter” to describe these two situations, it’s not clear that the same kind of stuff is at work. The simplest and most popular model holds that dark matter is made of weakly interacting particles that move about slowly under the force of gravity. This so-called “cold” dark matter accurately describes large-scale structures like galaxy clusters. However, it doesn’t do a great job at predicting the rotation curves of individual galaxies. Dark matter seems to act differently at this scale.
In the latest effort to resolve this conundrum, two physicists have proposed that dark matter is capable of changing phases at different size scales. Justin Khoury, a physicist at the University of Pennsylvania, and his former postdoc Lasha Berezhiani, who is now at Princeton University, say that in the cold, dense environment of the galactic halo, dark matter condenses into a superfluid — an exotic quantum state of matter that has zero viscosity. If dark matter forms a superfluid at the galactic scale, it could give rise to a new force that would account for the observations that don’t fit the cold dark matter model. Yet at the scale of galaxy clusters, the special conditions required for a superfluid state to form don’t exist; here, dark matter behaves like conventional cold dark matter.
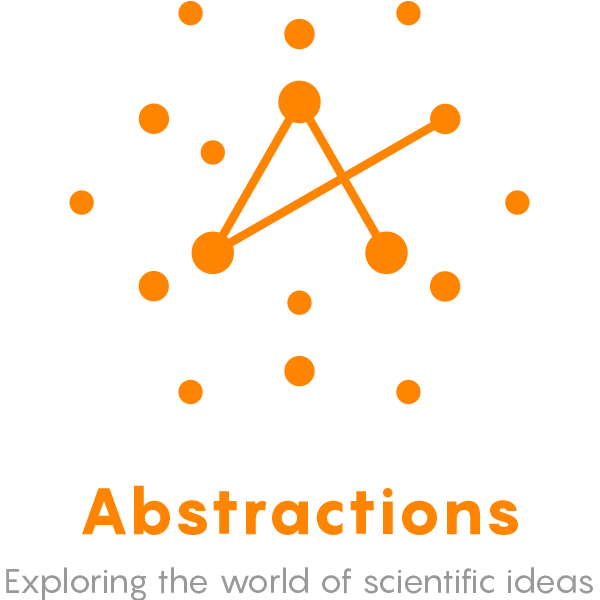
How Superfluid Dark Matter Mimics an Old Idea About Gravity
“It’s a neat idea,” said Tim Tait, a particle physicist at the University of California, Irvine. “You get to have two different kinds of dark matter described by one thing.” And that neat idea may soon be testable. Although other physicists have toyed with similar ideas, Khoury and Berezhiani are nearing the point where they can extract testable predictions that would allow astronomers to explore whether our galaxy is swimming in a superfluid sea.
Impossible Superfluids
Here on Earth, superfluids aren’t exactly commonplace. But physicists have been cooking them up in their labs since 1938. Cool down particles to sufficiently low temperatures and their quantum nature will start to emerge. Their matter waves will spread out and overlap with one another, eventually coordinating themselves to behave as if they were one big “superatom.” They will become coherent, much like the light particles in a laser all have the same energy and vibrate as one. These days even undergraduates create so-called Bose-Einstein condensates (BECs) in the lab, many of which can be classified as superfluids.
Superfluids don’t exist in the everyday world — it’s too warm for the necessary quantum effects to hold sway. Because of that, “probably ten years ago, people would have balked at this idea and just said ‘this is impossible,’” said Tait. But recently, more physicists have warmed to the possibility of superfluid phases forming naturally in the extreme conditions of space. Superfluids may exist inside neutron stars, and some researchers have speculated that space-time itself may be a superfluid. So why shouldn’t dark matter have a superfluid phase, too?
To make a superfluid out of a collection of particles, you need to do two things: Pack the particles together at very high densities and cool them down to extremely low temperatures. In the lab, physicists (or undergraduates) confine the particles in an electromagnetic trap, then zap them with lasers to remove the kinetic energy and lower the temperature to just above absolute zero.
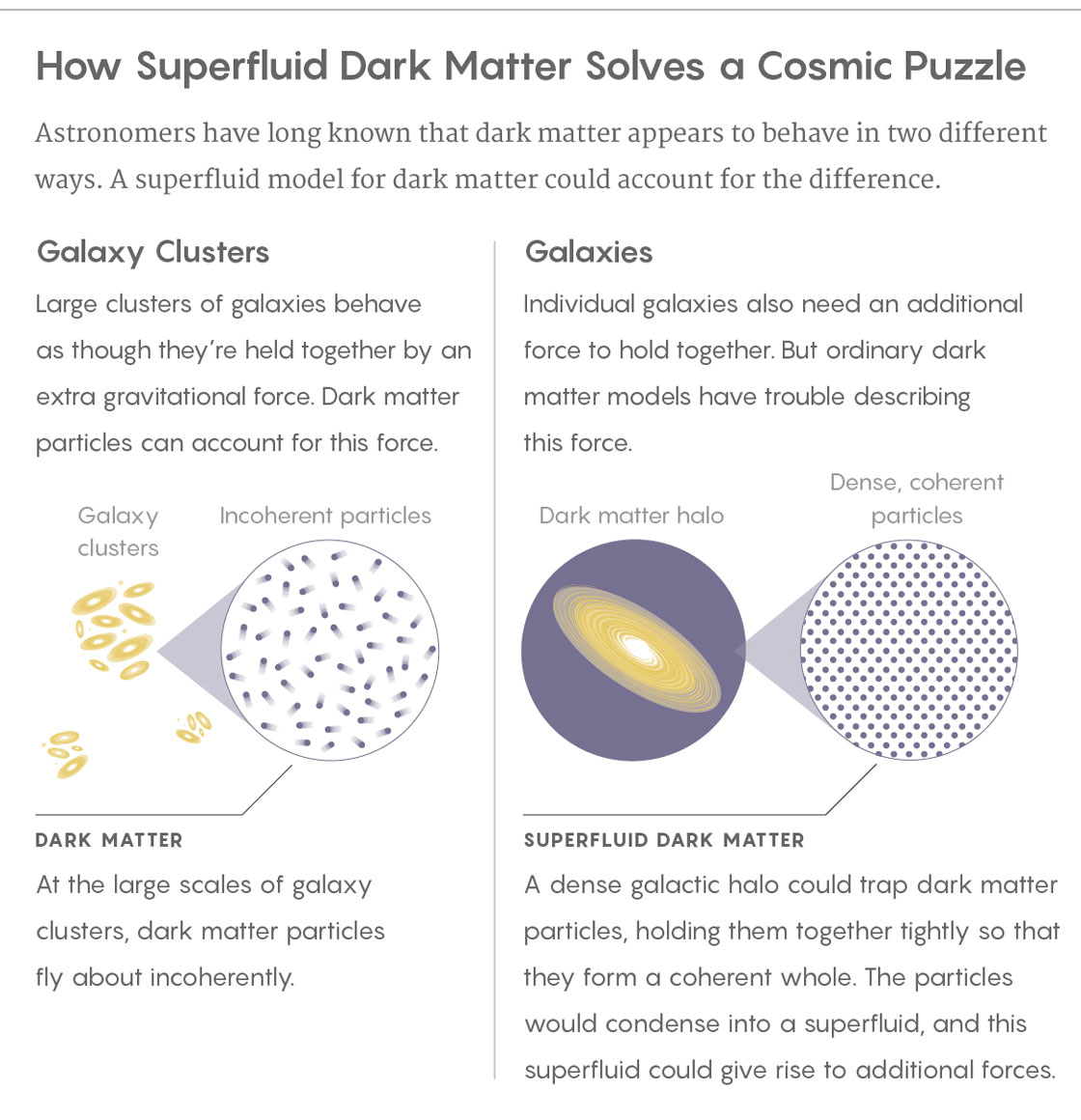
Lucy Reading-Ikkanda/Quanta Magazine
Inside galaxies, the role of the electromagnetic trap would be played by the galaxy’s gravitational pull, which could squeeze dark matter together enough to satisfy the density requirement. The temperature requirement is easier: Space, after all, is naturally cold.
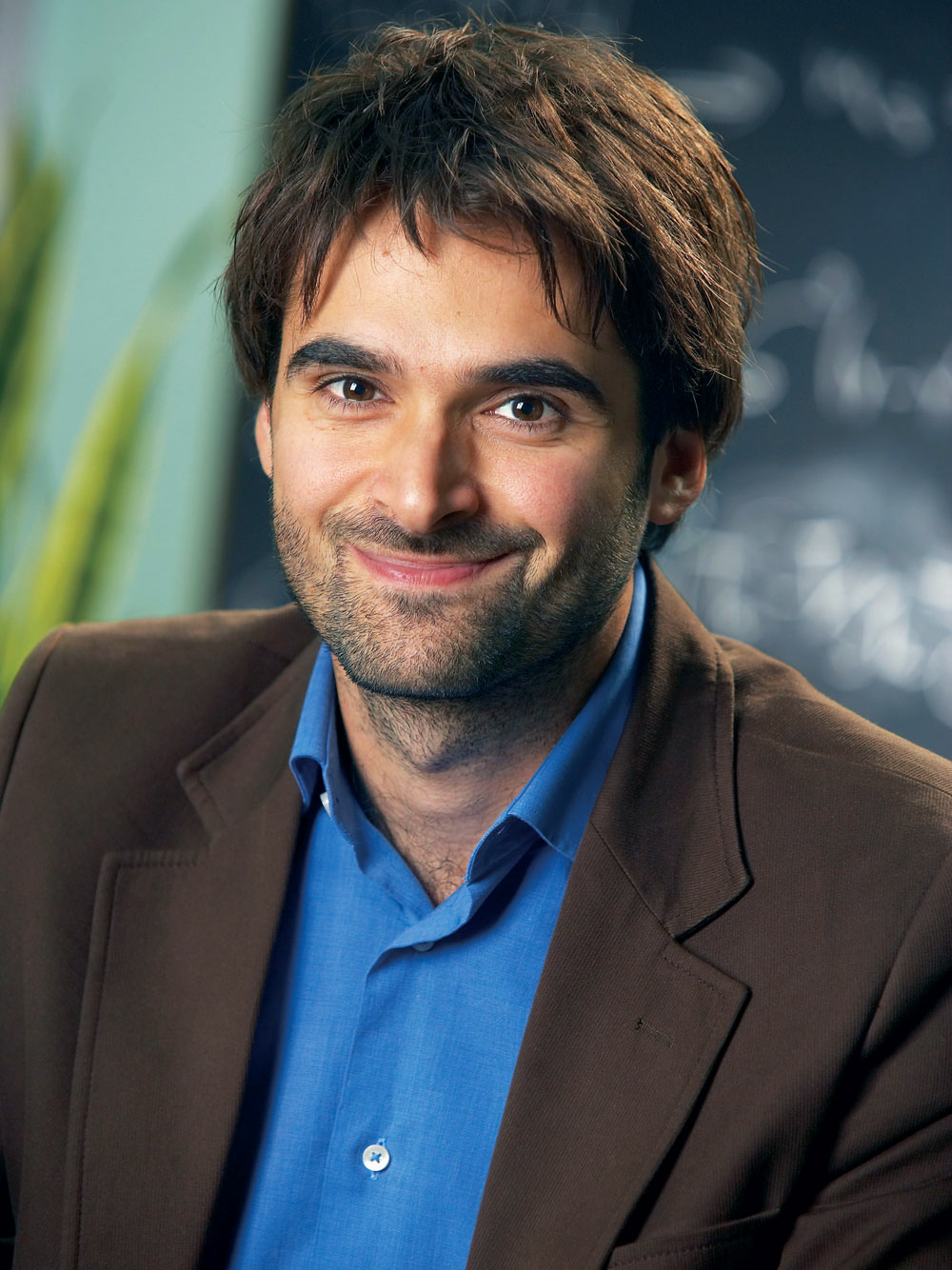
Justin Khoury, a physicist at the University of Pennsylvania, co-developed the dark matter superfluid model.
Perimeter Institute
Outside of the “halos” found in the immediate vicinity of galaxies, the pull of gravity is weaker, and dark matter wouldn’t be packed together tightly enough to go into its superfluid state. It would act as dark matter ordinarily does, explaining what astronomers see at larger scales.
But what’s so special about having dark matter be a superfluid? How can this special state change the way that dark matter appears to behave? A number of researchers over the years have played with similar ideas. But Khoury’s approach is unique because it shows how the superfluid could give rise to an extra force.
In physics, if you disturb a field, you’ll often create a wave. Shake some electrons — for instance, in an antenna — and you’ll disturb an electric field and get radio waves. Wiggle the gravitational field with two colliding black holes and you’ll create gravitational waves. Likewise, if you poke a superfluid, you’ll produce phonons — sound waves in the superfluid itself. These phonons give rise to an extra force in addition to gravity, one that’s analogous to the electrostatic force between charged particles. “It’s nice because you have an additional force on top of gravity, but it really is intrinsically linked to dark matter,” said Khoury. “It’s a property of the dark matter medium that gives rise to this force.” The extra force would be enough to explain the puzzling behavior of dark matter inside galactic halos.
A Different Dark Matter Particle
Dark matter hunters have been at work for a long time. Their efforts have focused on so-called weakly interacting massive particles, or WIMPs. WIMPs have been popular because not only would the particles account for the majority of astrophysical observations, they pop out naturally from hypothesized extensions of the Standard Model of particle physics.
Yet no one has ever seen a WIMP, and those hypothesized extensions of the Standard Model haven’t shown up in experiments either, much to physicists’ disappointment. With each new null result, the prospects dim even more, and physicists are increasingly considering other dark matter candidates. “At what point do we decide that we’ve been barking up the wrong tree?” said Stacy McGaugh, an astronomer at Case Western Reserve University.
The dark matter particles that would make Khoury and Berezhiani’s idea work are emphatically not WIMP-like. WIMPs should be pretty massive as fundamental particles go — about as massive as 100 protons, give or take. For Khoury’s scenario to work, the dark matter particle would have to be a billion times less massive. Consequently, there should be billions of times as many of them zipping through the universe — enough to account for the observed effects of dark matter and to achieve the dense packing required for a superfluid to form. In addition, ordinary WIMPs don’t interact with one another. Dark matter superfluid particles would require strongly interacting particles.
The closest candidate is the axion, a hypothetical ultralight particle with a mass that could be 10,000 trillion trillion times as small as the mass of the electron. According to Chanda Prescod-Weinstein, a theoretical physicist at the University of Washington, axions could theoretically condense into something like a Bose-Einstein condensate.
But the standard axion doesn’t quite fit Khoury and Berezhiani’s needs. In their model, particles would need to experience a strong, repulsive interaction with one another. Typical axion models have interactions that are both weak and attractive. That said, “I think everyone thinks that dark matter probably does interact with itself at some level,” said Tait. It’s just a matter of determining whether that interaction is weak or strong.
Cosmic Superfluid Searches
The next step for Khoury and Berezhiani is to figure out how to test their model — to find a telltale signature that could distinguish this superfluid concept from ordinary cold dark matter. One possibility: dark matter vortices. In the lab, rotating superfluids give rise to swirling vortices that keep going without ever losing energy. Superfluid dark matter halos in a galaxy should rotate sufficiently fast to also produce arrays of vortices. If the vortices were massive enough, it would be possible to detect them directly.
Unfortunately, this is unlikely to be the case: Khoury’s most recent computer simulations suggest that vortices in the dark matter superfluid would be “pretty flimsy,” he said, and unlikely to offer researchers clear-cut evidence that they exist. He speculates it might be possible to exploit the phenomenon of gravitational lensing to see if there are any scattering effects, similar to how a crystal will scatter X-ray light that passes through it.
Astronomers could also search for indirect evidence that dark matter behaves like a superfluid. Here, they’d look to galactic mergers.
The rate that galaxies collide with one another is influenced by something called dynamical friction. Imagine a massive body passing through a sea of particles. Many of the small particles will get pulled along by the massive body. And since the total momentum of the system can’t change, the massive body must slow down a bit to compensate.
That’s what happens when two galaxies start to merge. If they get sufficiently close, their dark matter halos will start to pass through each other, and the rearrangement of the independently moving particles will give rise to dynamical friction, pulling the halos even closer. The effect helps galaxies to merge, and works to increase the rate of galactic mergers across the universe.
But if the dark matter halo is in a superfluid phase, the particles move in sync. There would be no friction pulling the galaxies together, so it would be more difficult for them to merge. This should leave behind a telltale pattern: rippling interference patterns in how matter is distributed in the galaxies.
Perfectly Reasonable Miracles
While McGaugh is mostly positive about the notion of superfluid dark matter, he confesses to a niggling worry that in trying so hard to combine the best of both worlds, physicists might be creating what he terms a “Tycho Brahe solution.” The 16th-century Danish astronomer invented a hybrid cosmology in which the Earth was at the center of the universe but all the other planets orbited the sun. It attempted to split the difference between the ancient Ptolemaic system and the Copernican cosmology that would eventually replace it. “I worry a little that these kinds of efforts are in that vein, that maybe we’re missing something more fundamental,” said McGaugh. “But I still think we have to explore these ideas.”
Tait admires this new superfluid model intellectually, but he would like to see the theory fleshed out more at the microscopic level, to a point where “we can really calculate everything and show why it all works out the way it’s supposed to. At some level, what we’re doing now is invoking a few miracles” in order to get everything to fit into place, he said. “Maybe they’re perfectly reasonable miracles, but I’m not fully convinced yet.”
One potential sticking point is that Khoury and Berezhiani’s concept requires a very specific kind of particle that acts like a superfluid in just the right regime, because the kind of extra force produced in their model depends upon the specific properties of the superfluid. They are on the hunt for an existing superfluid — one created in the lab — with those desired properties. “If you could find such a system in nature, it would be amazing,” said Khoury, since this would essentially provide a useful analog for further exploration. “You could in principle simulate the properties of galaxies using cold atoms in the lab to mimic how superfluid dark matter behaves.”
While researchers have been playing with superfluids for many decades, particle physicists are only just beginning to appreciate the usefulness of some of the ideas coming from subjects like condensed matter physics. Combining tools from those disciplines and applying them to gravitational physics might just resolve the longstanding dispute on dark matter — and who knows what other breakthroughs might await?
“Do I need superfluid models? Physics isn’t really about what I need,” said Prescod-Weinstein. “It’s about what the universe may be doing. It may be naturally forming Bose-Einstein condensates, just like masers naturally form in the Orion nebula. Do I need lasers in space? No, but they’re pretty cool.”
This article was reprinted on Wired.com.