Deep Beneath Earth’s Surface, Clues to Life’s Origins
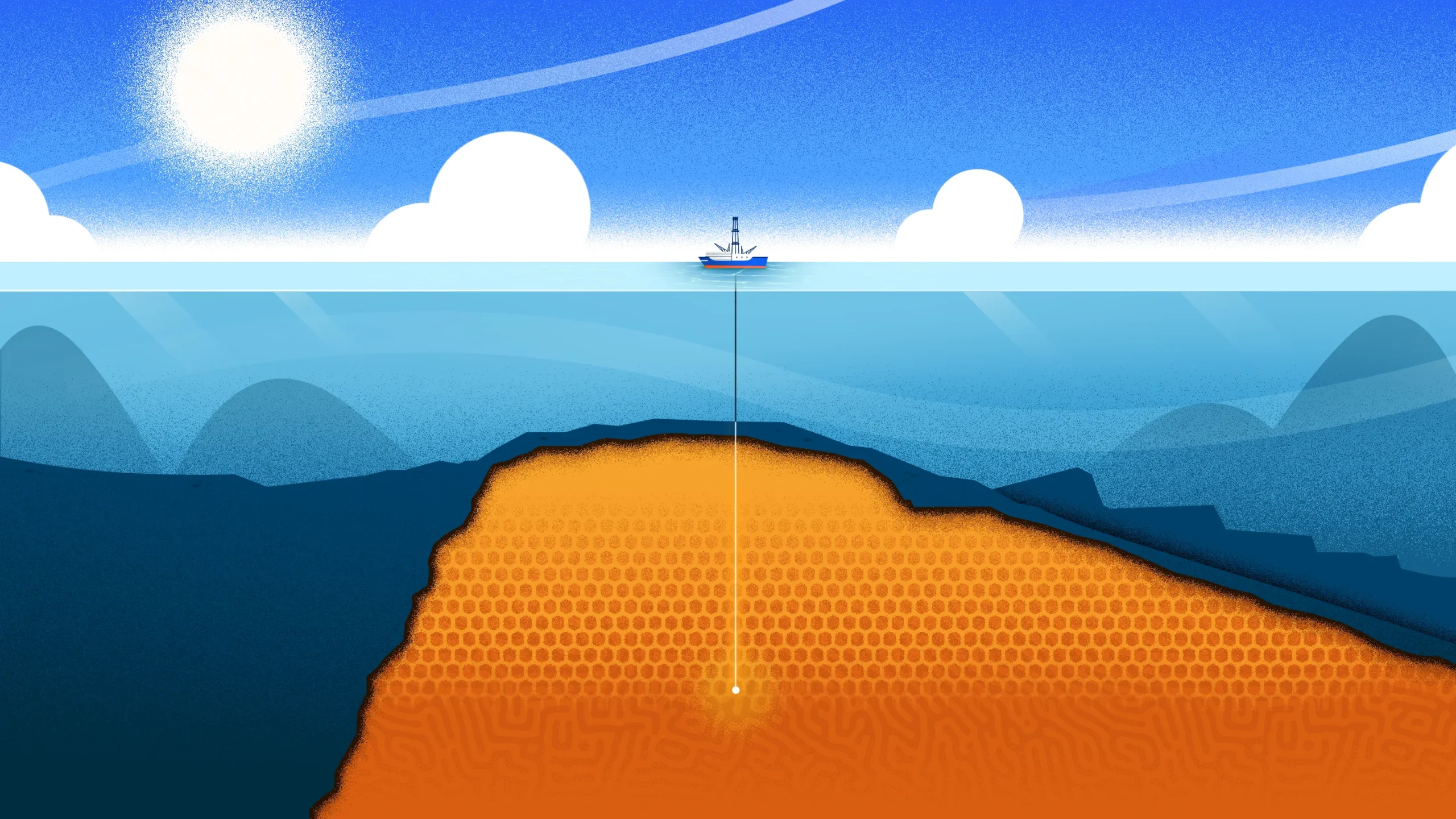
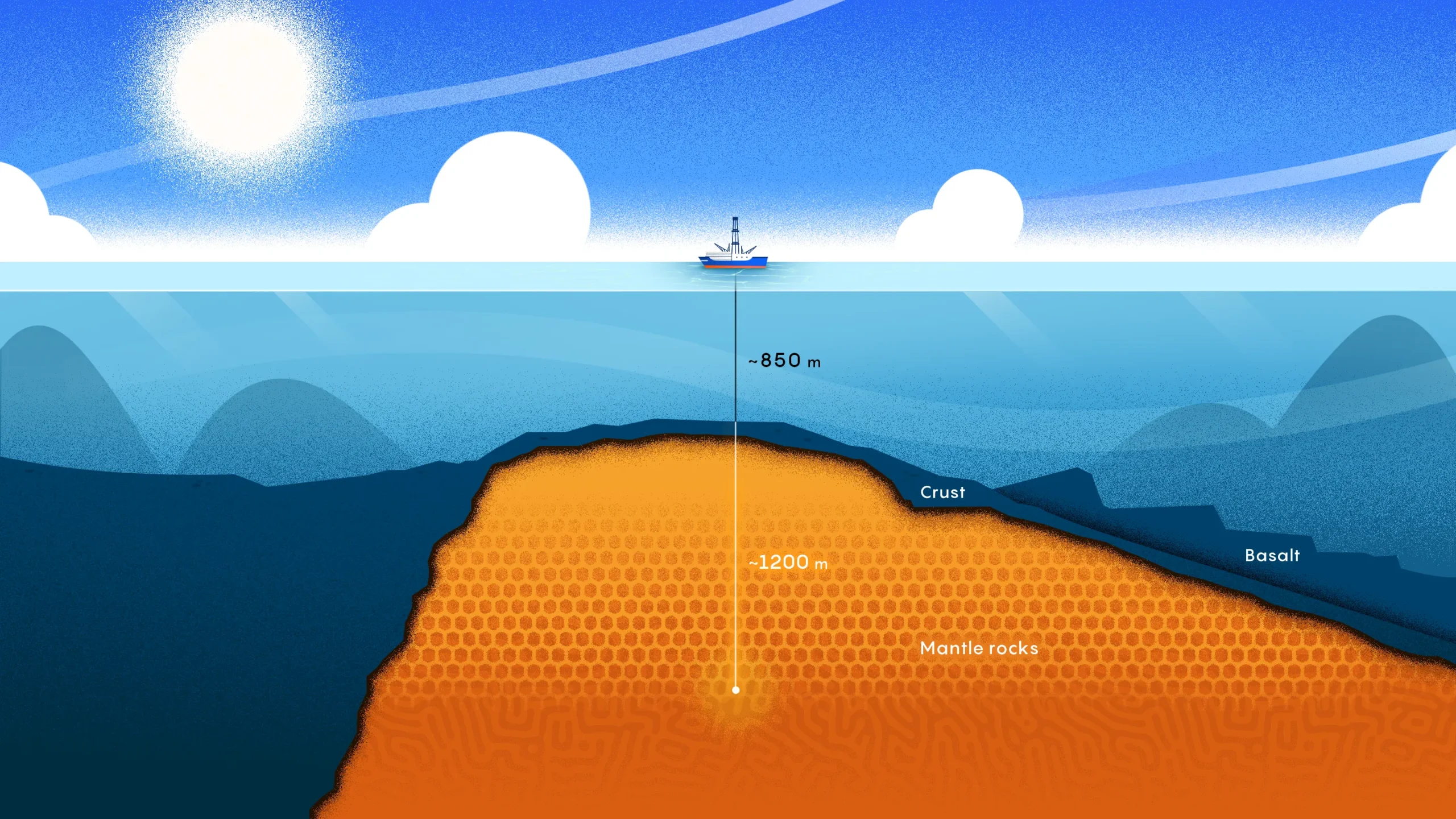
By studying the chemistry in Earth’s subterranean environments — such as the rocks deep beneath the ocean floor — scientists hope to learn what may have powered the earliest life forms on this planet.
Mark Belan for Quanta Magazine
Introduction
Near midnight on March 26, 1961, dark waters lapped at the hull of a converted naval barge as it queasily rocked in the Pacific Ocean. The ship had just arrived at this spot, some 240 kilometers off the Baja peninsula, after three days of fighting seas so rough the crew had lashed gear to the deck with heavy chains, “like a rogue elephant,” the novelist John Steinbeck, who was aboard the vessel, later wrote for Life magazine.
Back on shore, rumors flew about the crew’s goals. Some speculated that they were hunting for diamonds or sunken treasure. Others suspected that they were scouting for a place to stash a missile on the seafloor. But the team’s objectives were even loftier than the wildest hearsay. The plan — hatched over an alcohol-infused breakfast at the La Jolla home of the geologist Walter Munk — was to drill a hole so deep it would punch through Earth’s crust and reach the planet’s mantle, a hot, rocky layer sandwiched between Earth’s crust and its core.
Now, more than 62 years after the effort known as Project Mohole, scientists still have yet to successfully drill through an intact section of Earth’s crust. But this past spring, a team aboard the decades-old drillship the JOIDES Resolution accomplished the next-best thing: They retrieved a trove of mantle rocks from an area of the Atlantic seafloor where the crust is especially thin. The site is atop a submarine mountain known as Atlantis Massif, where the slow shifts of tectonic plates have shoved blocks of mantle rocks closer to the surface.
While the mantle makes up the bulk of our planet, its rocks are usually buried kilometers beneath the surface, making fresh samples tough to retrieve. But mantle rocks such as those excavated last spring could offer clues to the deep workings of Earth and help researchers better understand the tectonic choreography that’s fundamental to our world.
The newly collected rocks may also hold clues to another defining feature of our planet — life.
When seawater meets mantle rock, a series of chemical reactions generates a cocktail that can create the organic compounds needed to ignite life’s first sparks. Scientists have already found hints of small organic molecules created without microbial help at the Lost City hydrothermal vent system, a sprawling geologic metropolis atop Atlantis Massif. Some scientists have long speculated that such environments could have incubated our planet’s earliest life forms. Now, the team’s recently drilled hole, which bored more than a kilometer below the seafloor, has reached into what seems to be the beating heart of this hydrothermal system.
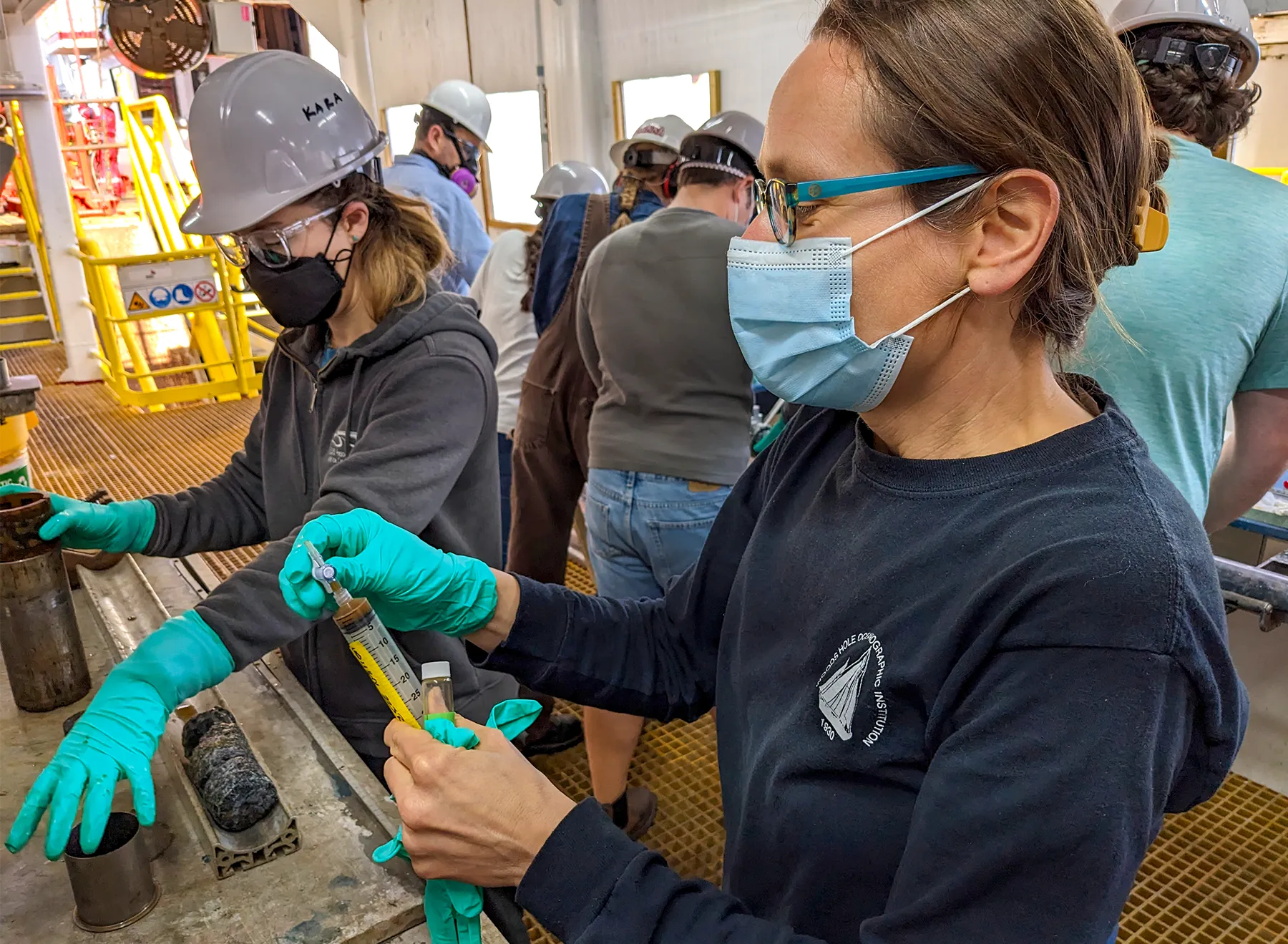
Last spring, Susan Lang (at right) co-led an expedition that retrieved a trove of mantle rocks from beneath the seafloor.
William Brazelton/University of Utah
“That opens a world of possibilities to us,” said Susan Lang, a biogeochemist at the Woods Hole Oceanographic Institution who co-led the expedition.
Already, there are hints that high concentrations of hydrogen gas in the borehole waters might be available to power organic synthesis. This natural laboratory promises to help the team untangle the origin of the life-giving stew that trickles up through Lost City’s towers, allowing them to study the organic chemistry of a world without organisms — the chemistry of life before life existed, or when life was extremely scarce. The few microbes that survive the extreme subsurface conditions may also offer clues to how the earliest beings made a living, ultimately helping scientists decipher the pivotal steps that turned chemical compounds into creatures.
Building a Lost City
Lang still remembers the day, about two decades ago, when she was offered a berth on the vessel conducting the first detailed study of the Lost City vents. Tears of excitement flooded her eyes. “I said yes without checking with anyone,” said Lang, who was a graduate student at the University of Washington at the time.
Her fervor reflected the revolutionary nature of Lost City, whose shimmery, translucent columns of hot water were first spotted by scientists aboard the research vessel Atlantis in 2000. At the time, all the other known hydrothermal vent systems were dark, with chimneys blackened by volcanic sulfides pumping thick, smoky plumes of searing fluids into the ocean. But the spires of Lost City were a ghostly white.
As scientists soon learned, the light hue stems from reactions between seawater and the rock tucked within Atlantis Massif. A bit taller than Mount Rainier, this submarine mountain is largely made of peridotite, a type of rock that dominates the upper mantle. The mountain formed from the sedate shifts of the nearby mid-Atlantic ridge, where the North American and African tectonic plates slowly pull apart. This movement stripped the upper crust from the rising peak, exposing swaths of its peridotite core.
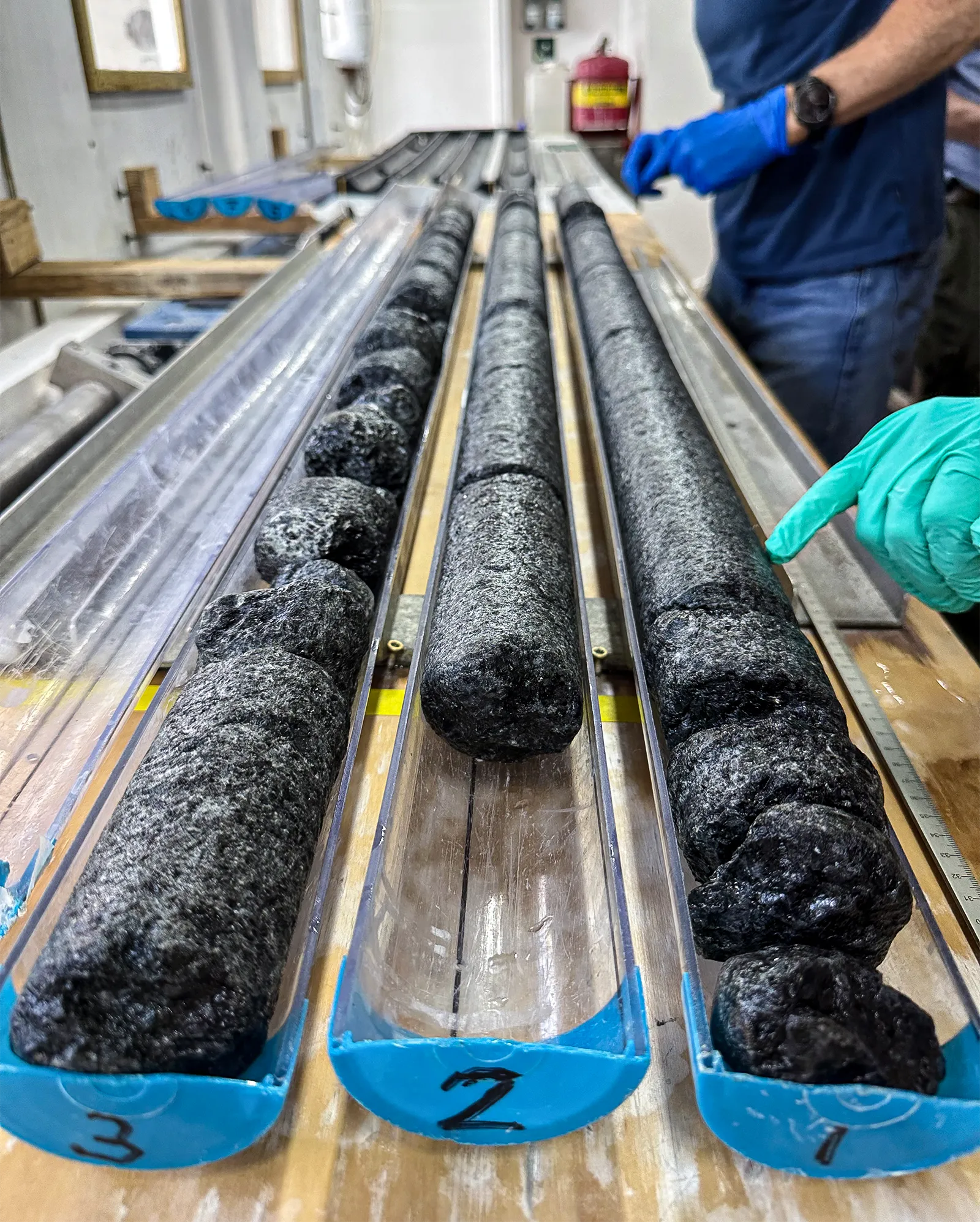
Sandwiched between Earth’s crust and its core, the mantle is normally very hard to access. But this past spring, scientists drilled into a place on the seafloor where mantle rocks sit close to the surface.
Lesley Anderson/IODP
Peridotite normally lingers under miles of crust. It’s unstable so near to Earth’s surface, where seawater can creep into cracks within the rocks. When that happens, a mineral called olivine that dominates the peridotite readily reacts with water molecules, sparking a series of chemical steps called serpentinization. The process makes the water highly alkaline, so when fluids from the crevice mingle with fresh seawater, pale minerals precipitate and build Lost City’s stunning spires, which stand as tall as a 20-story building.
But another byproduct of serpentinization, hydrogen, has drawn Lang and other scientists to the site for decades. Under the right conditions, hydrogen gas can fuel simple chemical reactions, such as turning carbon dioxide and water into small organic compounds, without microbial help (or abiotically). Continued reactions could create larger and more complex organic molecules, perhaps crafting just the right mix of ingredients — sugars, fats, amino acids — to cook up the earliest life forms. Plus, hydrogen and small organics may have also provided food for Earth’s earliest inhabitants. “Hydrogen is like the key to everything,” Lang said.
This gas was likely more common on early Earth, when the mineral makeup of the surface differed from that of today, making serpentinization reactions more common.
At Atlantis Massif, Lang and her colleagues want to know which organic compounds can form without microbial help and which microbes might survive on this unusual underground buffet. The results could offer clues about how the earliest life forms made a living, as well as the chemistry that preceded those ancient microbes.
But today life abounds on Earth’s surface, both above and below water, making it tough to identify compounds that were made without biology’s help. That’s especially true at Lost City. “You can just see the snotty biofilms growing all over those chimneys,” said William Brazelton, a microbiologist at the University of Utah and a JOIDES team member.
So researchers set their sights on the realms below the seafloor, where microbes are sparse and oxygen is scarce, creating conditions similar to those of early Earth. As Brazelton said, “We need to literally go deeper.”
Finding a Natural Laboratory
In the 1960s, Project Mohole marked the start of efforts to plumb the unexplored depths of our planet during a time of “heroic science,” said Damon Teagle, a geochemist at the University of Southampton and a veteran of many scientific ocean drilling expeditions.
The name was a play on the Mohorovičić discontinuity, or Moho, which defines the boundary between the crust and mantle. Under continents, the Moho can be found more than 30 kilometers deep; under the seafloor, it’s closer to 7 kilometers. Because of this, teams targeting the mantle usually choose to drill from ships.
Project Mohole didn’t even get close to its goal, only boring through 179 meters of sediments and a mere 4 meters of seafloor rock. Yet even that effort revealed a wealth of information about our planet, including the fact that hiding under seafloor sediments were relatively young volcanic rocks — a find that would later serve as a key piece of evidence in the case for plate tectonics. It also produced technologies that evolved into systems scientists still use, including some on board the JOIDES Resolution this past spring.
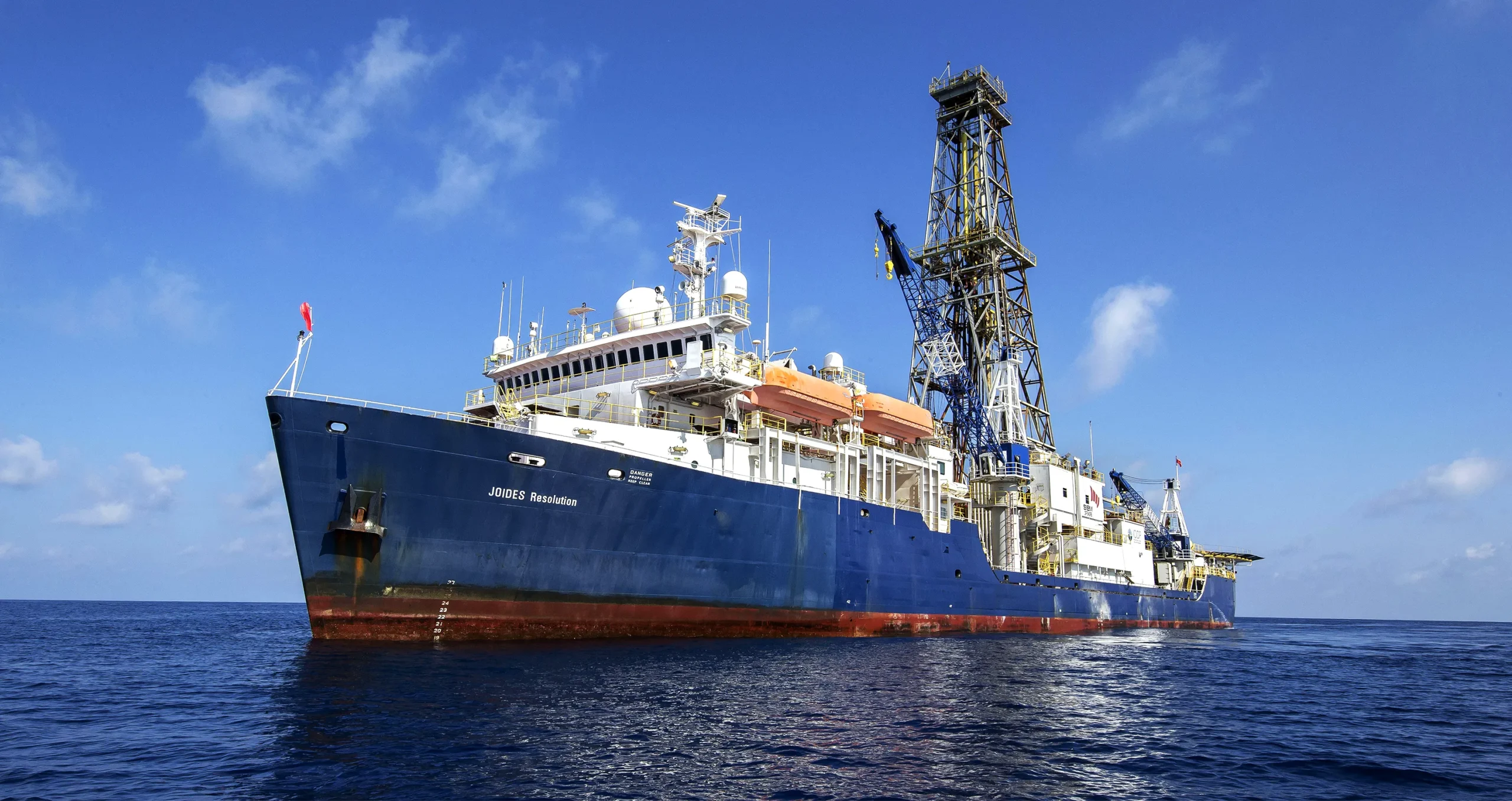
For decades, the JOIDES Resolution has helped scientists study Earth’s oceans — and what lies beneath the seafloor.
Tim Fulton/IODP/JRSO
Even today, though, deep-ocean drilling is tremendously challenging. For one thing, drilling through hard rock rapidly wears out drill bits, forcing regular bit changes and the need to reenter the same tiny borehole from a ship bobbing atop hundreds or thousands of meters of water, which is like dropping a needle into a pinhole. To make matters worse, last spring’s expedition had an inauspicious start. While the team was drilling their first pilot hole, their drill bit got stuck, and to prevent the ship from being forever anchored to Atlantis Massif, the crew severed the connection with a blast of dynamite. Then part of the system that allowed the drill to reenter a borehole multiple times broke into pieces.
With a bit of creativity, they finally got to drilling at a site now known as U1601C, which sits under nearly 850 meters of water. And that’s when their luck changed.
On most seafloor drilling expeditions, progress is slow, with rocky cores hauled on deck every three hours or so. But once the JOIDES team got going, they were heaving fresh cores on board almost every hour. Scientists processing the cores could barely keep up, and before they knew it, the drill bit had struck mantle rocks.
Before this expedition, the furthest anyone had ever drilled into altered mantle rocks was 200 meters. But the JOIDES team covered that distance in just a few days, ultimately boring through 1,267.8 meters of mostly peridotite. “It was just remarkable,” said Teagle, who was not part of the recent undertaking.
For Lang, one of the biggest surprises was hidden deep in the borehole. After removing their last core, the crew flushed the empty hole with clean water and let natural fluids and gases creep back in over 72 hours. Then they collected the borehole water at various depths and split it up for more than a dozen chemical tests, including a hydrogen gas analysis.
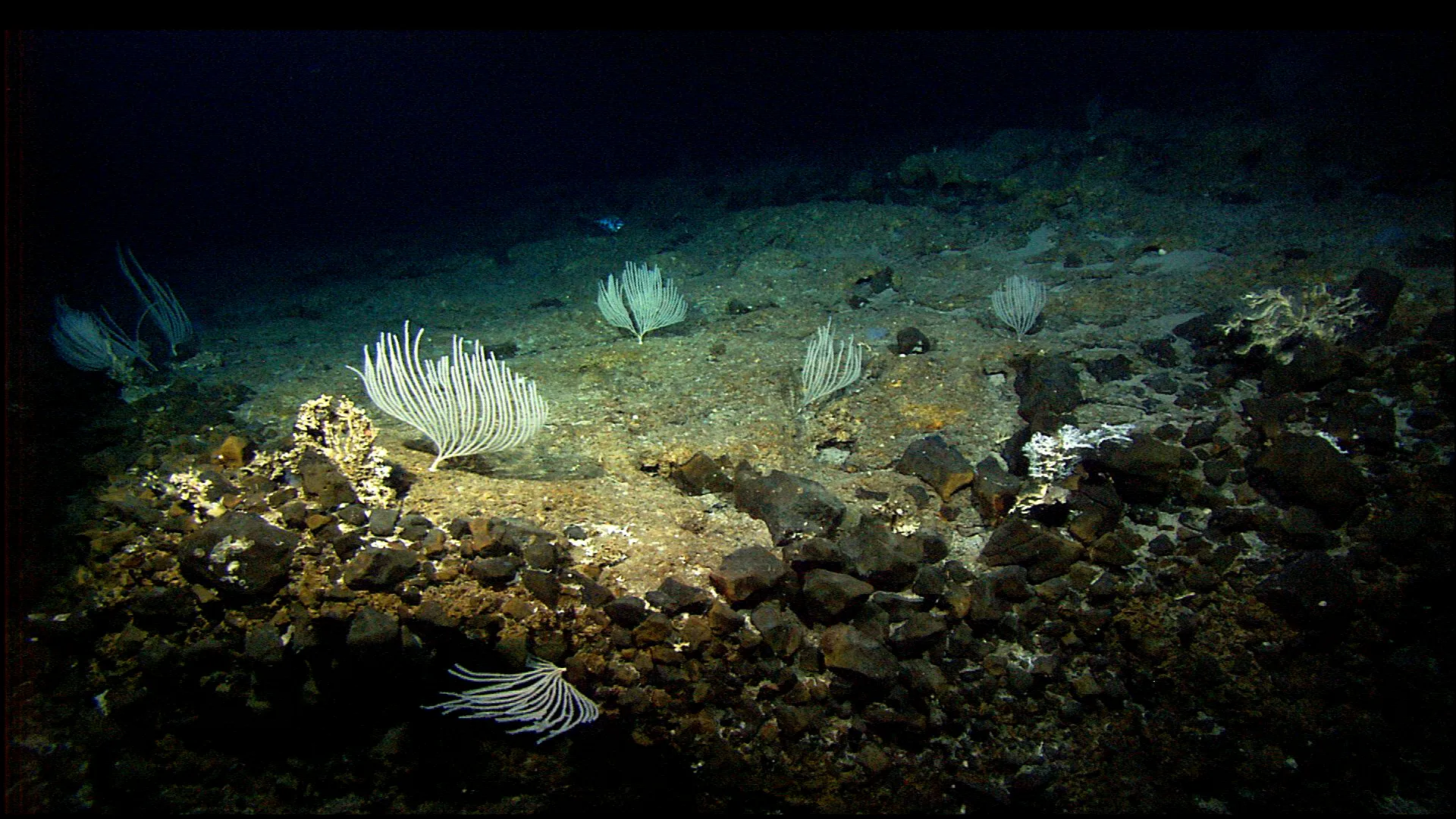
Situated on the seafloor near the mid-Atlantic ridge, Atlantis Massif offers relatively easy access to rocks with a mix of minerals thought to form in Earth’s mantle.
IFE, URI-IAO, UW, Lost City Science Party; NOAA/OAR/OER; The Lost City 2005 Expedition
At most, Lang expected to find trace amounts of hydrogen so far underground. But the deepest water sample contained so much gas that as it surfaced, bubbles formed in the tube, a phenomenon similar to what happens when you crack open a fresh can of soda.
“We were like, holy crap,” Lang said, recalling her own reaction and Brazelton’s. “There was a lot of swearing involved.”
The waters are chock-full of hydrogen, the fuel needed to power abiotic reactions.
The Building Blocks of Building Blocks
More than six months after the expedition, the team is still processing their enormous number of samples — studying the water chemistry, identifying microbes, characterizing the rocks, and more. “People are going to do a whole alphabet soup of elemental analyses on these rocks,” said Andrew McCaig, a geologist at the University of Leeds who co-led the expedition.
Preliminary models hint that temperatures near the bottom of the borehole might even reach 122 degrees Celsius, the currently known limit for life (though some studies suggest that the limit might sit even higher). Lang cautions that the models require confirmation because they’re based on measurements taken when the borehole temperatures were slightly suppressed by the cool waters circulating during drilling. If conditions are confirmed to be this extreme, though, the depth would allow scientists to study life-fueling chemical reactions without the muddling influence of microbes.
This would be a significant step forward for scientists studying life’s watery origins. “On Earth today, it’s really hard to witness abiotic or prebiotic chemistry because life dominates; life is everywhere,” said Laurie Barge, an astrobiologist at NASA’s Jet Propulsion Laboratory who was not part of the expedition.
Early analyses also suggest that the small organic acid formate is present in the borehole water. Formate is one of the simplest compounds that can form abiotically, from reactions between carbon dioxide and hydrogen, and it may mark an initial step toward the first glimmers of life on early Earth.
“It’s the raw material to build the building blocks,” Lang said. Continued abiotic reactions with formate could produce larger organic compounds like amino acids, which can be strung together into molecules essential for life, such as enzymes and other proteins.
But much of the chemical picture remains fuzzy at Atlantis Massif. The formate deep in the borehole may have formed without microbial help, as it has in the shallower subsurface nearby, but more testing is needed to be sure. The water also contains methane, a compound that some scientists think was vital for early metabolisms and one that could be generated abiotically from reactions with hydrogen. But how methane forms at Lost City is another mystery — it’s “complicated and confusing,” Brazelton said.
Identifying abiotic reactions in nature could inform future lab experiments testing prebiotic chemistry, where researchers can tweak the conditions to more closely simulate early Earth or other worlds, Barge explained. “Lost City is a really special place,” she said.
Hunting for Microbes
Even if the deep borehole isn’t devoid of life, the almost unprecedented quantity of recovered rocky cores will help scientists link shifts in water chemistry and rock types to the few microbes that may eke out a living underground. Studying how microbes survive amid scarce subsurface resources — perhaps by eating hydrogen and other abiotically formed compounds — could help sharpen our picture of early life.
Brazelton in particular is on the hunt for the specific enzymes microbes use to turn hydrogen and small organic compounds into energy. “The whole idea here is that you have chemistry going on in rocks, and at some point, that chemistry turns into life,” Brazelton said. Those enzymes might just be the knob that helps researchers rewind the evolutionary clock to decipher how the earliest metabolisms came to be.
Other efforts are focused on incubating samples from the rock and trying to catch deep microbes in action, explained Fengping Wang, the geomicrobiologist leading this work at Shanghai Jiao Tong University. Wang has been studying life in the subsurface for almost two decades, but she and other deep-biosphere researchers have largely searched for microbes hiding in ocean sediments. “We know very little about the rock microbes,” she said. “It’s one of the last questions in the deep biosphere: What’s in the hard rocks?”
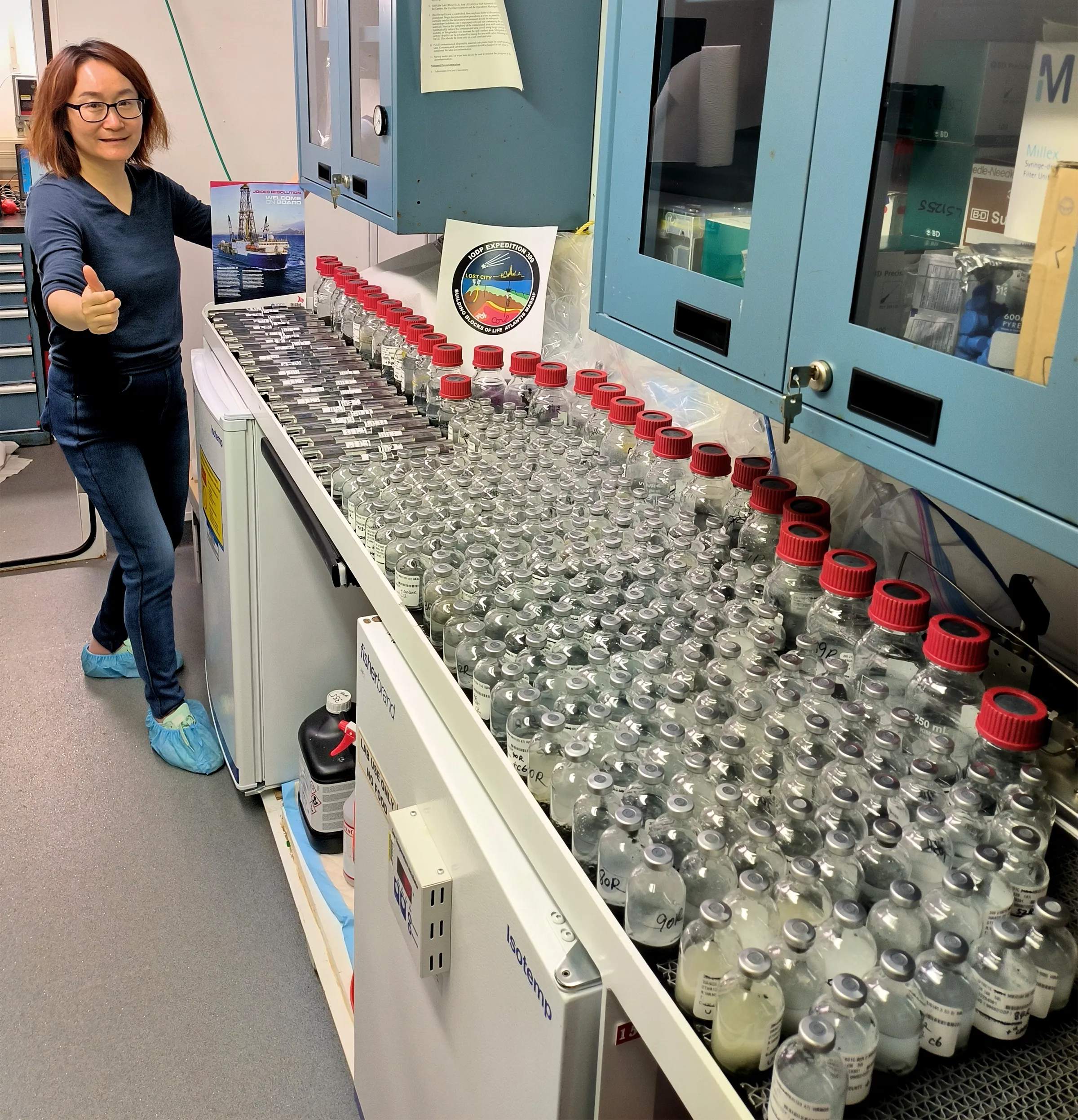
Fengping Wang, a geomicrobiologist, processed some 800 samples aboard the JOIDES Resolution. She wants to know which microbes, if any, can live deep beneath the seafloor.
Courtesy of Fengping Wang
In search of answers, Wang pulverized hundreds of core samples aboard the ship, placing each one into a metal reactor tube or glass bottle. She spiked the samples with a variety of foods — a microbial tasting menu suited for an unknown diversity of diets. And then she incubated the samples at different temperatures to see what would grow.
Overall, she set up nearly 800 incubations and posed for a picture with them in the shipboard lab “to show my hard work,” she said with a chuckle. In the picture, every inch of the table in front of her is packed with glass bottles, which are just a fraction of her total samples.
Wang’s preliminary results reveal an excess of methane in some samples, but whether the gas comes from burping microbes or reacting rocks is not yet clear.
Scientists across many fields are eagerly awaiting the team’s findings. “We’re definitely going to have a much better view of … what actual chemical processes are taking place,” said Yoshinori Miyazaki, a geophysicist at the California Institute of Technology.
The excitement and triumph surrounding the latest work, however, is also tinged with sadness. This expedition is one of the last for the JOIDES Resolution, which will retire at the end of 2024 after four decades of groundbreaking research in ocean waters around the world. There’s currently no concrete plan to replace the ship, which leaves a gaping hole in ocean research for U.S. scientists.
Over its lengthy tenure, expeditions on board the JOIDES Resolution have recovered more than 350 kilometers of cores from the seafloor. Hidden within this geologic trove are many secrets of our planet’s past — shifts in climate, ocean chemistry, and perhaps other clues to life’s origins. But even more information is still locked in the rocks of the seafloor, just waiting to be uncovered.
Correction: January 5, 2024
The original version of this article included an image of an undersea vent that was misidentified as being part of the Lost City. It has been removed.