Famous Experiment Dooms Alternative to Quantum Weirdness
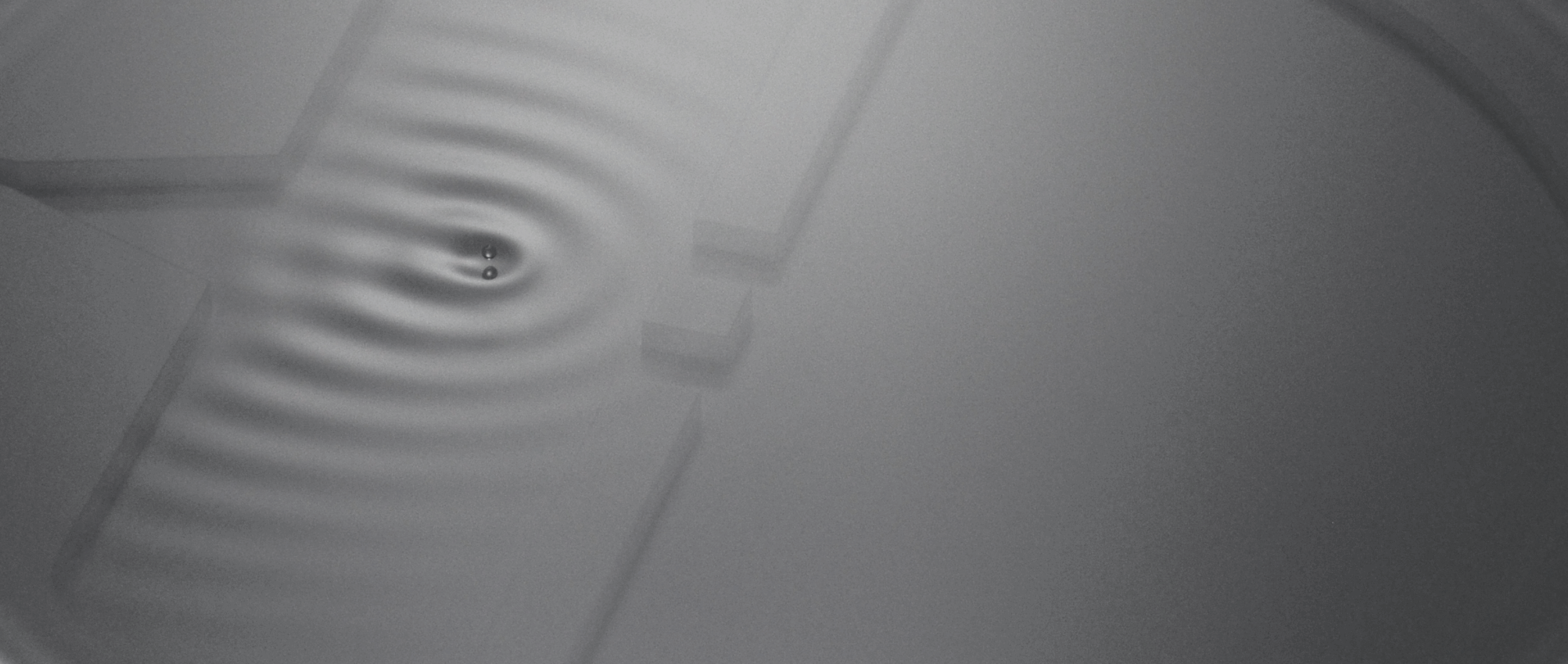
Introduction
In 2005, a student working in the fluid physicist Yves Couder’s laboratory in Paris discovered by chance that tiny oil droplets bounced when plopped onto the surface of a vibrating oil bath. Moreover, as the droplets bounced, they started to bunny-hop around the liquid’s surface. Couder soon figured out that the droplets were “surfing on their own wave,” as he put it — kicking up the wave as they bounced and then getting propelled around by the slanted contours of the wave.
As he watched the surfing droplets, Couder realized that they exactly embodied an early, largely forgotten vision of the quantum world devised by the French physicist Louis de Broglie.
A century ago, de Broglie refused to give up on a classical understanding of reality even as the unsettling outcomes of the first particle experiments suggested to most physicists that reality, at the quantum scale, is not as it seems. The standard “Copenhagen interpretation” of quantum mechanics, originated at that time by the Danish physicist Niels Bohr, broke with the past by declaring that nothing at the quantum scale is “real” until it is observed. Facts on the ground, like particles’ locations, are mere matters of chance, defined by a spread-out probability wave, until the moment of measurement, when the wave mysteriously collapses to a point, the particle hops to, and a single reality sets in. In the 1920s, Bohr persuaded most of his contemporaries to embrace the weirdness of a probabilistic universe, the inherent fuzziness of nature, and the puzzling wave-particle duality of all things.
But some physicists objected, Albert Einstein and de Broglie among them. Einstein doubted that God “plays dice.” De Broglie insisted that everything at the quantum scale was perfectly normal and above-board. He devised a version of quantum theory that treated both the wave and the particle aspects of light, electrons and everything else as entirely tangible. His “pilot-wave” theory envisioned concrete particles, always with definite locations, that are guided through space by real pilot waves — much like the waves propelling Couder’s bouncing droplets.
De Broglie couldn’t nail down the physical nature of the pilot wave, however, and he struggled to extend his description to more than one particle. At the celebrated 1927 Solvay Conference, a gathering of luminaries to debate the meaning of quantum mechanics, Bohr’s more radical views carried the day.
De Broglie’s pilot-wave vision of the quantum world was little remembered 78 years later, when the Paris droplets started bouncing. Suddenly, Couder and his colleagues had an “analogue system” for experimentally exploring de Broglie’s idea.
Straightaway, they saw the droplets exhibit surprisingly quantum-like behaviors — only traversing certain “quantized” orbits around the center of their liquid baths, for instance, and sometimes randomly jumping between orbits, as electrons do in atoms. There and in bouncing-droplet labs that soon sprang up at the Massachusetts Institute of Technology and elsewhere, droplets were seen to tunnel through barriers and perform other acts previously thought to be uniquely quantum. In reproducing quantum phenomena without any of the mystery, the bouncing-droplet experiments rekindled in some physicists de Broglie’s old dream of a reality at the quantum scale that consists of pilot waves and particles instead of probability waves and conundrums.
But a series of bouncing-droplet findings since 2015 has crushed this dream. The results indicate that Couder’s most striking demonstration of quantum-like phenomena, back in 2006 — “the experiment that got me hooked on this problem,” the fluid dynamicist Paul Milewski said — was in error. Repeat runs of the experiment, called the “double-slit experiment,” have contradicted Couder’s initial results and revealed the double-slit experiment to be the breaking point of both the bouncing-droplet analogy and de Broglie’s pilot-wave vision of quantum mechanics.
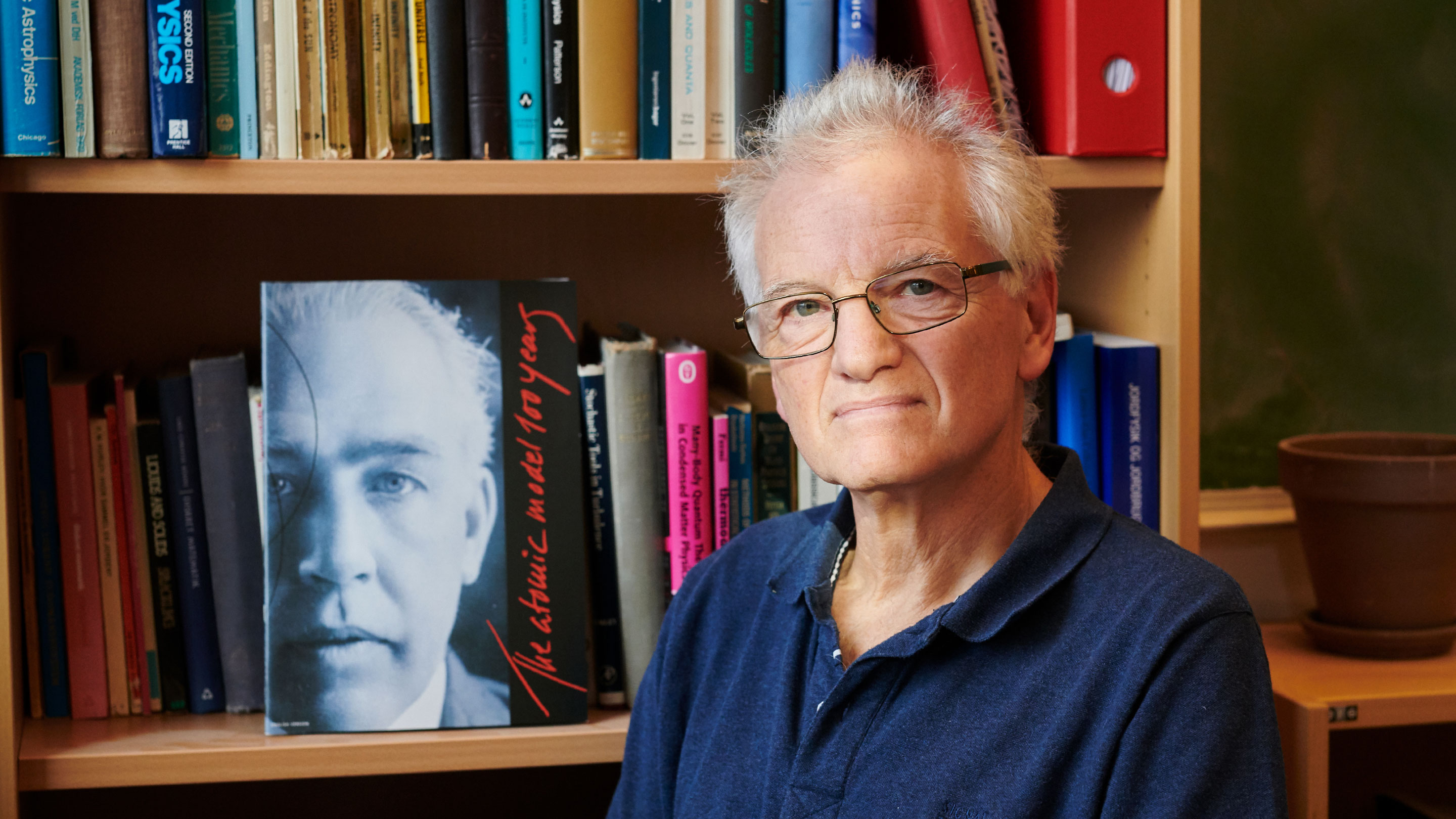
Tomas Bohr, a fluid physicist at the Technical University of Denmark, posing with an image of his grandfather, Niels Bohr, the famous pioneer of quantum mechanics.
Niels Busch for Quanta Magazine
Improbably, the person who put the irreparable crack in de Broglie’s idea is Niels Bohr’s grandson, the fluid physicist Tomas Bohr. A professor at the Technical University of Denmark who, as a child, enjoyed puzzling over riddles posed by his grandfather, Tomas Bohr heard about Couder’s bouncing-droplet experiments seven years ago and was immediately intrigued. “I felt a genuine interest in trying to see whether you could really get a deterministic quantum mechanics,” he said about his decision to enter the fray. Given his family history, he added, “maybe I also felt some obligation. I felt I should really try to see if it was true or not.”
The Heart of Quantum Mechanics
The physicist Richard Feynman called the double-slit experiment “impossible, absolutely impossible, to explain in any classical way,” and said it “has in it the heart of quantum mechanics. In reality, it contains the only mystery.”
In the experiment, particles are shot toward two slits in a barrier, and the ones that pass through the slits hit a sensor some distance away on the other side. Where any one particle ends up is always a surprise, but if you shoot many particles toward the slits, you start to see stripes develop in their detected locations, indicating places where they can and cannot go. The stripy pattern suggests that each particle is actually a wave that encounters the slitted barrier and passes through both slits at once, producing two wavefronts that converge and interfere, cresting in some places and canceling out in between. Each particle materializes in the sensor at the location of one of the crests of this strange probability wave.
Stranger still, when you add a second sensor and detect which slit each particle passes through, the interference stripes disappear, as if the probability wave, known as the wave function, has collapsed. This time, particles pass straight through their chosen slits to either of two spots on the far sensor.
To explain the double-slit experiment, a Copenhagenist will point to quantum uncertainty, arguing that the trajectory of each particle cannot be exactly known and is thus defined only probabilistically, by a wave function. After passing through both slits, as any wave would, and interfering on the other side, the wave function representing the particle’s possible locations is then “collapsed” by the sensor, which somehow selects a single reality from among the possibilities. Questions abound, both scientific and philosophical; Niels Bohr, who tended to answer questions with more questions, welcomed them.
To de Broglie, the double-slit experiment didn’t require an abstract, mysteriously collapsing wave function. Instead, he conceived of a real particle riding on a real pilot wave. The particle passes like driftwood through one slit or the other in the double-slit screen, even as the pilot wave passes through both. On the other side, the particle goes where the two wavefronts of the pilot wave constructively interfere and doesn’t go where they cancel out. De Broglie never actually derived dynamical equations to describe this complicated wave-particle-slit interplay. But with bouncing droplets in hand, Couder and a collaborator, Emmanuel Fort, moved quickly to perform the double-slit experiment, reporting their astonishing results in Physical Review Letters in 2006.
After recording the trajectories of 75 bouncing droplets through a double-slit barrier, Couder and Fort thought they detected rough stripes in the droplets’ final locations — an interference-like pattern that seemed as if it could only come from the pilot wave. Double-slit interference, considered “impossible to explain in any classical way,” was happening without mystery before everyone’s eyes. Drawn by the potential quantum implications, the fluid dynamicist John Bush started up a bouncing-droplet lab of his own at MIT and led others to the cause. Tomas Bohr heard Couder talk about his results in 2011 and later discussed the experiments at length with Bush. He teamed up with an experimentalist colleague, Anders Andersen, to study bouncing droplets further. “We really became fascinated with, in particular, the double-slit experiment,” Andersen said.
Bohr and Andersen’s group in Denmark, Bush’s team at MIT, and a team led by the quantum physicist Herman Batelaan at the University of Nebraska all set out to repeat the bouncing-droplet double-slit experiment. After perfecting their experimental setups, getting rid of air currents, and setting oil droplets bouncing on pilot waves toward two slits, none of the teams saw the interference-like pattern reported by Couder and Fort. Droplets went through the slits in almost straight lines, and no stripes appeared. The French pair’s earlier mistake is now attributed to noise, faulty methodology and insufficient statistics.
“The double-slit experiment, for me — it’s a bit of a disappointment,” said Milewski, who is the head of the department of mathematical sciences at the University of Bath.
Bush’s detailed double-slit studies, published earlier this year, showed no hint of interference, but he still thinks it might be possible to generate an interference pattern with pilot waves when the right combination of parameters is found — the right frequency for the vibrating fluid bath, perhaps, or a necessary addition of noise. Milewski shares this hope. However, in the Denmark group’s paper reporting their null double-slit results, Tomas Bohr presented a thought experiment that appears to demolish de Broglie’s pilot-wave picture completely.
In this hypothetical “gedanken” version of the double-slit experiment, the particles, before arriving at the slitted barrier, have to pass to one side or the other of a central dividing wall. In standard quantum mechanics, this wall can be very long, and it won’t matter, because the wave function representing the possible paths of a particle will simply go both ways around the wall, pass through both slits, and interfere. But in de Broglie’s picture, and likewise in the bouncing-droplet experiments, the driving force of the whole operation — the particle — can go only one way or the other, losing contact with the part of the pilot wave that passes to the other side of the wall. Unsustained by the particle or droplet, the wavefront disperses long before reaching its slit, and there’s no interference pattern. The Danish researchers verified these arguments with computer simulations.
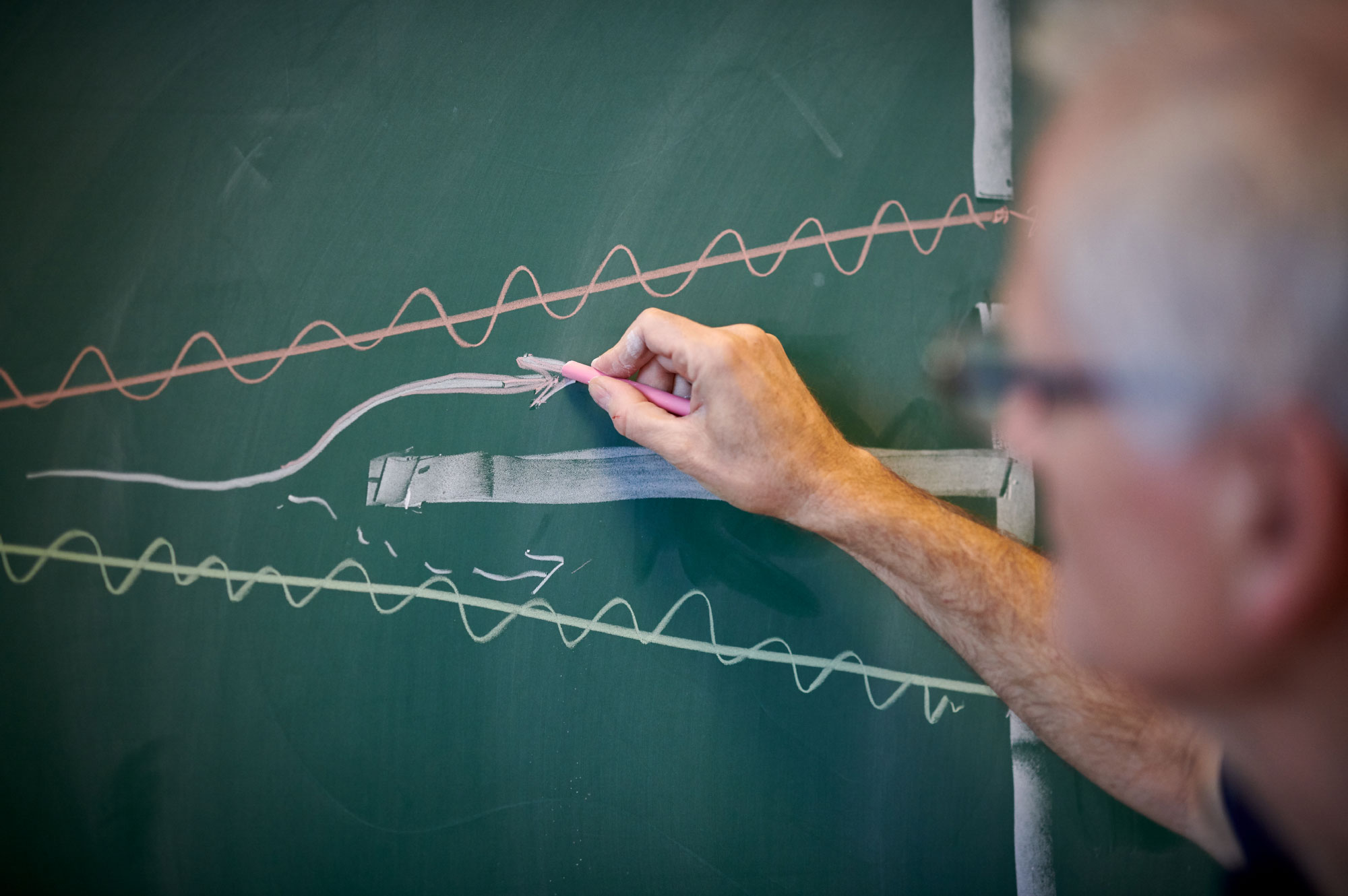
Tomas Bohr’s variation on the famous double-slit experiment considers what would happen if a particle must go to one side or the other of a central dividing wall before passing through one of the slits. Quantum mechanics predicts that the wall will have no effect on the resulting double-slit interference pattern. Pilot-wave theory, however, predicts that the wall will prevent interference from happening.
Niels Busch for Quanta Magazine
In explaining his decision to keep studying bouncing droplets, Bush said, “I never liked gedanken experiments. The beauty of this situation is you can actually do the experiment.” But the dividing-wall thought experiment highlights, in starkly simple form, the inherent problem with de Broglie’s idea. In a quantum reality driven by local interactions between a particle and a pilot wave, you lose the necessary symmetry to produce double-slit interference and other nonlocal quantum phenomena. An ethereal, nonlocal wave function is needed that can travel unimpeded on both sides of any wall. “To get the real quantum mechanical result, it’s really important that the possible paths of the particle enter in a democratic way,” Tomas Bohr said. But with pilot waves, “since one of these sides in the experiment carries a particle and one doesn’t, you’ll never get that right. You’re breaking this very important symmetry in quantum mechanics.”
A Matter of Taste
Experts note that the simplest version of de Broglie’s theory was bound to fail. In describing individual particles guided by corresponding pilot waves, de Broglie didn’t account for the way multiple interacting particles become “entangled,” or defined by a single, joint, nonlocal wave function that keeps their properties correlated even after the particles have traveled light-years apart. Experiments with entangled photons starting in the 1970s proved that quantum mechanics must be nonlocal. A theory of local interactions between a particle and its pilot wave like de Broglie’s would need to get a whole lot weirder in the jump from one particle to two to account for nonlocal entanglement.
Until his death in 1987, de Broglie questioned the arguments about nonlocality and entanglement and continued to believe that real pilot waves might somehow stir up the necessary long-distance connections. That improbable dream, shared by some bouncing-droplet experimenters, might have been allowed to stubbornly persist until now, but with pilot waves unable to even generate double-slit interference in the case of single particles, the dream collapses like a scrutinized wave function.
Early on, de Broglie did offer a kind of compromise, a version of his theory that was promulgated again in 1952 by the physicist David Bohm, and which is now known as Bohmian mechanics or de Broglie-Bohm theory. In this picture, there’s an abstract wave function that extends through space — an entity that’s just as mysterious in this theoretical framework as it is in the Copenhagen interpretation — as well as real particles somewhere in it. Proofs in the 1970s showed that de Broglie-Bohm theory makes exactly the same predictions as standard quantum mechanics. However, with one element of classical reality restored — concrete particles — new mysteries arise, like how or why a mathematical wave function that’s spread everywhere in space is bolted in certain places to physical particles. “Quantum mechanics is not less weird from that perspective,” Tomas Bohr said. Most physicists agree, but it’s really just a matter of taste, since the experimental predictions are identical.
Tomas Bohr attributes his grandfather’s certainty that nature is incurably weird at the quantum scale to Niels Bohr’s most important physics research: his 1913 calculations of the electronic energy levels of the hydrogen atom. Bohr realized that when electrons jump between orbits, releasing quantized packets of light, there was no mechanical picture of the situation that made sense. He couldn’t relate the electrons’ energy levels to their rotational motion. Even causality failed, because electrons seemingly know before they jump where they are going to land, in order to emit a photon of the correct energy. “He was probably more aware than most of how weird that whole thing was,” Tomas Bohr said. “He was just somehow philosophically inclined in such a way that he was ready to accept that nature was that strange — and most people were not.”
In the last few years, Tomas has often wondered what his grandfather would have said about the bouncing-droplet experiments. “I think he would have been very interested,” he said, adding with a laugh, “He would probably have been much quicker than me to figure out what he thought about it. But he would have thought it was an ingenious thing, that you could generate such a system, because it’s surprisingly close to what de Broglie was talking about.”