A Cosmic Burst Repeats, Deepening a Mystery
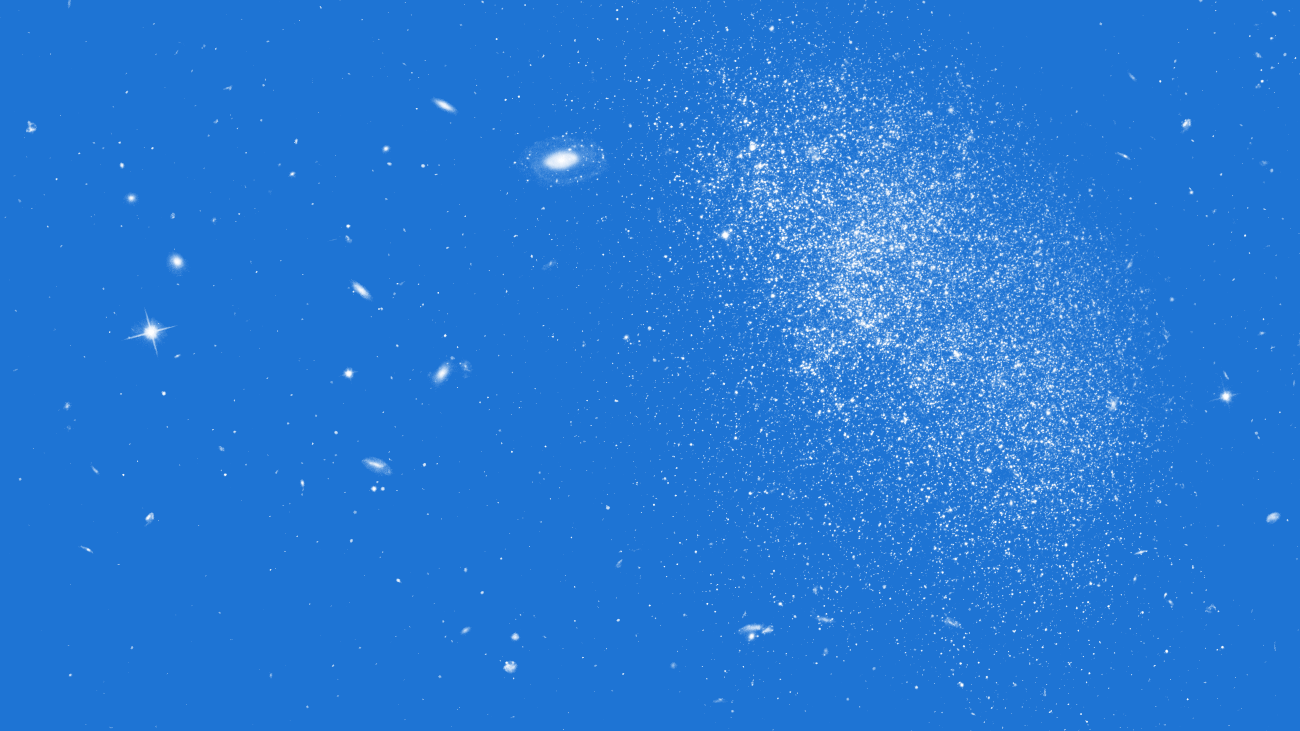
Olena Shmahalo/Quanta Magazine
Introduction
“A minor point of interest regarding the Spitler Burst.” The email subject line popped up on Shami Chatterjee’s computer screen just after 3 in the afternoon on Nov. 5, 2015.
When Chatterjee read the email, he first gasped in shock — and then sprinted out of his Cornell University office and down the corridor to tell a colleague. Twenty-eight minutes later, when he started to draft a reply, his inbox was already buzzing. The email thread grew and grew, with 56 messages from colleagues by midnight.
For nearly a decade, Chatterjee and other astrophysicists on the thread had been trying to understand the nature of short, superenergetic flashes of radio waves in space. These “fast radio bursts,” or FRBs, last just a few milliseconds, but they are the most luminous radio signals in the universe, powered by as much energy as 500 million suns. The first one was spotted in 2007 by the astronomer Duncan Lorimer, who together with one of his students stumbled upon the signal accidentally in old telescope data; at the time, few believed it. Skeptics suspected interference from mobile phones or microwave ovens. But more and more FRBs kept showing up — 26 have been counted so far, including the Spitler burst, detected by the astronomer Laura Spitler in data from 2012 — and scientists had to agree they were real.
The question was, what causes them? Researchers sketched dozens of models, employing the gamut of astrophysical mysteries — from flare stars in our own galaxy to exploding stars, mergers of charged black holes, white holes, evaporating black holes, oscillating primordial cosmic strings, and even aliens sailing through the cosmos using extragalactic light sails. For scientists, the FRBs were as blinding as flash grenades in a dark forest; their power, brevity and unpredictability simply made it impossible to see the source of the light.
The email alerting Chatterjee and colleagues to a “minor point of interest” changed all that. Its sender was Paul Scholz, a graduate student at McGill University in Montreal and a collaborator of Chatterjee’s. He was performing astrophysical “due diligence,” sifting with the help of a supercomputer through all the telescope data that had been collected from the part of the sky where the Spitler burst originated, to see whether the source might send a second signal. According to Chatterjee, after two years of doing this and seeing nothing, expectations had dimmed, but “it was just part of a regular rotation; you put in a few minutes to look for it anyway just in case.”
And suddenly, just like that, Scholz had spotted a repeater. The discovery was “both amazing and terrifying,” Chatterjee said — amazing, because “everyone knew that FRBs don’t repeat,” and terrifying because of the gargantuan energy required to produce even one of these bursts. Perhaps the only thing fiercer than emitting the energy of 500 million suns is doing it again.
The discovery instantly killed off a large number of the previously proposed models — at least, as explanations for this particular FRB. Any model that presumed a one-time cataclysm, such as a star’s dying flash or the merger of stars or black holes, was out. Still, many models remained, some pointing to sources within the galaxy, and others in galaxies far away.
As the repeater narrowed down the options, Scholz took a stab at guessing the source: “Extragalactic magnetar” he wrote in his initial email, referring to a young neutron star with an extremely powerful magnetic field. The first person to reply, Maura McLaughlin, an astrophysicist at West Virginia University in Morgantown, wrote: “WOW!!!!!!! Extragalactic radio magnetar sounds right to me.” It quickly became the most popular theory, but not the only one, and one not without difficulties.
To reveal the true nature of the burst, the scientists had to figure out the location of the source. But that wasn’t easy. To detect an FRB in the first place, a telescope must happen to be pointed directly at the area of the sky where it originates. This may explain why only 26 have been spotted during the past decade — with telescope time in high demand, there aren’t enough instruments available to watch every patch of the sky and wait. But even when an FRB is detected, scientists can’t pinpoint its origin within a telescope’s field of view. To localize a burst, they need to detect it with several telescopes and compare the signals to determine its exact position.
Now, though, there was a chance, provided that the repeater would flash a third time.
Flashes in the Dark
Within hours of Scholz’s email to a team of around 40 scientists — collaborators on a project called the Pulsar Arecibo L-band Feed Array survey — members of the team managed to secure time on the Very Large Array (VLA), the group of 27 radio telescopes in New Mexico made famous by the movie Contact. The VLA is large enough to make the combined measurements needed to localize a burst. At first, the team asked for 10 hours of VLA time, during which they planned to scan the relevant region of the cosmos every few milliseconds, hoping to catch the FRB flash. “It’s like making a movie of the sky at 200 frames per second,” said Chatterjee, who is one of the collaboration’s leaders. “And we made this movie over 10 hours and we saw absolutely nothing.”
They put in for another 40 hours of VLA time, and made yet another movie of the sky in the radio spectrum at 200 frames per second. Again, they saw nothing. Worried, the researchers had to beg for yet more time. They managed to persuade VLA management to give them another 40 hours on the telescope. This time, during a first test run, they spotted their flash.
“It looks like the fast radio burst came out to play today,” Casey Law, the researcher monitoring the VLA in real time, wrote in an email to the rest of the team.
The repeater would go on to make eight reappearances. Bizarrely, the bursts seemed to be totally random. After 50 hours of seeing none during previous observations, the team now spotted them frequently, including, one time, a “double burst” of signals only 23 seconds apart.
The repeat signals allowed the team to localize the source. To nearly everybody’s surprise, as reported in January in the journal Nature, the bursts originated in a small “dwarf irregular” galaxy, one about a gigaparsec (just over 3 billion light years) away. This made the strength of the signal and its frequent repetitions even more astonishing. “If you’re detecting a bright flash from a gigaparsec, there’s an awful lot of energy associated with it,” Chatterjee said. “The more energy you associate with each event, the harder it gets to explain the repetition. Basically, what’s recharging the battery so quickly?”
Magnetars Imagined
In February, experts gathered at a conference in Aspen, Colorado, to discuss FRBs for the first time since the repeater’s location was identified. Most astrophysicists agreed that both the source’s distance and setting are consistent with the theory that it is a magnetar. It’s one of the few candidate sources capable of producing such a strong signal from so far away. And, according to Laura Spitler, namesake of the Spitler burst and a researcher at the Max Planck Institute for Radio Astronomy, in Bonn, Germany, magnetars generally form from stellar explosions called Type-I superluminous supernovas. These events occur disproportionately often in dwarf irregular galaxies, which are thought to be similar to some of the earliest galaxies that populated the universe.
Each successive generation of stars that have lived and died since the Big Bang has fused protons and neutrons together into heavier and heavier elements, increasing what astronomers call the “metallicity” of the universe. But dwarf irregular galaxies are likely to have formed from lightweight hydrogen and helium that remain pristine from when the universe was young. Their low metallicity allows these tiny galaxies to produce more massive stars, and, probably because massive stars have stronger magnetic fields, their explosive deaths can leave behind highly magnetized neutron stars, or magnetars.
However, magnetar proponents like Brian Metzger of Columbia University acknowledge that it would take a very special magnetar to unleash such monstrous FRBs in quick succession. “A neutron star bursting at this rate for thousands of years would quickly run out of fuel,” he said. His best guess is that the repeater is a very young magnetar — probably less than 100 years old.
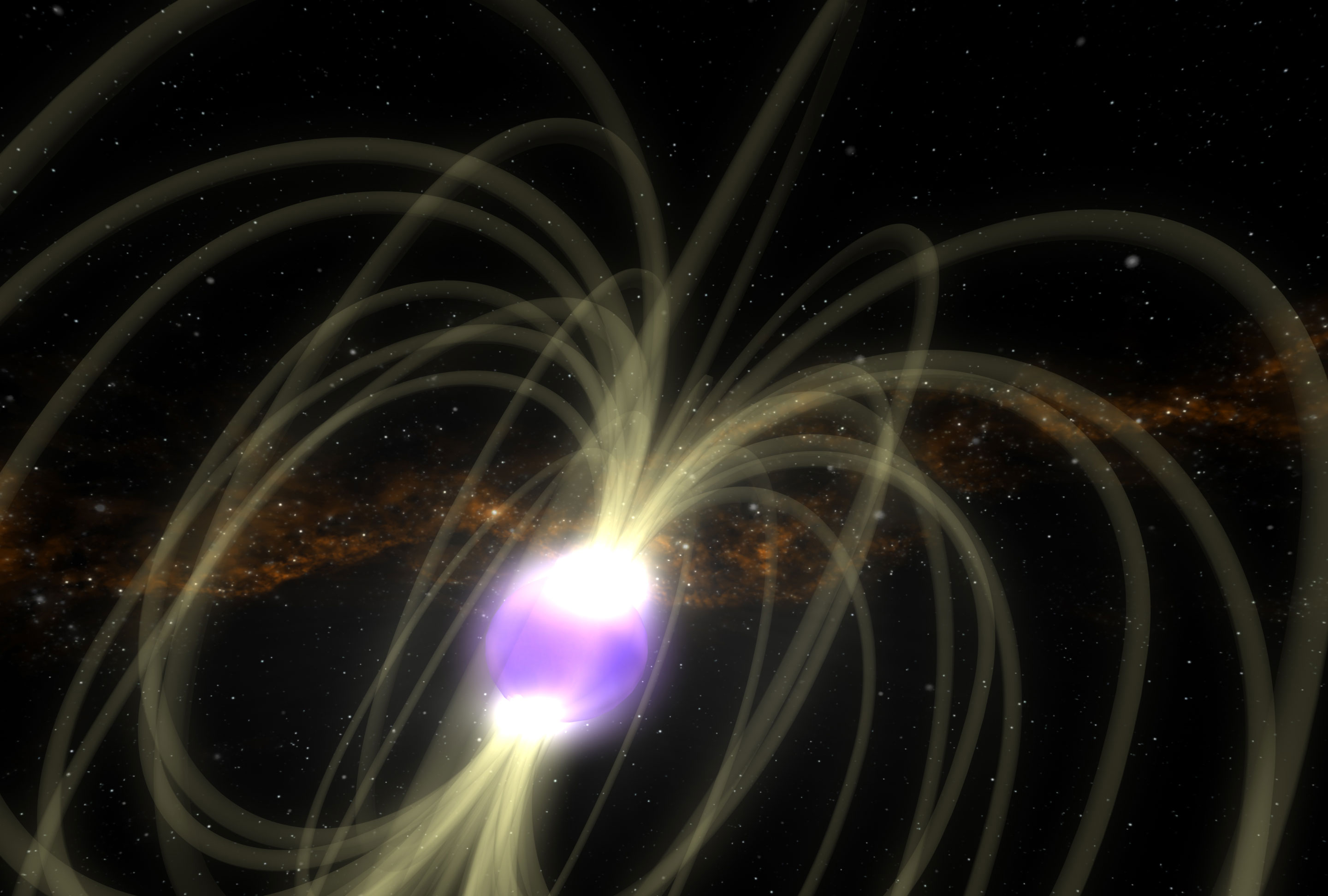
Artist’s rendition of a highly magnetized neutron star, or magnetar.
NASA
If the young-magnetar theory is correct, then — according to one possible version of the story — we have to envisage a newborn, superdense neutron star cloaked in a powerful and highly unstable magnetic field. This magnetar also remains embedded in an expanding cloud of debris from a supernova explosion. As the newborn magnetar’s magnetic field changes and reconfigures and reconnects, it pumps energy into the surrounding gas and dust cloud. This in turn absorbs the energy and then occasionally experiences shocks, releasing sudden, gargantuan bursts of energy into the cosmos.
This story is still just hypothetical, but astrophysicists point to a piece of supporting evidence: The FRBs are coming from the same vicinity as a steady source of radio emission — possibly the background signal from the expanding debris cloud that surrounds the young magnetar. Bryan Gaensler, an astrophysicist at the University of Toronto, said that as this debris expands, the properties of this background signal should change. “If we see this happen, it is more support for the young magnetar model,” he said, “plus it gives us information about the magnetar’s environment and birth process.”
However, Gaensler warned that there are some issues with the magnetar model. For starters, why have we not seen any FRBs from magnetars that are much closer to Earth? For instance, the magnetar SGR 1806-20 in the Milky Way gave off a giant gamma-ray burst in December 2004, yet no FRBs. “If it had produced an FRB as powerful as the repeater,” Gaensler said, “it would have been so bright that we would have seen it even through radio telescopes that were pointing in completely different directions at that moment.”
On the other hand, he said, maybe magnetars produce FRBs in narrow beams or jets. “We’d then only see the FRB when the beam is pointing right at us. Maybe SGR 1806-20 produces FRBs all the time, but pointed in a different direction. We don’t really know.”
Either way, if the researchers fail to spot a dimming of the steady radio source associated with the Spitler burst, then the whole magnetar theory may be ready for the astrophysical scrapheap.
Another idea floating around is that FRBs are emitted by active galactic nuclei, or AGNs — superluminous regions at the centers of some galaxies. AGNs are thought to be powered by supermassive black holes, and many of them have jets that could beam FRBs into space. Yet this theory is less popular, said Metzger, because AGNs usually exist in bigger galaxies, not dwarfs.
There are other possibilities. “New theories are continuing to show up,” said Emily Petroff, an astrophysicist at the Netherlands Institute for Radio Astronomy. “Every time a new observational paper about an FRB comes out there are a few new theory papers that rush in to describe it, which is kind of a fun place for the field to be because it’s not often that observations jump so far ahead of theory in astronomy.”
One key question is whether the repeater is representative of all FRBs — in other words, whether all FRBs repeat. It’s possible that they all do, but that most of the time only the first, brightest bursts are seen. “The current data cannot lead to a firm conclusion,” Chatterjee said.
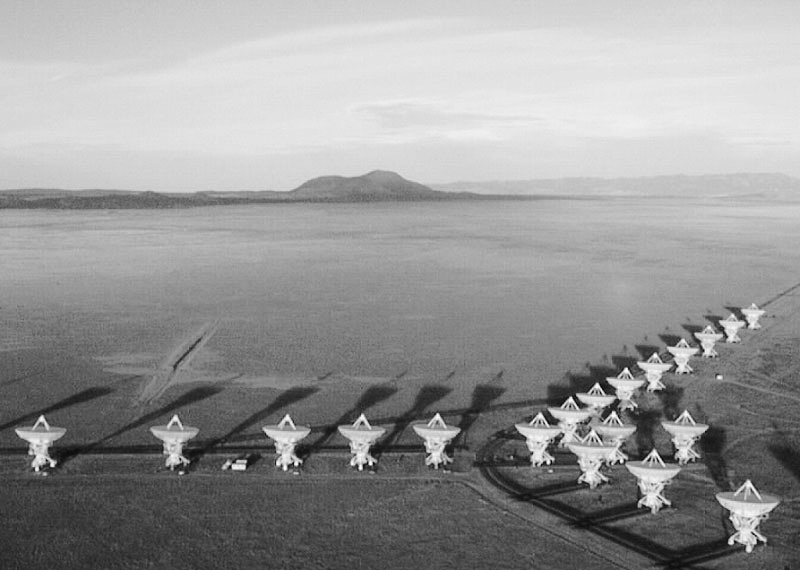
The Very Large Array, a group of 27 radio antennas in New Mexico that has been in operation since 1980, allows data from each 25-meter-wide antenna to be combined electronically to localize signals.
National Radio Astronomy Observatory
Array of Possibilities
The repeater may have created more questions than it delivered answers. To know more, scientists need more FRBs, and more repeaters. They hope to localize more bursts to see whether they usually live in dwarf irregular galaxies, and whether they all appear alongside steady radio sources, both of which would support the newborn-magnetar theory. They also plan to keep monitoring the steady radio emission from the vicinity of the Spitler burst to see if its properties change in time, as expected based on that theory.
It might turn out that more than one astrophysical mechanism can make an FRB. Upcoming next-generation radio telescopes, such as the Square Kilometer Array, slated to be the world’s largest radio telescope, and a suite of smaller planned telescopes called “light buckets” should help astronomers sort out the possibilities. The light buckets will act like floodlights in reverse, pulling in radio waves from a huge swath of sky. According to Gaensler, they should spot more FRBs in one day than have been found in the past 10 years, providing ample opportunity to look for repeaters and localize signals. Other future telescopes, including the VLA equipped with a feature called Realfast, should be able to pinpoint the locations of FRBs even if they don’t repeat.As patterns emerge in the locations of FRBs and their origins become clear, scientists hope to use the signals to better understand the nature of their host galaxies, and to more precisely map the distribution of matter in the universe. If they can locate FRB beacons sitting at different cosmological distances, then according to Bing Zhang, an astrophysicist at the University of Nevada, Las Vegas, it should be possible to measure the amount of matter spread out in the vast emptiness of space between us and the sources of the flashes. This might help confirm simulations that suggest the universe is rather clumpy, with clusters and voids. And it could give researchers a better handle on the distribution of the invisible “dark matter” that also seems to pervade the cosmos, Zhang added.
“The breakthrough with the repeating FRB came from being able to measure its precise position,” Gaensler said. Now, scientists are eager to pin down more and more bursts. “The results and advances will be spectacular,” he said.
This article was reprinted on Wired.com and in German on Spektrum.de.