Heat Destroys All Order. Except for in This One Special Case.
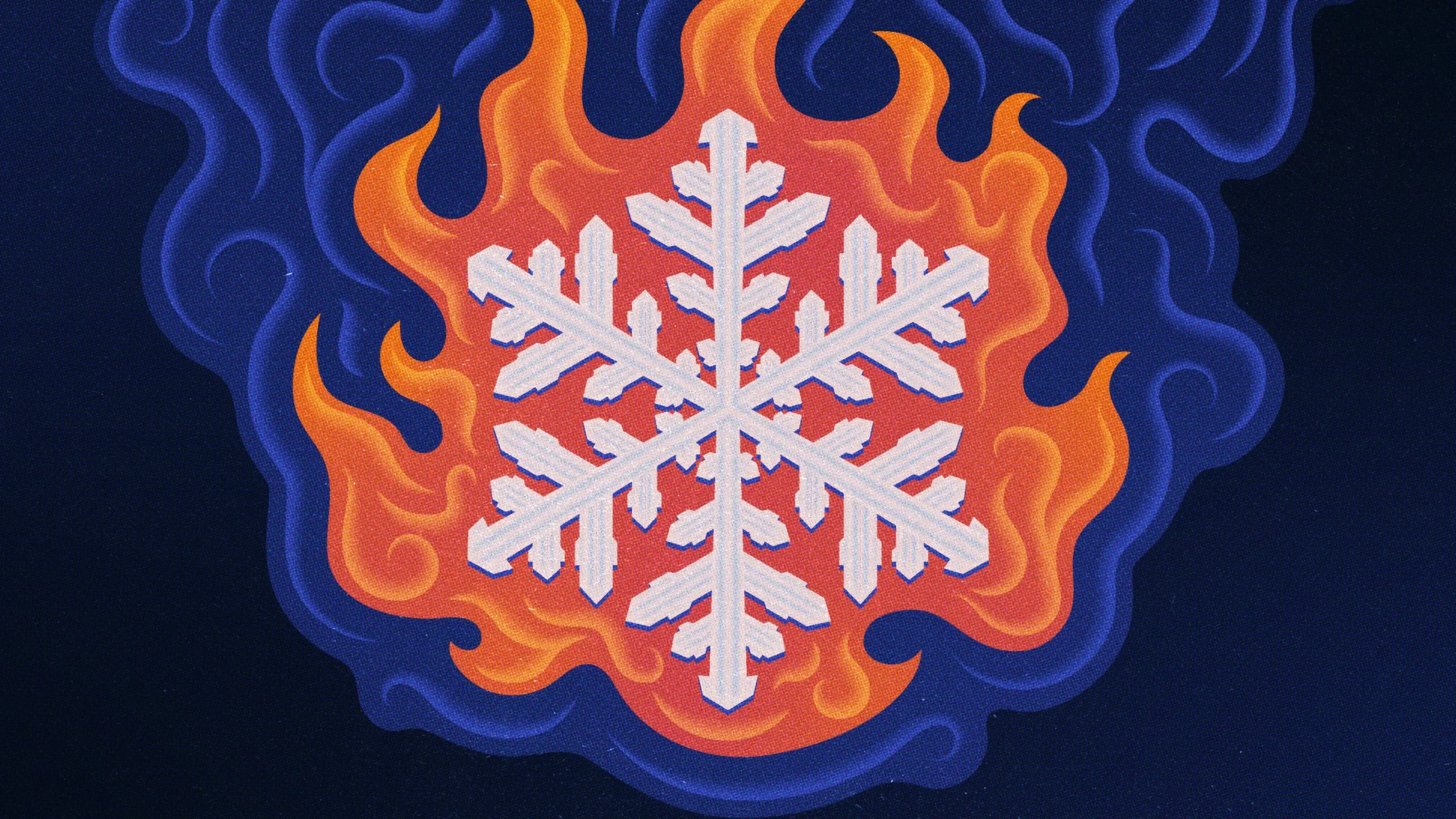
Kristina Armitage/Quanta Magazine
Introduction
Sunlight melts snowflakes. Fire turns logs into soot and smoke. A hot oven will make a magnet lose its pull. Physicists know from countless examples that if you crank the temperature high enough, structures and patterns break down.
Now, though, they’ve cooked up a striking exception. In a string of results over the past few years, researchers have shown that an idealized substance resembling two intermingled magnets can — in theory — maintain an orderly pattern no matter how hot it gets. The discovery might influence cosmology or affect the quest to bring quantum phenomena to room temperature.
Several physicists expressed surprise and delight that such an effect is possible, even if only in theory.
“It just hits you in the face because it’s not what you expect,” said Fabian Rennecke, a researcher at the Institute for Theoretical Physics in Giessen, Germany, who was not involved in the work.
“I am quite intrigued and am thinking how to find a concrete realization of this framework,” said Jörg Schmalian, a physicist at the Karlsruhe Institute of Technology in Germany.
Turning Up the Heat
The discovery was sparked by an audience question at a lecture at the Hebrew University of Jerusalem in 2019. Zohar Komargodski, a physicist visiting from Stony Brook University, had commented that any form of order — such as the regular spacing of atoms in a solid or the alignment of atoms in a magnet — inevitably breaks down at sufficiently high temperatures. An audience member, Eliezer Rabinovici of Hebrew University, asked Komargodski if he was certain this was true. After the talk, the two began to collaborate, along with other colleagues.
They weren’t the first physicists to wonder. In the 1950s, Isaak Pomeranchuk had calculated that slightly heating supercooled liquid helium-3 atoms would make them freeze. A crystal known as Rochelle salt, which is used as a laxative, shifts to a more ordered structure at warmer temperatures. Curiosities like these motivated the physicist and future Nobel laureate Steven Weinberg to develop an idealized quantum theory of heat-resistant order in the 1970s. But in both liquid helium and Rochelle salt, further heating destroys the order. And Weinberg’s theory also failed above a certain temperature.
Was it possible for some pattern to persist forever, no matter how hot it got? Komargodski, Rabinovici and collaborators aimed to find out.
Heatproof Order
The physicists homed in on magnetism.
Picture a bunch of atoms arranged in a square grid. Each atom acts like a mini magnet with a north pole that points up or down.
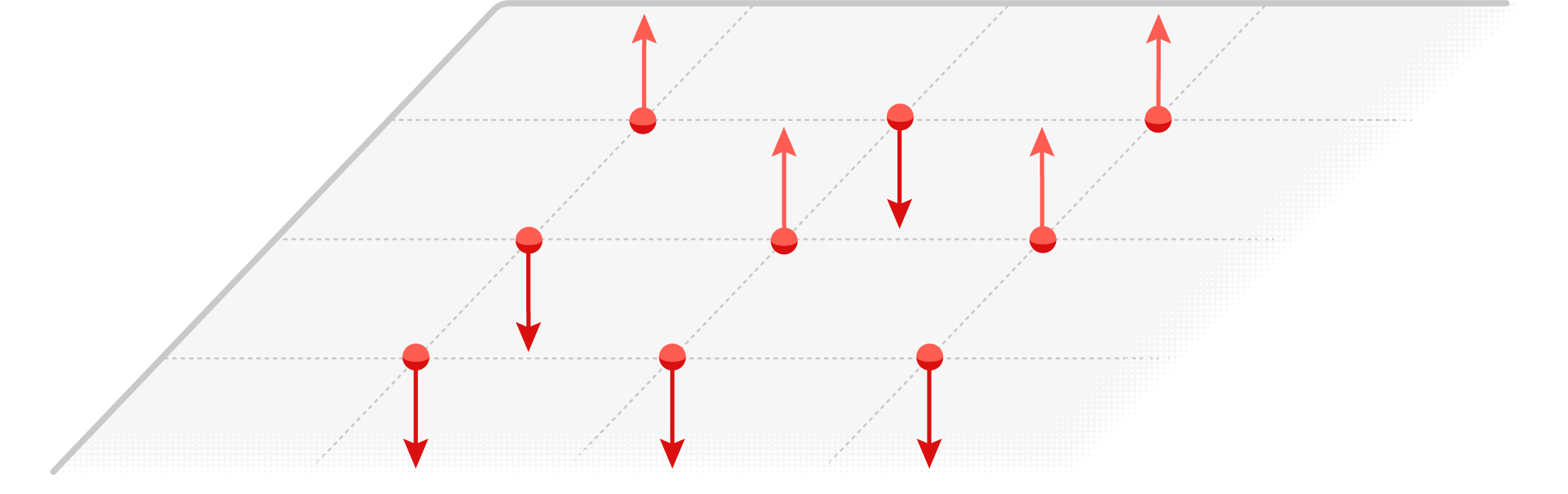
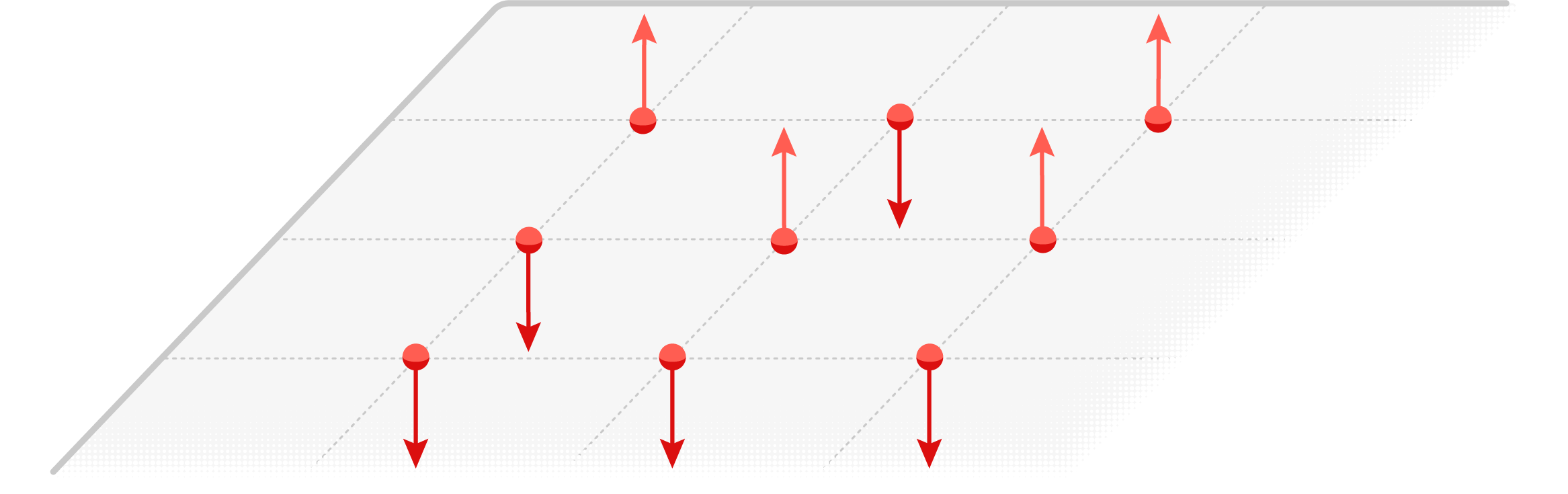
Mark Belan/Quanta Magazine
If the atoms line up in some pattern — all pointing the same way, for instance — the material has magnetic order.
Imagine laying this grid directly on top of a second atomic grid. These new atoms can swing freely, pointing in any direction as opposed to only up or down. Nearby atoms will interact, with ripples in one grid triggering ripples in the other.
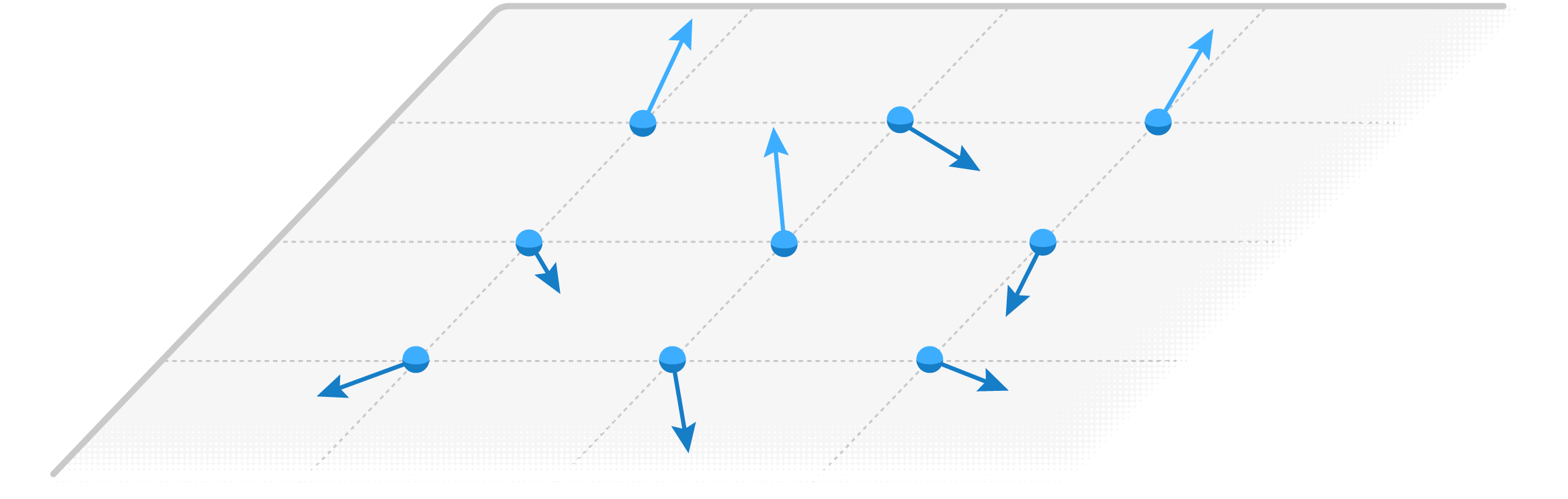
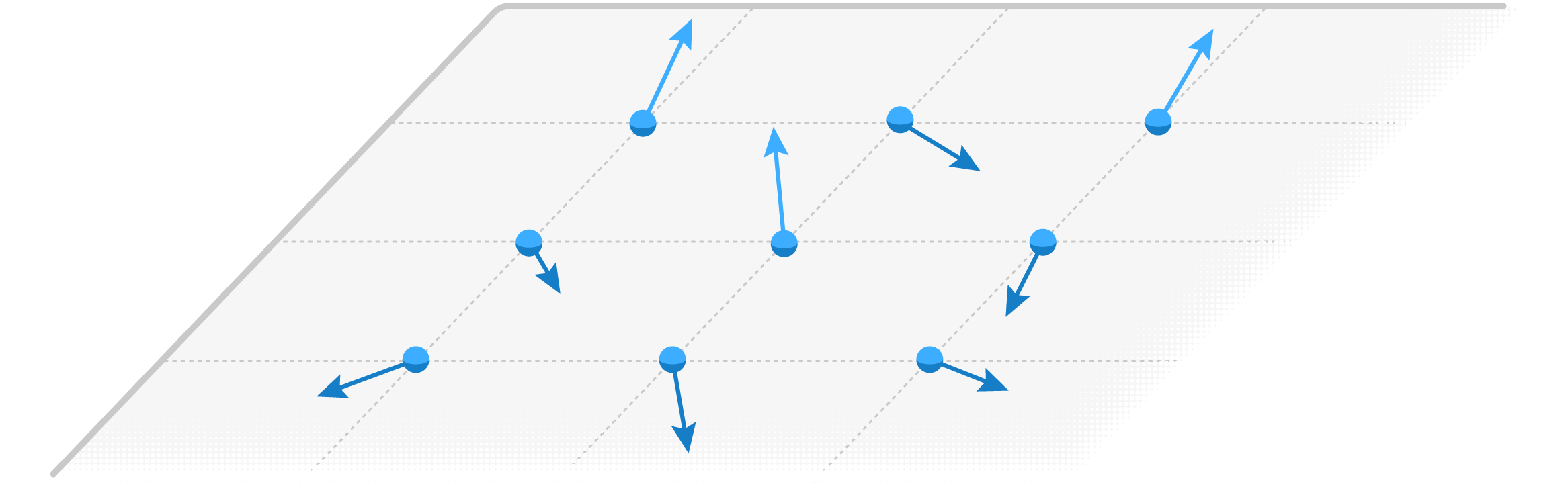
Now zoom out until the grid lines disappear and the system becomes a smooth sheet — a quantum field. The atoms have vanished, but the field still has two magnetic arrows at every point: one pointing straight up or down and another pointing in any direction.
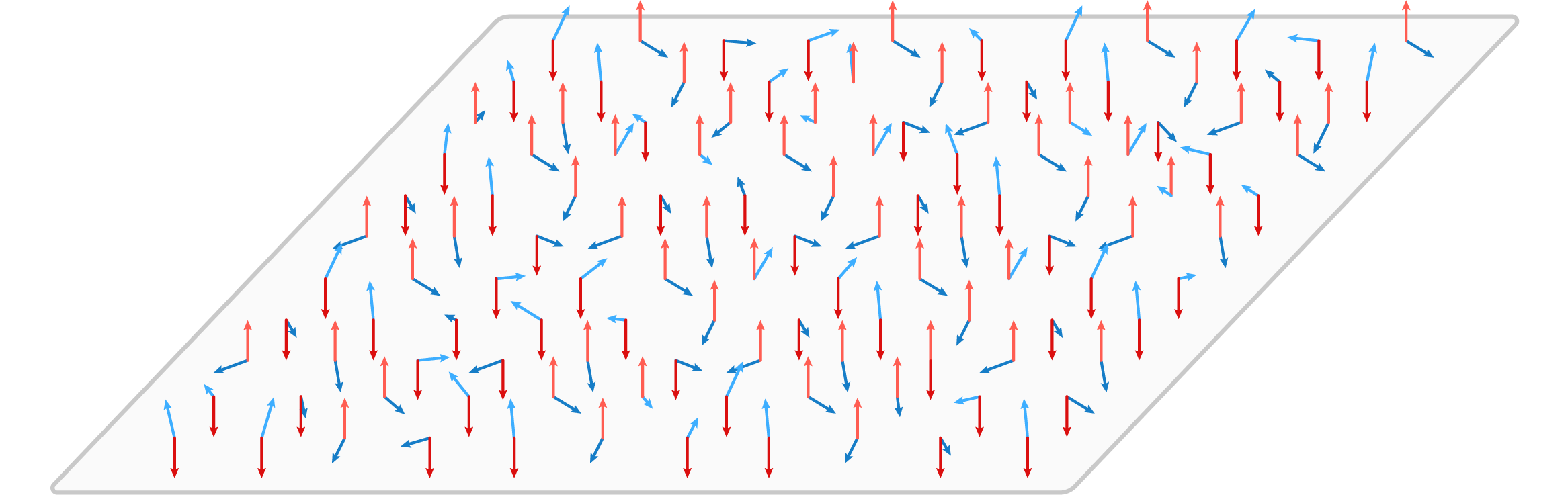
It’s this kind of idealized field that the researchers realized could maintain magnetic order at every temperature.
Under cool conditions, the up-down arrows nudge each other into alignment — all up, say — while the freewheeling arrows point in random directions. As the temperature rises, one would expect the thermal energy to start flipping all the arrows violently, washing out any alignment. But it doesn’t. The free arrows pinwheel around more, stabilizing the magnetic order in the up-down arrows. And this arrangement survives even as the temperature climbs higher for all eternity. The magnetic order never melts away.
A caveat is that the trick seems to work best when the freewheeling arrows have a great deal of freedom. Komargodski imagines arrows that are free to point in any direction in an abstract space of hundreds of dimensions. These need not literally be directions in real space, however. They represent all the ways the field can vary mathematically from point to point.
In 2020, Komargodski and collaborators calculated that magnetism will endure in this system up to infinite temperatures, but their math relied on the assumption that probabilities need not add up to exactly 100% — a physical and logical impossibility.
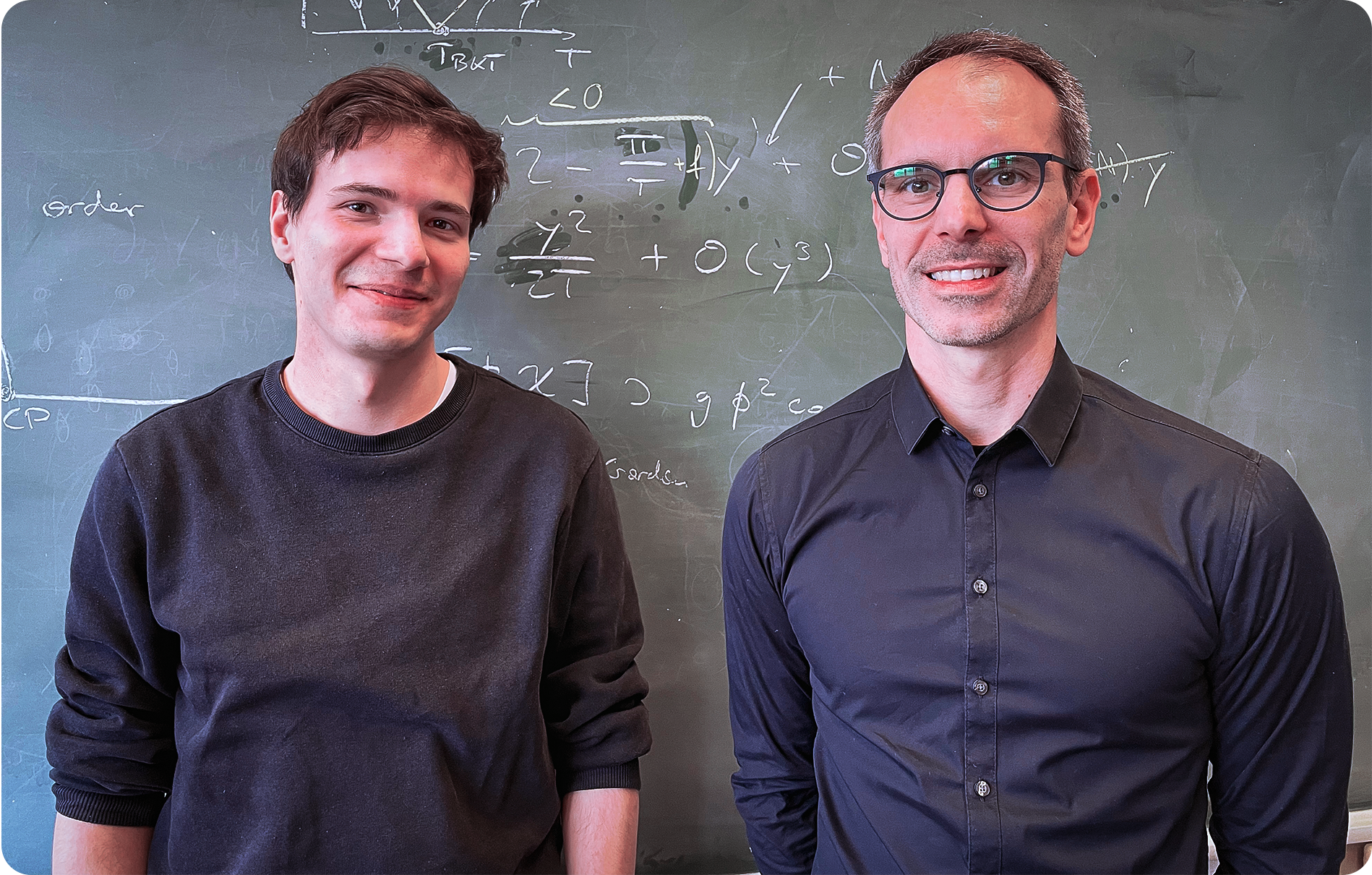
Bilal Hawashin (left) and Michael Scherer were able to rid the infinite-order system of an unphysical assumption.
Cinja Bosel
They gave up the search for a more solid proof until this fall, when a team of European physicists — Michael Scherer, Junchen Rong and Bilal Hawashin — advanced the case. They restored 100% probabilities (at the price of ignoring certain mild magnetic interactions) and found that order persisted for arrows spinning through as few as 15 abstract dimensions. Their work inspired Komargodski and a new collaborator, Fedor Popov, to return to the problem and finally find a rigorous proof of the unmeltable order that overcomes all previous shortcomings. They posted a preprint of a paper describing the work in December and will soon submit it for publication.
“We can safely say this is something that quantum field theory realizes,” said Scherer, who thinks the new proof will hold up. “The question is: What do we do with this now?”
New Inspiration
Knowing that order can theoretically survive any amount of heat might influence theories of the universe’s birth. The typical story is that order developed as the inferno of the young universe cooled, but the recent work highlights stranger possibilities.
“Now you have in your toolbox new theories that you can use,” said Francesco Sannino, a physicist at the University of Southern Denmark who has independently found proof of heat-resistant order in fundamental quantum theories.
This new way of heatproofing quantum patterns may also inspire physicists who study delicate phenomena like superconductivity, a phase in which electric current flows with no resistance. Normally, heat disrupts the quantum ordering that makes superconductivity possible, limiting its applications. But perhaps in a material borrowing key features from the magnetic theory, perfect currents could be made to endure rising temperatures.
“I’m pretty sure the same story would still hold for a superconductor,” Schmalian said.