How Animals Map 3D Spaces Surprises Brain Researchers
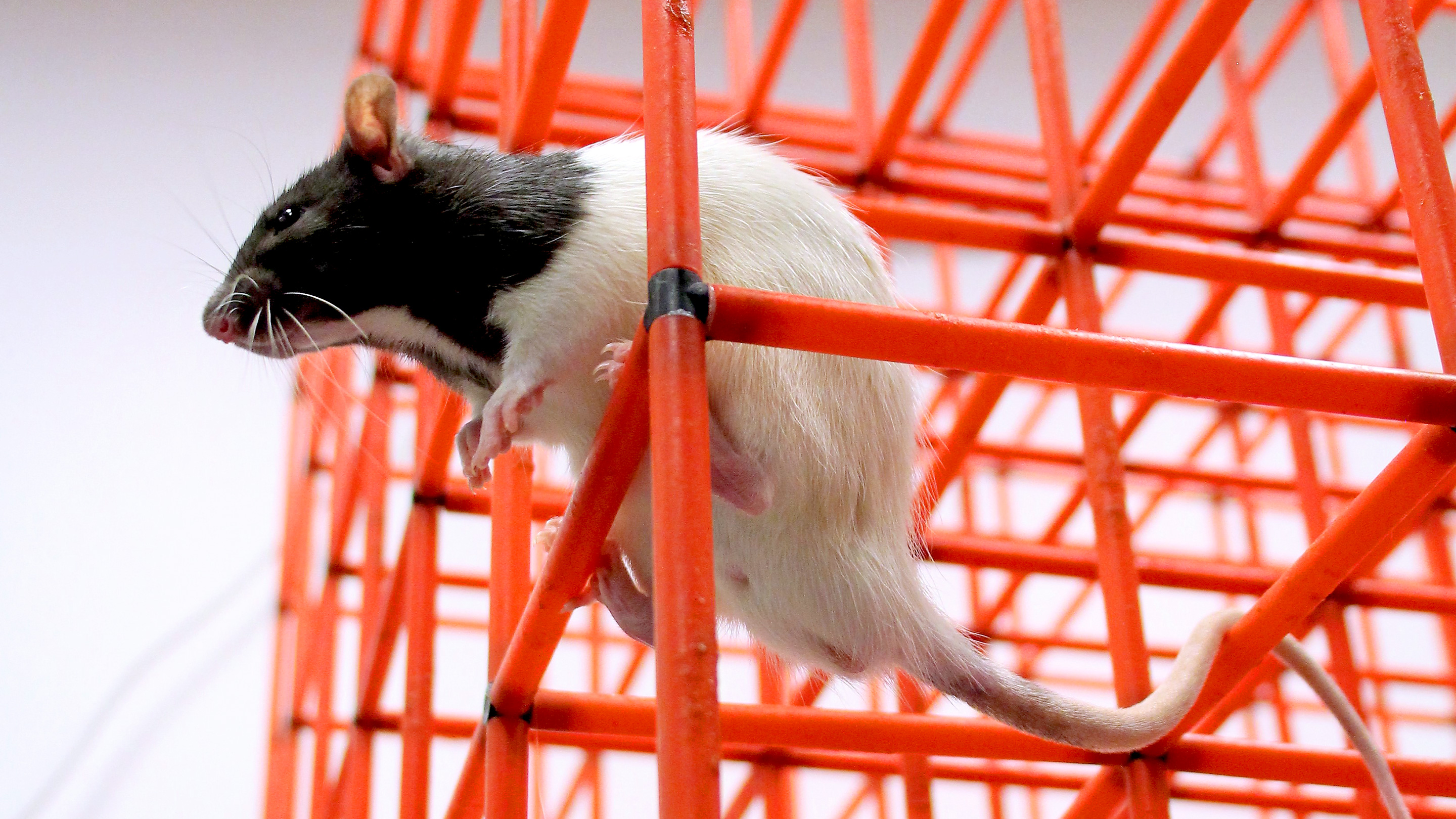
To study how animals’ brains represent three-dimensional space, some researchers have coaxed rats to explore latticelike mazes and other complex environments.
Roddy Grieves
Introduction
Leaping, scurrying, flying and swimming through their natural habitats, animals compile a mental map of the world around them — one that they use to navigate home, find food and locate other points of vital interest. Neuroscientists have chiseled away at the problem of how animals do this for decades. A crucial piece of the solution is an elegant neural code that researchers uncovered by monitoring the brains of rats in laboratory settings. That landmark discovery was awarded a Nobel Prize in 2014, and many scientists think the code could be a key component of how the brain handles other abstract forms of information.
Yet lab animals in a box with a flat floor only need to navigate through two dimensions, and researchers are now finding that extending the lessons of that situation to the real world is full of challenges and pitfalls. In a pair of studies recently published in Nature and Nature Neuroscience, scientists working with bats and rats showed — to their surprise — that the brain encodes 3D spaces very differently from 2D ones, employing a mechanism that they are still struggling to describe and understand.
“We expected something else entirely,” said Nachum Ulanovsky, a neurobiologist at the Weizmann Institute of Science in Israel who led the work in Nature and has studied neural representations of 3D spaces for more than 10 years. “We had to reboot our thinking.”
The findings suggest that neuroscientists might need to reconsider what they thought they knew about how the brain encodes natural environments and how animals navigate those spaces. The work also hints at the possibility that other cognitive processes, including memory, might operate very differently than researchers have come to believe.
When Grid Cells Go 3D
Decades of work have established that the brain’s navigational system consists of several types of neurons. Place cells in the hippocampus fire when an animal passes through a known location in its environment. Head direction cells fire when the animal’s head points in a certain direction, like north or south. Border cells fire at specific distances from a boundary.
But most intriguing are grid cells, which are found in a brain region near the hippocampus, called the entorhinal cortex, that plays an important role in both spatial navigation and memory. When an animal is navigating a two-dimensional space — a flat room or maze, for example — these neurons fire as it moves through different locations. The locations that make any one grid cell fire are arranged like the vertices of a periodic hexagonal lattice tiling the floor. Different grid cells have hexagonal firing patterns with different spatial scales and offsets, allowing them to cover the entire 2D plane.
Because of the striking symmetry, regularity and consistency of their activity, grid cells are often thought of as an elegant, fixed and seemingly limitless coordinate system that enables an animal to track precise distances and directions as it moves. In recent years, researchers have reported evidence that the cells might be using that hexagonal code to represent not just physical spaces, but abstract cognitive spaces as well.
But since all of the experiments were conducted in 2D environments, it hasn’t been clear how grid cells might represent environments of three dimensions (or higher, in the case of cognitive spaces).
Kate Jeffery, a behavioral neuroscientist at University College London, has — like Ulanovsky — sought to answer that question for more than a decade. To get her rats to explore the vertical dimension and measure their neural representations, she and her colleagues built a veritable playground for them, gradually adding ramps and spiral staircases, climbing walls and jungle gyms. Watching the animals roam through those contraptions, Jeffery has looked for clues into how grid cells might extend their regular patterns into 3D space.
Theoretically, if the system simply generalized its 2D optimal packing to the third dimension, researchers would expect to see grid cells fire in spherical patches, neatly arranged in a hexagonal 3D lattice structure, much like a stack of oranges in a grocery store. But there had already been hints that something more complex was going on — that grid patterns weren’t always so perfectly organized and symmetrical even in just two dimensions. Researchers had seen, for instance, that changing the geometry of a room could push and pull at the hexagonal grids, distorting their activity and rigid periodicity. The grids also seemed to get warped at locations of importance to the rats, or at places where rewards were present.
Still, it seemed possible that those observations were just deviations within the same hexagonal framework. But when researchers were finally able to record from grid cells in animals navigating 3D spaces, the findings got “much more dramatic,” Ulanovsky said — seeming to demonstrate not just deviations from the framework, but departures from it.
After years spent getting the technology and experimental setup right — which included building a latticelike climbing frame for the rats and setting up wireless recording and three-dimensional tracking systems — Jeffery and her colleagues were finally able to take a look at grid cell activity in the animals’ entorhinal cortex during 3D navigation.
To their surprise, the hexagonal patterns that defined the cells’ behavior in 2D were gone entirely: The researchers couldn’t find even traces of that global order. Instead, the clumps of grid cell activity seemed to be distributed throughout the three-dimensional space at random. “Some properties were preserved,” Jeffery said, “but the most visually striking property of grid cells was not.”
Meanwhile, Ulanovsky was finding something similar in Egyptian fruit bats as they flew around a large room. In fact, when he and his team started recording from grid cells nearly 10 years ago, it was difficult for them to make sense of what they were seeing. “That took two or three years, just to get to this point where we were actually starting to think along the right tracks,” Ulanovsky said.
As in Jeffery’s rats, the bats’ grid cells did not seem to fire in a three-dimensional hexagonal arrangement. In fact, exhaustive analyses showed that there wasn’t any regular global structure to the cellular activity whatsoever.
But the grid cells’ firing wasn’t entirely random either. Instead, there was local order: For each grid cell, the places where it fired weren’t arranged in a perfect periodic lattice, but the distances between them were too regular to be merely a matter of chance. Rather than the neat stack of oranges, the researchers were seeing something similar but less orderly, more like marbles filling a box. “They’re always stuck in some local minimum, such that there is not a lattice,” Ulanovsky said. “On the other hand, the local distances there are fixed, because all the [marbles] are sort of touching their neighbors.”
“This pattern that everybody loves to see and has spawned numerous theoretical examinations just wasn’t there,” Jeffery said. “Maybe the regularity of the pattern is not the important thing about grid cells, even though it’s the most interesting thing to us.”
Enthralled by the Elegance
The hexagonal periodicity of grid cells in two dimensions “has been a really beautiful thing to explore,” said Loren Frank, a neuroscientist at the University of California, San Francisco who was not involved in either study. “And this usually happens in science: When you have something beautiful, people attribute a great deal of importance and centrality to it.”
But the lack of a crystalline structure of grid cell firing in three dimensions “forces you to kind of step back a bit and say, OK, have I been imbuing this particular network with too much capability?” he said.
For one thing, the results suggest that the brain’s intrinsic map of space isn’t as precisely metric as some models would have it — at least not in natural environments, where obstacles, landmarks and other complexities affect the landscape. Rather than plotting out exact geometric relationships between reference points, the mental map might make broader connections — “a loose kind of metric to be imposed upon the world,” Jeffery said. From that, “we can build up topological relationships and adjacency relationships and so on.” Frank likens it to having a map of a city’s subway system, which gives a sense of connectivity and an idea of relative but not entirely precise distances, rather than having an actual GPS.
Samuel Velasco/Quanta Magazine
That’s sure to affect how scientists think about path integration, an animal’s ability to tell precisely where it is in space in relation to its starting location without the aid of external cues — an ability often attributed to grid cells. Path integration is also thought to enable animals to compute novel shortcuts, find their way home across long distances, and more. But those hypotheses all assume that grid cells map landscapes with perfect periodicity, Ulanovsky notes. “If it’s not perfect, then this entire idea falls apart … and you can’t encode your position robustly anymore using the current models of grid cells,” he said.
At the very least, then, the new results raise questions about proposed grid-based mechanisms of distance estimation and path integration. “We need to consider how this works without strict hexagonal periodicity, or rethink what function grid cells perform altogether,” said Roddy Grieves, a postdoctoral researcher at Dartmouth College who previously worked in Jeffery’s lab, and the first author of their recent paper.
Some models depicting how animals navigate toward goals and across long distances might also need updating. Ila Fiete, a neurobiologist at the McGovern Institute for Brain Research at the Massachusetts Institute of Technology who has done theoretical modeling work on grid codes, speculates that the lack of global structure in three-dimensional grid cell activity could mean that the brain represents higher dimensions very differently than it does flat surfaces. “When you’re in 3D and higher dimensions, maybe we don’t create a continuous, seamless representation of the whole space,” she said. “Maybe the brain just uses a completely different strategy.”
Moving Past the Grid
Hypotheses about grid cell activity and its associated functions generally involve “continuous attractor” models in which each grid cell seeks to activate while suppressing its neighbors. In 2D, that leads to the observed hexagonal pattern of patches of local excitation surrounded by disks of inhibition. But those models don’t predict the breakdown of periodicity in three dimensions. They “are quite rigid in how the grid cells are networked and what arrangements their firing fields can take,” Grieves said. “Our 3D data, in combination with the data from flying bats, casts a large doubt on these models.”
Most scientists think the models can still work — but that they’ll need to be adapted to fit the new three-dimensional observations. Ulanovsky’s team has proposed some additional dynamics to guide that adaptation, and they are now collaborating with theoreticians on a new model in which global hexagonal order emerges in 2D but not 3D.
Meanwhile, to rescue the 2D attractor framework, Fiete, her postdoc Mirko Klukas and their colleagues have proposed a novel model — one for which Fiete sees evidence in some of Ulanovsky’s and Jeffery’s findings. Even before the new results were published, Fiete was skeptical of models that generalized 2D attractor states to three dimensions because they were so biologically costly: Getting nice patterns in 3D, according to her calculations, would require far more grid cells than the entorhinal cortex possesses. Moreover, the construction of a 3D grid would require very different connectivity from a 2D one.
She and Klukas came up with an alternative idea akin to reconstructing a 3D statue from a bunch of photographs taken from different vantage points. Certain sets of grid cells act as a two-dimensional slice of the 3D space. Other sets of grid cells do the same thing, but at a different angle. Together, they form several intersecting columns of grid activity within the entorhinal cortex, and other cells combine those responses — which yields local but not global structure, just as Ulanovsky’s group found.
This model has the advantage of continuing to build on classical theories of attractor networks, path integration and so on. “You just reuse the exact same network, same connectivity, same everything,” Fiete said. “You don’t redo anything. There’s no overhead cost, and you can still represent 3D” — or, according to the model, 4D or 5D or higher.
Other scientists think something else is going on entirely. Regardless, “I am excited to see the next generation of models born out of this,” Grieves said.
And the main takeaway remains true: that “in nature, probably, the pattern doesn’t crystallize very neatly most of the time,” Jeffery said. “I don’t think it’s a natural state for a grid cell.”
“We’ve been a bit captivated by the spatial regularity that we see in grid cells, but that’s just a side issue, really,” she added. “It’s not the most interesting thing about them.”
Perfect Regularity Matters
But if global regularity isn’t the common defining feature of grid cells across different circumstances, scientists have wide-ranging opinions about what is. Ulanovsky, for instance, thinks it’s the characteristic distances between the cells’ firing fields that his team observed. Jeffery thinks it’s the discrete way the cells fire: Even if it’s not perfectly periodic, it could still allow the brain to keep spatial (and perhaps more abstract) representations separate. Fiete emphasizes the ability of grid cells to integrate information about movement and velocity.
Edvard Moser, a neuroscientist at the Norwegian University of Science and Technology and one of the Nobel laureate discoverers of grid cells, thinks their global order and periodicity is still very much what defines them. He and his colleagues have recently shown that even grid cells that seem to fire in distorted, nonperiodic patterns in 2D maintain the same correlations with other grid cells across different environments and brain states, preserving an intrinsic grid.
“I think that the internal structure activity is always precise,” Moser said. “It is something about the mapping to the environment that is not so precise.”
Similarly, Lisa Giocomo, a neuroscientist at Stanford University, thinks that the breakdown of long-range structure in grid cell activity suggests that the cells might be encoding variables other than spatial position, such as a visual cue or the position of the animal’s eye as it surveys the environment. “If you knew what that latent variable was, you might see more structure,” she said.
In fact, the results suggest not only that grid cells might be encoding additional, non-spatial variables, but that they might also be playing a greater role in non-spatial processes — particularly memory. Usually, memory is the purview of the hippocampus, which bundles streams of information from various brain regions to build representations of past experiences and general knowledge. One of those streams of information, perhaps providing a spatial component for memories, comes from the entorhinal cortex and its grid cells. “There is a division of labor,” said Federico Stella, a neuroscientist at the Donders Institute for Brain, Cognition and Behavior in the Netherlands.
But “if these grid cells are not so perfect … they are muddying the waters,” he added. That’s why Stella favors a different interpretation of Ulanovsky and Jeffery’s findings: that grid cells might play a more integral role in memory formation, processing and consolidation than they’re typically given credit for. “One can think of the medial entorhinal cortex as a memory system of its own,” he said.
That opens up the possibility that other brain regions could be processing memories in parallel as well — that the flow of such information is complex and might involve other types of neurons that haven’t gotten as much attention as grid cells. It also implies that other memory processes, including replay and reactivation in the hippocampus, might need to be understood in the context of the entorhinal cortex and grid cells too.
Moving away from thinking about grid cells’ periodic hexagonality could lead to many more important insights. But while “it’s true that our fixation on looking for perfect regularity certainly could have led us to miss some things,” Fiete said, “I think that the periodicity of the grid cell response has been a gift.” It allowed researchers to constrain their models and guide the search for potential mechanisms and functions.
And “it really seems to suggest that 2D is pretty special, doesn’t it?” Fiete added. “It’s somehow privileged.” That could be because, even when animals navigate three-dimensional space, they’re mostly sticking to something close to a 2D plane: Even bats and fish have preferred heights or depths at which they move. The 2D findings might also offer a glimpse into the grid system’s origins — into what parts evolved first, and how the same system was then co-opted to form other representations, with modifications.
“It was very important to first find out that these cells can get to be very perfect,” Stella said. Now, though, the point is to ask, “What can these cells do anyway, even without this level of symmetry?”
The new work in 3D has “certainly not made life conceptually much easier,” Fiete said — but for her, that’s where the fun is. “The brain has so many surprises for us. Here’s this system that you kind of understand, and it’s orderly — and the brain just throws a curveball.”
Editor’s note: Ila Fiete, Loren Frank and Lisa Giocomo have received funding from the Simons Foundation, which also funds Quanta as an editorially independent science magazine. Funding decisions do not affect editorial coverage.