Astronomers Reimagine the Making of the Planets
![An array of images of protoplanetary disks with bright suns at the centers surrounded by rings, arcs, filaments and spirals.]](https://www.quantamagazine.org/wp-content/uploads/2022/06/Planet-Formation_2880x1620_Lede.jpg)
Newborn star systems imaged by the ALMA telescope, featuring protoplanetary disks with rings, arcs, filaments and spirals, are among the observations changing the theory of how planets are made.
ALMA (ESO/NAOJ/NRAO), S. Andrews et al.; N. Lira
Introduction
Start at the center, with the sun. Our middle-aged star may be more placid than most, but it is otherwise unremarkable. Its planets, however, are another story.
First, Mercury: More charred innards than fully fledged planet, it probably lost its outer layers in a traumatic collision long ago. Next come Venus and Earth, twins in some respects, though oddly only one is fertile. Then there’s Mars, another wee world, one that, unlike Mercury, never lost layers; it just stopped growing. Following Mars, we have a wide ring of leftover rocks, and then things shift. Suddenly there is Jupiter, so big it’s practically a half-baked sun, containing the vast majority of the material left over from our star’s creation. Past that are three more enormous worlds — Saturn, Uranus, and Neptune — forged of gas and ice. The four gas giants have almost nothing in common with the four rocky planets, despite forming at roughly the same time, from the same stuff, around the same star. The solar system’s eight planets present a puzzle: Why these?
Now look out past the sun, way beyond. Most of the stars harbor planets of their own. Astronomers have spotted thousands of these distant star-and-planet systems. But strangely, they have so far found none that remotely resemble ours. So the puzzle has grown harder: Why these, and why those?
The swelling catalog of extrasolar planets, along with observations of distant, dusty planet nurseries and even new data from our own solar system, no longer matches classic theories about how planets are made. Planetary scientists, forced to abandon decades-old models, now realize there may not be a grand unified theory of world-making — no single story that explains every planet around every star, or even the wildly divergent orbs orbiting our sun. “The laws of physics are the same everywhere, but the process of building planets is sufficiently complicated that the system becomes chaotic,” said Alessandro Morbidelli, a leading figure in planetary formation and migration theories and an astronomer at the Côte d’Azur Observatory in Nice, France.
Still, the findings are animating new research. Amid the chaos of world-building, patterns have emerged, leading astronomers toward powerful new ideas. Teams of researchers are working out the rules of dust and pebble assembly and how planets move once they coalesce. Fierce debate rages over the timing of each step, and over which factors determine a budding planet’s destiny. At the nexus of these debates are some of the oldest questions humans have asked ourselves: How did we get here? Is there anywhere else like here?
A Star and Its Acolytes Are Born
Astronomers have understood the basic outlines of the solar system’s origins for nearly 300 years. The German philosopher Immanuel Kant, who like many Enlightenment thinkers dabbled in astronomy, published a theory in 1755 that remains pretty much correct. “All the matter making up the spheres belonging to our solar system, all the planets and comets, at the origin of all things was broken down into its elementary basic material,” he wrote.
Indeed, we come from a diffuse cloud of gas and dust. Four and a half billion years ago, probably nudged by a passing star or by the shock wave of a supernova, the cloud collapsed under its own gravity to form a new star. It’s how things went down afterward that we don’t really understand.
Once the sun ignited, surplus gas swirled around it. Eventually, the planets formed there. The classical model that explained this, known as the minimum-mass solar nebula, envisioned a basic “protoplanetary disk” filled with just enough hydrogen, helium and heavier elements to make the observed planets and asteroid belts. The model, which dates to 1977, assumed planets formed where we see them today, beginning as small “planetesimals,” then incorporating all the material in their area like locusts consuming every leaf in a field.
“The model was just somehow making this assumption that the solar disk was filled with planetesimals,” said Joanna Drążkowska, an astrophysicist at the Ludwig Maximilian University of Munich and author of a recent review chapter on the field. “People were not considering any smaller objects — no dust, no pebbles.”
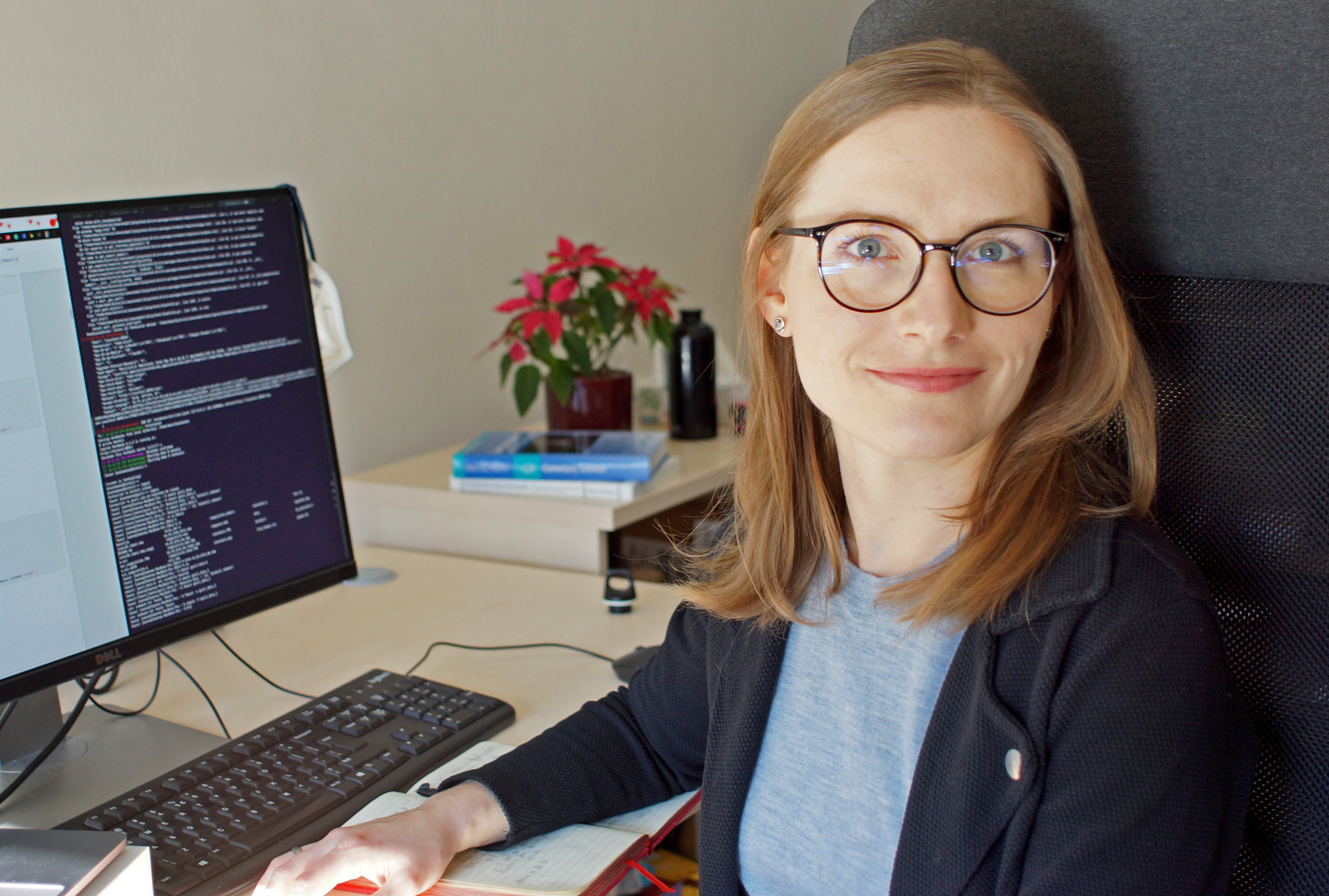
Joanna Drążkowska, an astrophysicist at Ludwig Maximilian University of Munich, uses computer simulations to explore the formation of planetesimals and planets out of dust grains swirling around young stars.
Wieńczysław Bykowski
Astronomers vaguely reasoned that planetesimals arose because dust grains pushed around by the gas would have drifted into piles, the way wind sculpts sand dunes. The classical model had planetesimals randomly strewn throughout the solar nebula, with a statistical distribution of sizes following what physicists call a power law, meaning there are more small ones than big ones. “Just a few years ago, everybody was assuming the planetesimals were distributed in a power law throughout the nebula,” said Morbidelli, “but now we know it is not the case.”
The change came courtesy of a handful of silver parabolas in Chile’s Atacama Desert. The Atacama Large Millimeter/submillimeter Array (ALMA) is designed to detect light from cool, millimeter-size objects, such as dust grains around newborn stars. Starting in 2013, ALMA captured stunning images of neatly sculpted infant star systems, with putative planets embedded in the hazy disks around the new stars.
Astronomers previously imagined these disks as smooth halos that grew more diffuse as they extended outward, away from the star. But ALMA showed disks with deep, dark gaps, like the rings of Saturn; others with arcs and filaments; and some containing spirals, like miniature galaxies. “ALMA changed the field completely,” said David Nesvorny, an astronomer at the Southwest Research Institute in Boulder, Colorado.
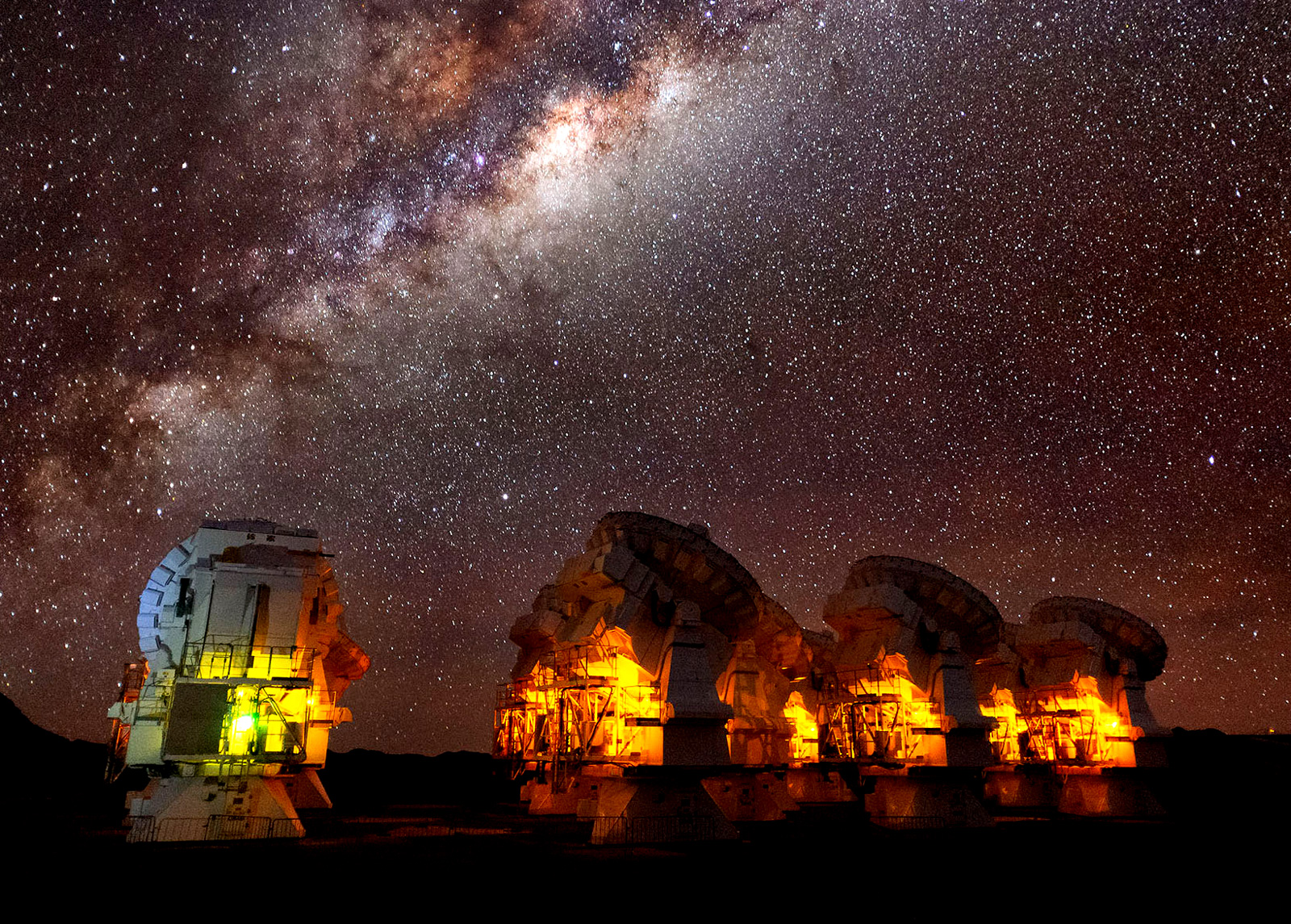
The Atacama Large Millimeter/submillimeter Array (ALMA) in Chile’s Atacama Desert observes distant, dusty planet nurseries.
Sergio Otarola (ESO/NAOJ/NRAO)
ALMA disproved the classical model of planetary formation. “We have to now reject it and start thinking about completely different models,” Drążkowska said. The observations showed that, rather than being smoothly dispersed through the disk, dust collects in particular places, as dust likes to do, and that is where the earliest planet embryos are made. Some dust, for instance, probably clumps together at the “snow line,” the distance from the star where water freezes. Recently, Morbidelli and Konstantin Batygin, an astronomer at the California Institute of Technology, argued that dust also clumps at a condensation line where silicates form droplets instead of vapor. These condensation lines probably cause traffic jams, curbing the rate at which dust falls toward the star and allowing it to pile up.
“It’s a new paradigm,” Morbidelli said.
From Dust to Planets
Even before ALMA showed where dust likes to accrue, astronomers were struggling to understand how it could pile up quickly enough to form a planet — especially a giant one. The gas surrounding the infant sun would have dissipated within about 10 million years, which means Jupiter would have had to collect most of it within that time frame. That means dust must have formed Jupiter’s core very soon after the sun ignited. The Juno mission to Jupiter showed that the giant planet probably has a fluffy core, suggesting it formed fast. But how?
The problem, apparent to astronomers since about the year 2000, is that turbulence, gas pressure, heat, magnetic fields and other factors would prevent dust from orbiting the sun in neat paths, or from drifting into big piles. Moreover, any big clumps would likely be drawn into the sun by gravity.
In 2005, Andrew Youdin and Jeremy Goodman, then of Princeton University, published a new theory for dust clumps that went part of the way toward a solution. A few years after the sun ignited, they argued, gas flowing around the star formed headwinds that forced dust to gather in clumps, and kept the clumps from falling into the star. As the primordial dust bunnies grew bigger and denser, eventually they collapsed under their own gravity into compact objects. This idea, called streaming instability, is now a widely accepted model for how millimeter-size dust grains can quickly turn into large rocks. The mechanism can form planetesimals about 100 kilometers across, which then merge with one another in collisions.
But astronomers still struggled to explain the creation of much bigger worlds like Jupiter.
In 2012, Anders Johansen and Michiel Lambrechts, both at Lund University in Sweden, proposed a variation on planet growth dubbed pebble accretion. According to their idea, planet embryos the size of the dwarf planet Ceres that arise through the streaming instability quickly grow much bigger. Gravity and drag in the circumstellar disk would cause dust grains and pebbles to spiral onto these objects, which would grow apace, like a snowball rolling downhill.
Merrill Sherman/Quanta Magazine
Pebble accretion is now a favored theory for how gas giant cores are made, and many astronomers argue it may be taking place in those ALMA images, allowing giant planets to form in the first few million years after a star is born. But the theory’s relevance to the small, terrestrial planets near the sun is controversial. Johansen, Lambrechts and five coauthors published research last year showing how inward-drifting pebbles could have fed the growth of Venus, Earth, Mars and Theia — a since-obliterated world that collided with Earth, ultimately creating the moon. But problems remain. Pebble accretion does not say much about giant impacts like the Earth-Theia crash, which were vital processes in shaping the terrestrial planets, said Miki Nakajima, an astronomer at the University of Rochester. “Even though pebble accretion is very efficient and is a great way to avoid issues with the classical model, it doesn’t seem to be the only way” to make planets, she said.
Morbidelli rejects the idea of pebbles forming rocky worlds, in part because geochemical samples suggest that Earth formed over a long period, and because meteorites come from rocks of widely varying ages. “It’s a matter of location,” he said. “Processes are different depending on the environment. Why not, right? I think that makes qualitative sense.”
Research papers appear nearly every week about the early stages of planet growth, with astronomers arguing about the precise condensation points in the solar nebula; whether planetesimals start out with rings that fall onto the planets; when the streaming instability kicks in; and when pebble accretion does, and where. People can’t agree on how Earth was built, let alone terrestrial planets around distant stars.
Planets on the Move
The five wanderers of the night sky — Mercury, Venus, Mars, Jupiter and Saturn — were the only known worlds besides this one for most of human history. Twenty-six years after Kant published his nebular hypothesis, William Herschel found another, fainter wanderer and named it Uranus. Then Johann Gottfried Galle spotted Neptune in 1846. Then, a century and a half later, the number of known planets suddenly shot up.
It started in 1995, when Didier Queloz and Michel Mayor of the University of Geneva pointed a telescope at a sunlike star called 51 Pegasi and noticed it wobbling. They inferred that it’s being tugged at by a giant planet closer to it than Mercury is to our sun. Soon, more of these shocking “hot Jupiters” were seen orbiting other stars.
The exoplanet hunt took off after the Kepler space telescope opened its lens in 2009. We now know the cosmos is peppered with planets; nearly every star has at least one, and probably more. Most seem to have planets we lack, however: hot Jupiters, for instance, as well as a class of midsize worlds that are bigger than Earth but smaller than Neptune, uncreatively nicknamed “super-Earths” or “sub-Neptunes.” No star systems have been found that resemble ours, with its four little rocky planets near the sun and four gas giants orbiting far away. “That does seem to be something that is unique to our solar system that is unusual,” said Seth Jacobson, an astronomer at Michigan State University.
Enter the Nice model, an idea that may be able to unify the radically different planetary architectures. In the 1970s, geochemical analysis of the rocks collected by Apollo astronauts suggested that the moon was battered by asteroids 3.9 billion years ago — a putative event known as the Late Heavy Bombardment. In 2005, inspired by this evidence, Morbidelli and colleagues in Nice argued that Jupiter, Saturn, Uranus and Neptune did not form in their present locations, as the earliest solar nebula model held, but instead moved around 3.9 billion years ago. In the Nice model (as the theory became known), the giant planets changed their orbits wildly at that time, which sent an asteroid deluge toward the inner planets.
Merrill Sherman/Quanta Magazine
The evidence for the Late Heavy Bombardment is no longer considered convincing, but the Nice model has stuck. Morbidelli, Nesvorny and others now conclude that the giants probably migrated even earlier in their history, and that — in an orbital pattern dubbed the Grand Tack — Saturn’s gravity probably stopped Jupiter from moving all the way in toward the sun, where hot Jupiters are often found.
In other words, we might have gotten lucky in our solar system, with multiple giant planets keeping each other in check, so that none swung sunward and destroyed the rocky planets.
“Unless there is something to arrest that process, we would end up with giant planets mostly close to their host stars,” said Jonathan Lunine, an astronomer at Cornell University. “Is inward migration really a necessary outcome of the growth of an isolated giant planet? What are the combinations of multiple giant planets that could arrest that migration? It’s a great problem.”
There is also, according to Morbidelli, “a fierce debate about the timing” of the giant-planet migration — and a possibility that it actually helped grow the rocky planets rather than threatening to destroy them after they grew. Morbidelli just launched a five-year project to study whether an unstable orbital configuration soon after the sun’s formation might have helped stir up rocky remains, coaxing the terrestrial worlds into being.
The upshot is that many researchers now think giant planets and their migrations might dramatically affect the fates of their rocky brethren, in this solar system and others. Jupiter-size worlds might help move asteroids around, or they could limit the number of terrestrial worlds that form. This is a leading hypothesis for explaining the small stature of Mars: It would have grown bigger, maybe to Earth size, but Jupiter’s gravitational influence cut off the supply of material. Many stars studied by the Kepler telescope harbor super-Earths in close orbits, and scientists are split on whether those are likelier to be accompanied by giant planets farther out. Teams have convincingly shown both correlations and anti-correlations between the two exoplanet types, said Rachel Fernandes, a graduate student at the University of Arizona; this indicates that there’s not enough data yet to be sure. “That’s one of those things that is really fun at conferences,” she said. “You’re like, ‘Yeah, yell at each other, but which science is better?’ You don’t know.”
Rebounding Planets
Recently, Jacobson came up with a new model that radically changes the timing of the Nice model migration. In a paper published in April in Nature, he, Beibei Liu of Zhejiang University in China and Sean Raymond of the University of Bordeaux in France argued that gas flow dynamics may have caused the giant planets to migrate only a few million years after they formed — 100 times earlier than in the original Nice model and probably before Earth itself arose.
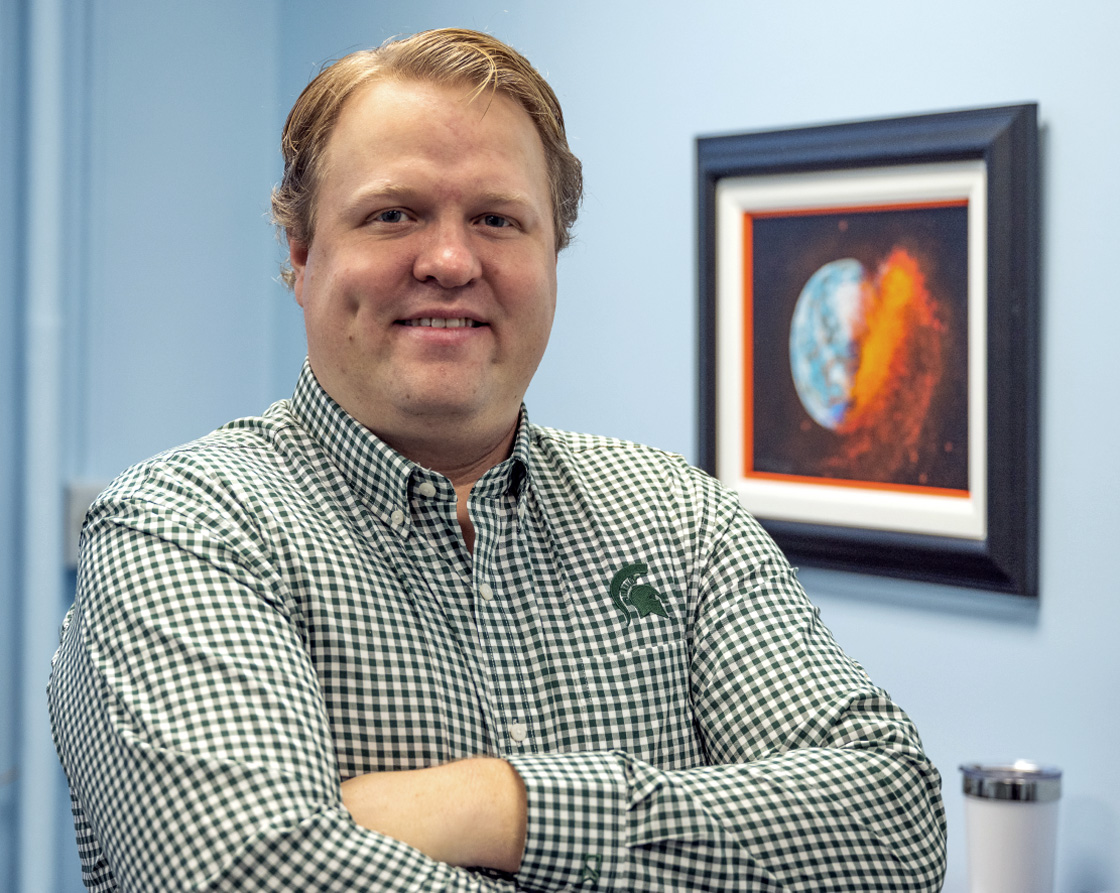
Seth Jacobson, a planetary scientist at Michigan State University, and collaborators recently identified a rebound mechanism by which giant planets that have moved close to their stars might then move back out.
Credit: Derrick Turner, University Communications, Michigan State University
In the new model, the planets “rebounded,” moving in and then back out as the sun warmed up the gas in the disk and blew it off into oblivion. This rebound would have happened because, when a baby giant planet is bathed in a warm disk of gas, it feels an inward pull toward dense gas closer to the star and an outward pull from gas farther out. The inward pull is greater, so the baby planet gradually moves closer to its star. But after the gas begins to evaporate, a few million years after the star’s birth, the balance changes. More gas remains on the far side of the planet relative to the star, so the planet is dragged back out.
The rebound “is a pretty significant shock to the system. It can destabilize a very nice arrangement,” Jacobson said. “But this does a great job of explaining [features] of the giant planets in terms of their inclination and eccentricity.” It also tracks with evidence that hot Jupiters seen in other star systems are on unstable orbits — perhaps bound for a rebound.
Between condensation lines, pebbles, migrations and rebounds, a complex story is taking shape. Still, for now, some answers may be in hiding. Most of the planet-finding observatories use search methods that turn up planets that orbit close to their host stars. Lunine said he would like to see planet hunters use astrometry, or the measurement of stars’ movements through space, which could reveal distantly orbiting worlds. But he and others are most excited for the Nancy Grace Roman Space Telescope, set to launch in 2027. Roman will use microlensing, measuring how the light from a background star is warped by the gravity of a foreground star and its planets. That will let the telescope capture planets with orbital distances between Earth’s and Saturn’s — a “sweet spot,” Lunine said.
Nesvorny said modelers will continue tinkering with code and trying to understand the finer points of particle distributions, ice lines, condensation points and other chemistry that may play a role in where planetesimals coalesce. “It will take the next few decades to understand that in detail,” he said.
Time is the essence of the problem. Human curiosity may be unbounded, but our lives are short, and the birth of planets lasts eons. Instead of watching the process unfold, we have only snapshots from different points.
Batygin, the Caltech astronomer, compared the painstaking effort to reverse-engineer planets to trying to model an animal, even a simple one. “An ant is way more complicated than a star,” Batygin said. “You can perfectly well imagine writing a code that captures a star in pretty good detail,” whereas “you could never model the physics and chemistry of an ant and hope to capture the whole thing. … In planet formation, we are somewhere between an ant and a star.”