How Do Merging Supermassive Black Holes Pass the Final Parsec?
Introduction
Galaxies have been merging into ever-bigger structures over the course of cosmic history. When galaxies merge, the supermassive black holes that sit in their centers must eventually merge, too, forming an even more gargantuan black hole.
For decades, though, a question has vexed astrophysicists: How can supermassive black holes get close enough to spiral together and coalesce? In calculations, when the converging holes reach the so-called final parsec — a distance of about one parsec, or 3.26 light-years — their progress stalls. They should essentially orbit each other indefinitely.
“It was thought in-spiral times could be as high as … the age of the universe,” said Stephen Taylor, an astrophysicist at Vanderbilt University. “People were concerned that you might not get any merging black holes.”
Evidence has accrued that they do merge. Last year, observations of the subtle movements of pulsating stars known as a pulsar timing array revealed a background hum of gravitational waves in the universe — ripples in the fabric of space-time. These gravitational waves most likely come from tightly orbiting supermassive black holes within a parsec of each other that are close to merging. “This was our first evidence that black hole binaries do overcome the final-parsec problem,” said Laura Blecha, an astrophysicist at the University of Florida.
So how do they do it?
Astrophysicists have a new suggestion: Dark matter could sap angular momentum from the two black holes and nudge them closer.
Dark matter is the term for the undiscovered 85% of matter in the universe. We can see its gravitational effects on galaxies and cosmic structure, but at the moment we can’t work out what it is. The simplest hypothetical particles that could comprise this invisible form of matter would be no help in facilitating black hole mergers. But this summer, a group of physicists in Canada argued that something more complex called self-interacting dark matter could. These particles could drag on the supermassive black holes enough to drop them within a parsec of each other. If this explanation is right, it will “tell you that dark matter is not as simple as we thought,” said Gonzalo Alonso-Álvarez, a theoretical physicist at the University of Toronto and one of the authors.
Then in September, a separate group of physicists pointed out that another dark matter candidate sometimes called fuzzy dark matter could do the trick as well.
More prosaic solutions to the puzzle have also been floated over the years. Amid this slew of options — some mundane, some exotic — scientists are devising ways to test the possibilities against each other.
“It’s almost taken for granted at this point by most of the community that the final-parsec problem is solved,” said Sean McWilliams, a theoretical astrophysicist at West Virginia University who has studied several solutions to the problem. “The only question is: What’s the most efficient thing that solves it?”
Two to Tango
Small black holes — star-size objects so dense that their gravity traps anything that gets too close, even light — are sprinkled throughout galaxies. They form from the gravitational collapse of individual stars. But the supermassive black holes found in galaxies’ centers, which can be as heavy as billions of suns, are more mysterious and influential. They somehow steer the formation and evolution of the galaxy around them.
When two galaxies merge, gravitational interactions with stars, gas and dark matter cause the two supermassive black holes to slowly fall toward each other. Astrophysicists first described this process, called dynamical friction, in 1980. “This is thought to be the main way black holes get closer,” said Dan Hooper, an astrophysicist at the University of Wisconsin, Madison.
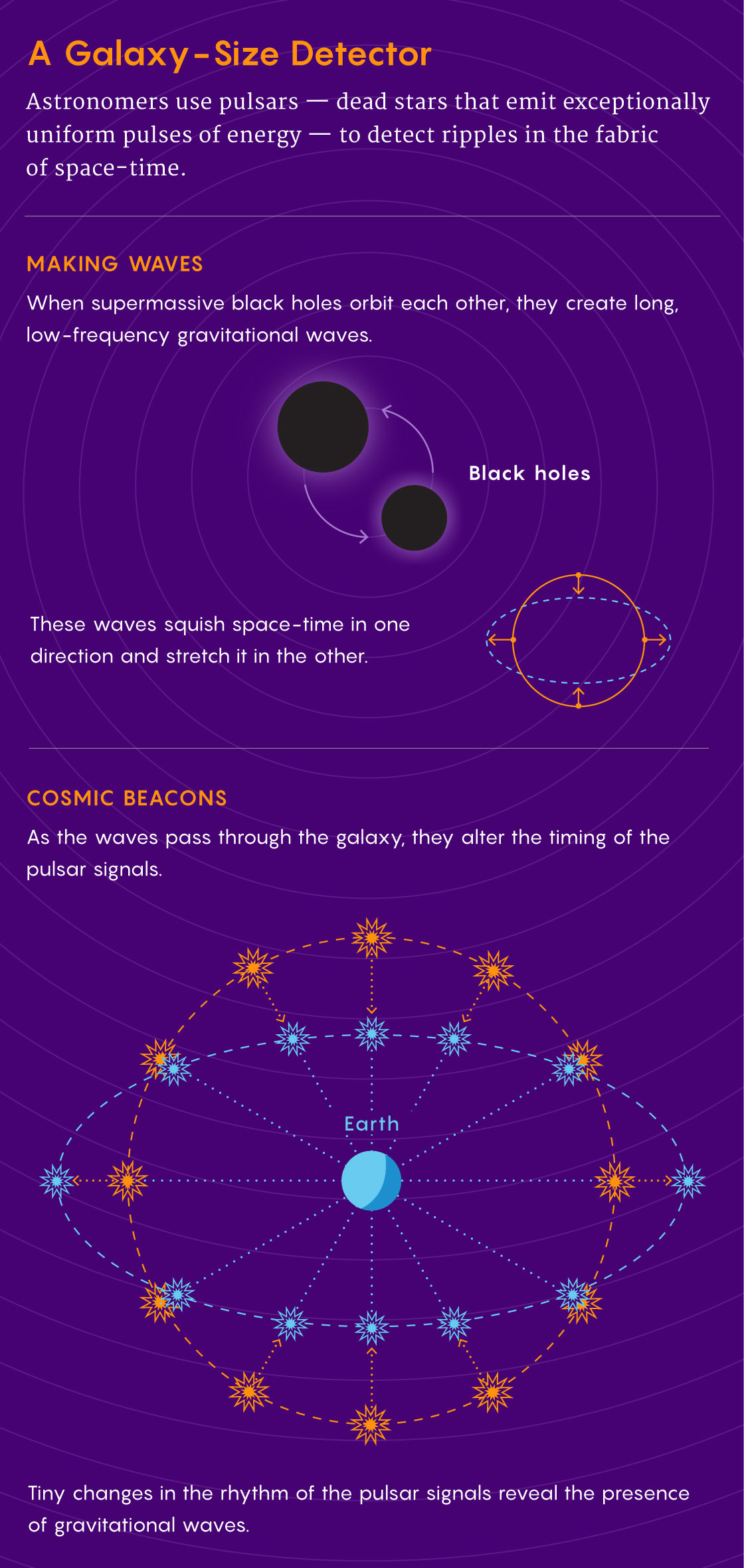
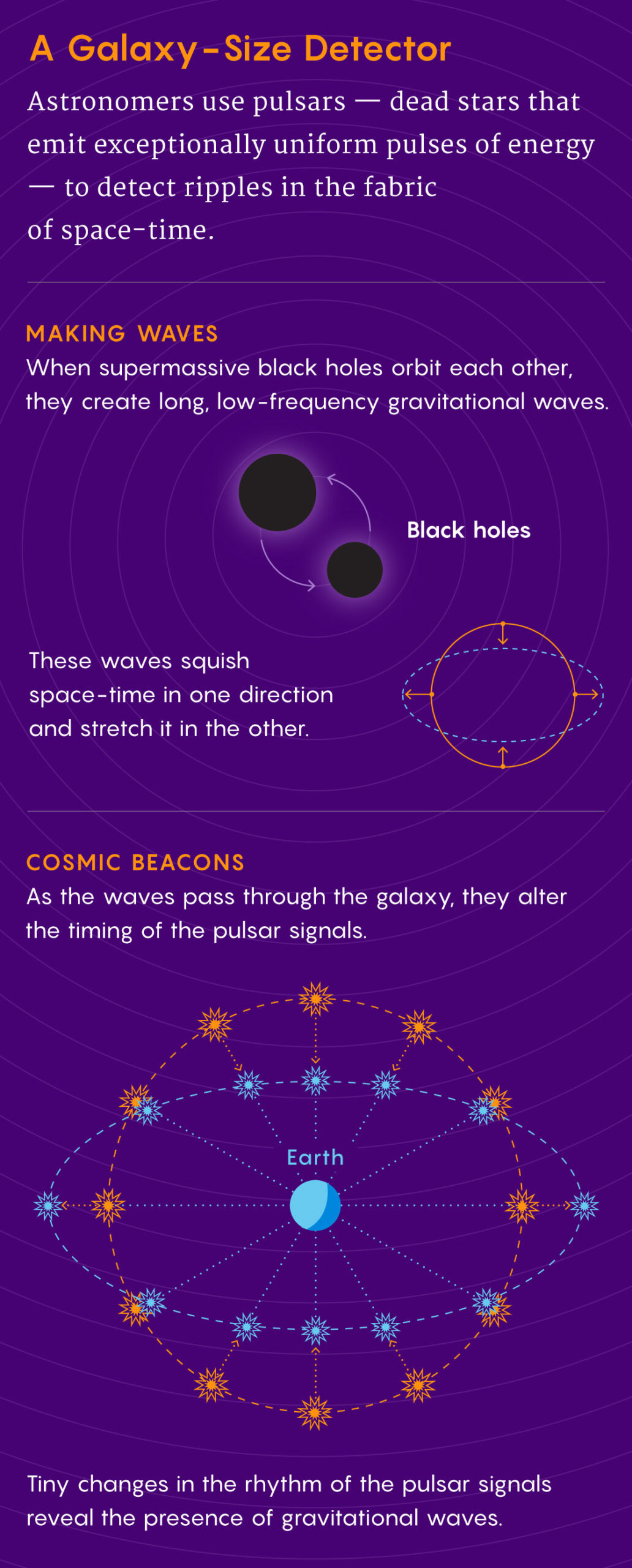
Merrill Sherman for Quanta Magazine
At a certain point, however — technically ranging from a fraction of a parsec to a few parsecs, depending on the black holes’ masses — dynamical friction “turns out to stop being very effective,” Hooper said. Here, at the center of the coalescing galaxies, the two black holes eat material and fling it away, carving out a gap. As a result, the density of stars and gas drops dramatically, leaving the black holes in relatively empty space. Without stuff around them to slow them down, they should then orbit each other almost endlessly.
“Earth is orbiting the sun, and we’re not falling into each other,” Alonso-Álvarez said, and the same should be true for two black holes. “There is a conservation of angular momentum in the orbit that prevents them from falling, unless there’s something that’s extracting this energy.”
Self-interacting dark matter could play this role, as Alonso-Álvarez and colleagues proposed in Physical Review Letters in July. This type differs from so-called cold dark matter — the simplest kind of hypothetical dark matter particles, which would be heavy, slow and inert. Cold dark matter wouldn’t interact with anything except via gravity, so the gravitational influence of the black holes should kick it out of the vicinity well before the black holes reach the final parsec.
Self-interacting dark matter, however, consists of lightweight particles that have at least one force acting between them. Because self-interacting dark matter particles scatter off one another like billiard balls on a table, they would not be as easily dispersed and would instead pull at the black holes’ heels, slowing them down. “It stays there and generates friction,” Alonso-Álvarez said. “It has some sort of viscosity.” That friction could then result in a merger within 100 million years, solving the final-parsec problem.
“Ultralight” or “fuzzy” dark matter would consist of particles with extremely small masses that would come together to form vast waves. These particles would also concentrate in the galactic center and experience friction with the black holes, allowing the fuzzy dark matter to “efficiently carry away their angular momentum and orbital energy,” said Jae-Weon Lee, a cosmologist at Jungwon University in South Korea and a co-author of a September paper in Physics Letters B describing the idea. The black holes would cause this dark matter to vibrate like a bell rather than dispersing.
Occam’s Razor
Not everyone is convinced we need to invoke such exotic physics to explain how supermassive black holes merge. “I wouldn’t say that we need self-interacting dark matter,” said Priyamvada Natarajan, a theoretical astrophysicist at Yale University.
A different possibility is that stars could swing past the merging black holes and remove enough angular momentum to bring them together. Perhaps the stars get randomly slung in the direction of the black holes from elsewhere in the galaxy through interactions with other stars. “If you have a ton of these stars that get close to the central two supermassive black holes, then you can extract more and more angular momentum,” said Fabio Pacucci, a theoretical astrophysicist at Harvard University.
However, modeling has shown that it is difficult to scatter enough stars toward the black holes to solve the final-parsec problem.
Alternatively, each black hole might have a small disk of gas around it, and these disks might draw in material from a wider disk that surrounds the empty region carved out by the holes. “The disks around them are being fed from the wider disk,” Taylor said, and that means, in turn, that their orbital energy can leak into the wider disk. “It seems a very efficient solution,” Natarajan said. “There’s a lot of gas available.”
In January, Blecha and her colleagues investigated the idea that a third black hole in the system could provide a solution. In some cases where two black holes have stalled, another galaxy could begin to merge with the first two, bringing with it an additional black hole. “You can have a strong three-body interaction,” Blecha said. “It can take away energy and greatly decrease the merger timescale.” In some scenarios, the lightest of the three holes is ejected, but in others all three merge.
Tests on the Horizon
The task now is to work out which solution is correct, or if multiple processes are at play.
Alonso-Álvarez hopes to test his idea by seeking a signal of self-interacting dark matter in upcoming pulsar timing array data. Once black holes get closer than the final parsec, they shed angular momentum primarily by emitting gravitational waves. But if self-interacting dark matter is at play, then we should see it sap some of the energy at distances around the parsec limit. This in turn would make for less energetic gravitational waves, Alonso-Álvarez said.
Hai-Bo Yu, a particle physicist at the University of California, Riverside who is a proponent of self-interacting dark matter, said the idea is plausible. “It’s an avenue to look for microscopic features of dark matter from gravitational wave physics,” he said. “I think that’s just fascinating.”
The European Space Agency’s LISA (Laser Interferometer Space Antenna) spacecraft, a gravitational wave observatory that’s set to launch in 2035, might give us even more answers. LISA will pick up the strong gravitational waves emitted by merging supermassive black holes in their final days. “With LISA we will actually see supermassive black holes merging,” Pacucci said. The nature of that signal could reveal “particular traits that show the slowing process,” solving the final-parsec problem.