How Do New Organs Evolve? A Beetle Gland Shows the Way.
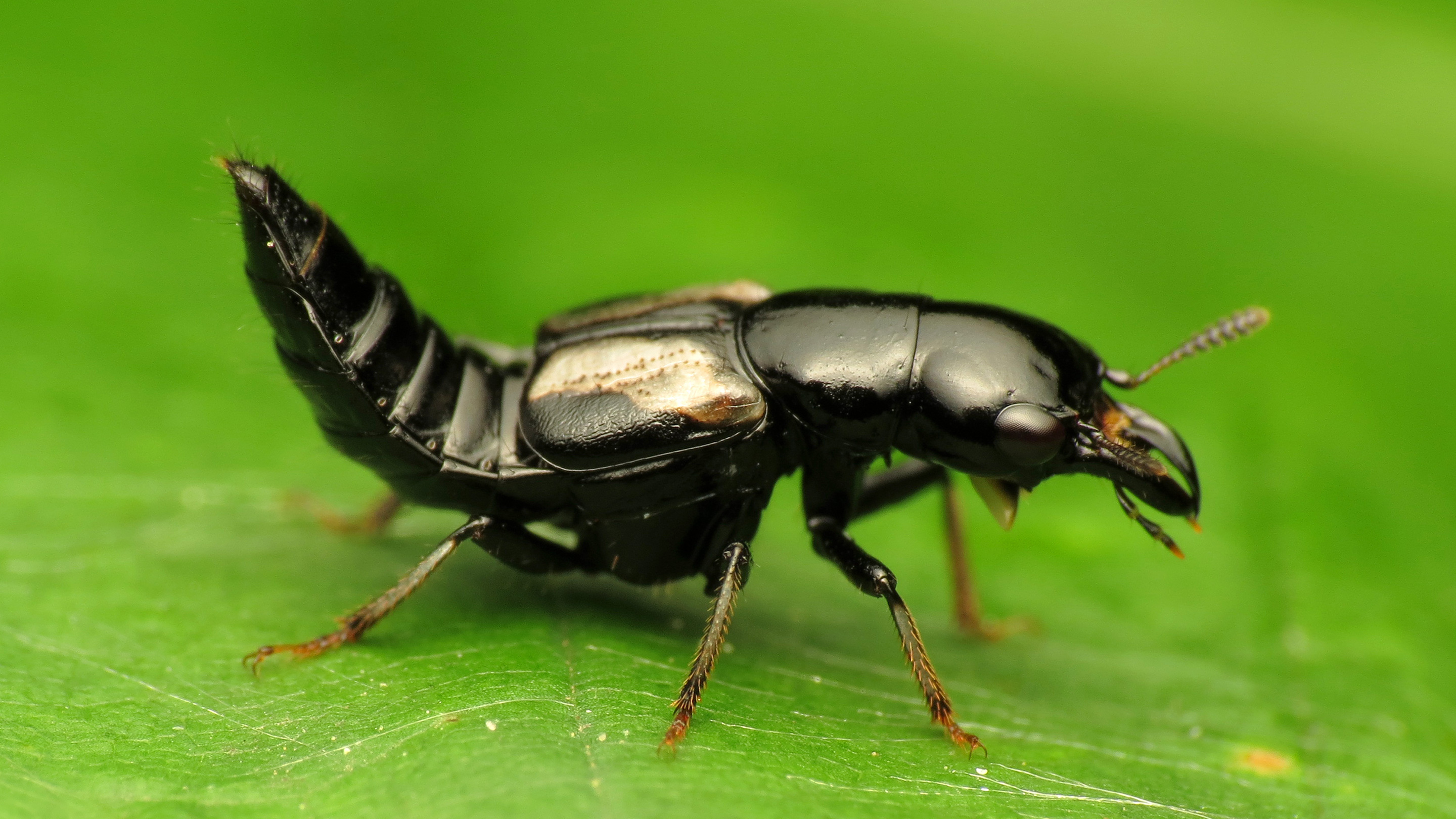
Rove beetles defend themselves against predators by expelling a toxic mixture of chemicals from a unique gland in their abdomen. The origin of that gland holds clues to the evolution of organs in general.
Introduction
The tiny rove beetle, no bigger than a grain of rice, looked dangerously exposed on the bottom of the glass dish in Joe Parker’s laboratory — and that was even before its fellow prisoner, an ant three times as big, advanced on it. The ant’s mandibles gaped open and prepared to seize the beetle. Suddenly, the beetle’s abdomen curved up and something happened, something that sent the ant reeling backward, its legs and antennae flailing. The beetle quickly escaped, taking at least temporary refuge in another part of the chamber.
The beetle’s salvation was a gland just beneath the top back surface of its abdomen that ejected a noxious mix of chemicals into the ant’s face. Parker, an evolutionary biologist at the California Institute of Technology, leads a team that recently retraced the steps that led to the evolution of that simple but novel structure, which is unique to a certain group of beetles. And although the gland may be unique, the insights from that work may illuminate a more universal question: How do new organs evolve?
Whether it’s an eye, a heart or a liver, a new organ evolves when a multicellular organism sets up a division of labor among its cells. But how that specialization evolves is a problem like the “chicken or the egg” riddle: The diverse cells in an organ perform different functions whose benefit may only be apparent in the context of the whole working organ’s purpose, so why did those cells and functions evolve in the first place?
Deepening the mystery, many organs are so ancient that their origins are almost impossible to reconstruct. “It’s very murky,” said Rajendhran Rajakumar, a developmental biologist at the University of Ottawa who works on the evolution of insects. “It’s really hard to pinpoint the transitions that occurred to make something as complex as a whole organ.”
In recent years, however, scientists have begun to make deep inroads into the problem by probing the origins of eyes and other features in various invertebrates. And now the study from Parker and his colleagues identifies what could be important principles guiding the emergence of new organs: first, that changes in one type of cell can open up new opportunities, or niches, for neighboring cells to capitalize on. And second, that once such a partnership is successfully established, the different cell types can become reliant on one another, locked in a cycle of mutual dependence.
The coevolution that the researchers observed in the beetle gland could be “a kind of paradigm for functional evolution of organs in general, through this process of niche creation,” Parker said.
Glands may seem humble in comparison to more elaborate organs, but they have become an important focus for these kinds of studies. Because they have fewer cell types than complex organs, they offer a simpler starting point for the discovery of basic principles. They have typically evolved more recently, which makes their evolution easier to reconstruct. They are also often the sites for remarkable biological innovations, such as new chemical defenses and bioluminescence.
To Leslie Babonis, an evolutionary developmental biologist at Cornell University, it also makes sense that the secretory cells in glands and other tissues could be “hot spots for the evolution of novel function.” By definition, such cells are proficient at concentrating gene products into vesicles and then removing them from the cell body through secretion. “The ability to concentrate gene products — proteins — into a vesicle and secrete it to the outside of the cell is really powerful,” Babonis said. “It allows the cell a lot of control over what’s going on inside of it” while also communicating information and exerting effects outside.
How New Cell Types Evolve
For evidence that glands can be cauldrons for rapid, remarkable evolutionary change, there may be no better place to look than in the large family of insects called rove beetles (Staphylinidae). Of the more than 350,000 known species of beetles, 64,000 are rove beetles. These small, unassuming insects lack the hardened forewings that cover and protect the abdomen of most beetles, so they are flexible when wriggling through the leaf litter they inhabit, but also vulnerable to predators. For protection, many rove beetle lineages independently evolved diverse defense glands that spray noxious chemicals from different points on their abdomen.
To understand how a defense gland evolved, the Caltech researchers focused on the species Dalotia coriaria, in the Aleocharinae subfamily. Its defensive tergal gland is a key innovation that likely explains the evolutionary success of this lineage: With about 17,000 species, the aleocharines are the largest clade of rove beetles.
Led by the postdoctoral fellow Adrian Brückner, Parker’s team began by analyzing the gland’s chemical cocktail. It proved to be a liquid mixture of highly irritating benzoquinones in a solution of long hydrocarbon compounds (alkanes and esters). Further work showed that the two cell types making up the gland were each responsible for ingredients in that mixture. The “solvent cells” that produce the alkanes and esters are part of the soft membrane in the exoskeleton; they form a pouch or reservoir between two of the abdominal segments. Beneath the solvent cells is a set of “gland cells” that make the benzoquinones, which they release into the reservoir through ducts. When the rove beetle lifts its abdomen and bends apart the segments, the reservoir opens and squirts the mixture at ants or other predators.
But when the researchers looked more deeply into how these cells evolved and acquired the ability to make their products, they ran into a series of surprises.
Parker’s team had reason to think that the solvent cells evolved first: The earliest, oldest branch of aleocharines with a tergal gland makes esters, but not benzoquinones. The researchers hypothesized that the alkanes and esters are made by a fatty acid pathway derived from part of the beetle’s core metabolism. When the researchers shut down the synthesis of fatty acids in the cells encasing the gland’s main reservoir, they found that the alkanes and esters had vanished. “So that tells us that that cell type is making the hydrocarbon-type compounds, and it’s doing so via a cell-autonomous fatty acid pathway,” Parker said.
Samuel Velasco/Quanta Magazine; source: Brückner et al.
When the researchers looked at the RNA molecules expressed in individual solvent cells, they discovered that sets of gene transcripts expressed together in the solvent cells were also expressed together in certain other tissues, such as the beetle’s fat body, which is roughly equivalent in function to the mammalian liver.
“The beetle is reusing this ancient fatty acid biosynthesis toolkit, which evolved in the fat body and the pheromone-producing cells,” Parker said — but evolution installed that toolkit in a patch of cuticle and turned it into a chemical secretory reservoir. He calls this phenomenon “transcriptomic hybridization,” because the new solvent cells are in effect a fusion of the gene expression patterns from two ancestral cell types, the cuticle cells and the fat body or pheromonal cells.
“I think they just deploy this set of gene products that have coevolved for millions of years to work very effectively together, stick it in some part of the cuticle, and then they are able to start manufacturing new secreted compounds,” Parker said.
The solvent cell “looks to be a hybrid cell type, taking parts of existing cell types and putting them together into a new combination,” said Todd Oakley, who studies the evolution of eyes and bioluminescence at the University of California, Santa Barbara and did not participate in the Caltech project. The scientific literature on such expression hybrids is limited “because we didn’t have the technology to study all the genes that are expressed in individual cell types until recently,” he said. “And yet, that is a principle that we see time and time again in evolution — combining existing parts into a new thing.” He compares the evolutionary principle to the art technique called bricolage, in which found materials are assembled inventively into new creations.
One Signal Can Be Enough
The modularity of these gene regulatory networks means that a single transcription factor may be all it takes to make a new cell type, because it can activate or repress entire suites of genes involved in differentiation. In a study presented at the Society for Developmental Biology’s annual meeting last month, Babonis showed that cnidocytes — the stinging cells found in sea anemones, jellyfish, corals and other members of the marine invertebrate group called cnidarians — arise from a cell lineage that also produces a specific set of neurons. Babonis found that a single transcription factor was enough to stop immature cells from developing as neurons and turn them into stinging cells instead, complete with a barbed, coiled filament for capturing prey.
Parker believes that it may be relatively easy for beetles to invent novel features by reusing existing sets of genes in new contexts. “I think insects are doing this all the time,” he said. “They’re always evolving little patches of pheromone-producing cells or defensive glands.” This could explain why gland cells are among the most rapidly and convergently evolving types of cells in all animals.
Although the rove beetles likely evolved fatty acid derivatives as defenses early in their history, compounds like alkanes and esters are not by themselves particularly good for repelling predators. The benzoquinones are the real key, because they bind to and activate certain pain receptors. The addition of this second component to the chemical defenses “surely would have had macroevolutionary consequences,” Parker said, and it may well explain why the branch of rove beetles that makes benzoquinones has 17,000 species while an earlier sister branch that does not has only a few dozen.
While the solvent cells are clearly derived from the exoskeleton membrane cells, the developmental origin of the novel benzoquinone-producing cells is still mysterious. However, the researchers discovered that the benzoquinone-producing cells expressed high levels of two enzymes related to enzymes in the “tanning” process that hardens and darkens the cuticle of the exoskeleton. When they used RNA interference to silence the enzymes, the researchers found that the beetles could no longer produce benzoquinones.
In short, enzymes that initially evolved for cuticle tanning seem to have been redeployed in the defense gland. “These are cassettes that have evolved to work in one context, and function together pretty effectively when redeployed in another context,” Parker said.
Yet the benzoquinones would not have been effective defenses for the beetles if they had evolved in the absence of the alkanes and esters. Benzoquinones are solids; they need to be dissolved in the carbon solvents to be sprayed or smeared on predators.
Parker believes that it was only after the solvent cells evolved that an opportunity arose for the benzoquinone-making gland cells to emerge. “All of a sudden, you have this really effective chemical defense mechanism,” he said. “As soon as you get the second cell type that changes the function of the organ, it cements the two things together as a unit that will coevolve with each other.”
There is already evidence that these two principles — the creation of new physiological niches for cells and the repurposing of ancient genetic circuitry — operate in species other than beetles. A few years ago, Oakley saw them at work while studying the evolution of eyes, which have evolved independently at least nine times in cnidarians alone.
Oakley and his colleagues found that in all nine of those cases, many of the molecular components of the photoreceptor cells, pigment cells and lens cells had previous roles in the stress response to light. For example, the original role of the cryptochrome gene was to use energy from ultraviolet light to repair DNA damage. As cryptochrome became highly expressed in areas of cnidarian bodies that received a lot of light, variant copies of that gene evolved into photoreceptors that helped set the animals’ circadian rhythm.
Evolving Under Stress
Oakley suspects that similar mechanisms for coping with stress from light might have gradually shaped photosensitive cells into photoreceptors. That could have initiated a further chain of events in which UV-protective pigment cells evolved to shield the photoreceptor cells — and that shielding provided the opportunity for the photoreceptor cells to detect the direction of incident light, not just its intensity. A capability for directionality in turn created the opportunity for lenses to evolve to fine-tune the directional information. Lenses seem to be the result of many heat shock proteins and detoxification enzymes crystallizing together.
“Time and time again, there are these linkages to stress — heat stress, UV stress and oxidative damage — which is caused by light,” Oakley said. All of this “fits the principle of working with what’s there — the bricolage idea.”
Recently, Oakley has also directed some of his lab’s energy toward another evolutionary innovation, one that has independently evolved almost 100 times: bioluminescence. Bioluminescent ostracod crustaceans, like tiny fireflies of the sea, have a gland that makes their bioluminescent goo. One type of cell in the gland secretes a luciferase enzyme, while a second type of cell secretes the substrate vargulin. When these chemicals mix together in the secreted mucus, the luciferase oxidizes the vargulin, and that change in energy is released as light. Lisa Mesrop and other members of Oakley’s team are currently working to understand the origin of the cell types that make and secrete the luciferase and the vargulin.
While the questions about the origins of new organs are old, the study of how novel organs evolve is only in its infancy. But the rise of new techniques like single-cell RNA sequencing means that “now we’re actually starting to get the ability to probe and understand what’s going on genetically,” said Jacob Musser, who studies cell-type evolution at the European Molecular Biology Laboratory in Heidelberg. “We don’t necessarily all have agreement on exactly what is going on, or how to interpret some of our data. But that’s the nature of an emerging field.”