How Is Flocking Like Computing?
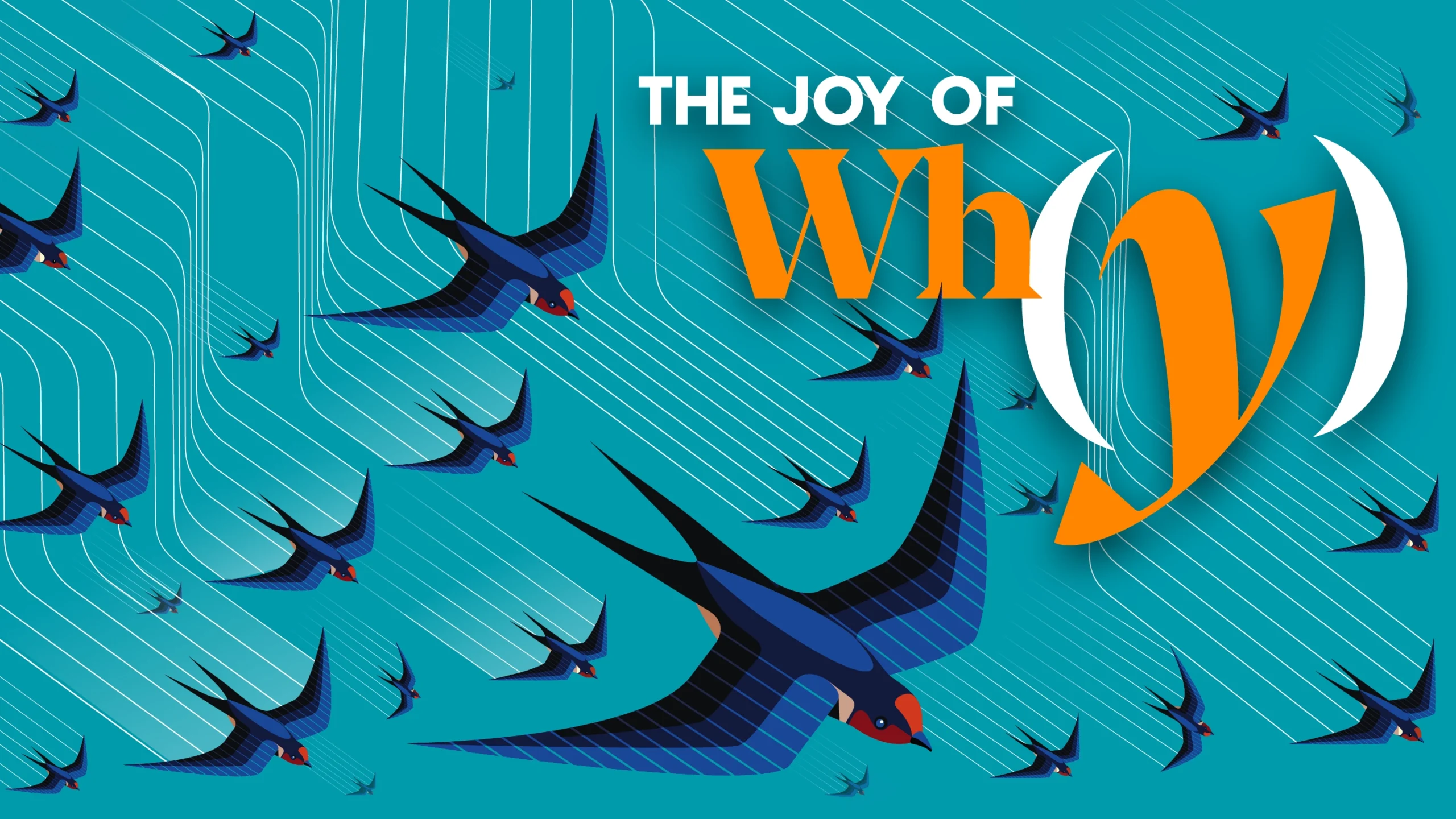
Peter Greenwood for Quanta Magazine
Introduction
Birds flock. Locusts swarm. Fish school. Within assemblies of organisms that seem as though they could get chaotic, order somehow emerges. The collective behaviors of animals differ in their details from one species to another, but they largely adhere to principles of collective motion that physicists have worked out over centuries. Now, using technologies that only recently became available, researchers have been able to study these patterns of behavior more closely than ever before.
In this episode, the evolutionary ecologist Iain Couzin talks with co-host Steven Strogatz about how and why animals exhibit collective behaviors, flocking as a form of biological computation, and some of the hidden fitness advantages of living as part of a self-organized group rather than as an individual. They also discuss how an improved understanding of swarming pests such as locusts could help to protect global food security.
Listen on Apple Podcasts, Spotify, Google Podcasts, TuneIn or your favorite podcasting app, or you can stream it from Quanta.
Transcript
[Theme plays]
STEVEN STROGATZ: Throughout the animal kingdom, from tiny gnats to fish, birds, gazelles, even primates like us, creatures tend to organize into large moving patterns that pursue a seemingly spontaneous collective goal. Often, no individual creature appears to act as the leader, orchestrating these mass movements. Rather, the animals just seamlessly fall into line.
And even though it feels like such systems would teeter into chaos or instability, these collectives somehow manage to move in ways that appear extraordinarily well-coordinated and purposeful, as anyone who has watched a murmuration of starlings or a school of fish can attest. But what’s the driving force behind this behavior?
I’m Steve Strogatz, and this is “The Joy of Why,” a podcast from Quanta Magazine where my co-host Janna Levin and I take turns exploring some of the biggest unanswered questions in math and science today.
[Theme ends]
In this episode, we’re going to be getting to the heart of why animals flock, swarm and school. How are the latest technologies, like artificial intelligence and 3D cameras, providing new insight? And what can studying animal group dynamics tell us about ourselves, both individually and as collectives?
Here to shed light on these mysteries is evolutionary ecologist Iain Couzin. Iain is the director of the Department of Collective Behavior at the Max Planck Institute of Animal Behavior and a full professor at the University of Konstanz. Among the many honors he’s received are the National Geographic Emerging Explorer Award, the Lagrange Prize, the highest honor in the field of complexity science, and the Leibniz Prize, Germany’s highest research honor. Iain, we’re so happy to have you with us today.
IAIN COUZIN: It’s great to be here, Steve.
STROGATZ: Well, I’m very happy to see you again. We’re old friends, and this is going to be a real treat to hear about the latest in collective behavior. But let’s begin — I suppose we should talk about, who are your specimens? Could you tell us a little about some of the animals, and the variety of forms that their collective behavior take in the systems that you’ve studied?
COUZIN: Well, that’s one of the most amazing things about studying collective behavior. It’s that it’s central to so many processes on life on our planet that we literally study a range of organisms, from the simplest animal on the planet — it’s called a placozoa; it’s a basal phylum, possibly the simplest multicellular animal on the planet; it’s a swarm of cells, thousands of cells, much moving like a bird flock or a fish school — up through the invertebrates, like ants, that have amazing coordinated behavior, or locusts, that form some of the largest, most devastating swarms, to vertebrates, such as schooling fish, flocking birds, herding ungulates, and primates, including ourselves — humans.
STROGATZ: So, it really seems to run the whole gamut, all the way from — I have to admit, I’d never heard of this, did I get it right: placozoa?
COUZIN: Placozoa, yeah. This little creature was found crawling around on the glass of aquaria, tropical aquaria. You can see it with the naked eye. It’s about a millimeter, maybe a millimeter and a half if it’s very big. And, you know, looking into this remarkable creature has only really recently sort of drawn the attention of scientists.
And that is largely because this strange little quirky swarm of cells actually has the genetic complexity that you would associate with a much more sophisticated organism. For example, it has a large range of neurotransmitters, yet it doesn’t have neurons.
[STROGATZ laughs]
It has what are called Hox genes. Hox genes are in developmental biology associated with complex body plans. It does not have a complex body plan. And so perhaps you may think, well, this creature may have evolved to become more complicated and then re-evolved to simplify itself, and therefore it kept these characteristics of complexity.
But genetic researchers published a sort of landmark paper in the journal Nature that showed, no, in actual fact, this is one of the most primal groups of cells. And of course, collective behavior, what more beautiful example than cells coming together to form an organism. You know? So this is one of the reasons we study this: to try to understand how collective behavior was central to the origins of complex life on our planet.
STROGATZ: Man, this is an early stage in the interview and you’re already blowing my mind. You’re also derailing me from what I thought I was going to be talking to you about. This is so interesting and so new to me that I’m stunned. I want to come back to this part of the story because it’s so — I mean, it’s really surprising that they would have… Did I hear you right, that they have things associated with having a nervous system, but have no nervous system? And have developmental biological genes as if they needed to evolve a whole complicated body plan like a fruit fly, but they don’t have a body like that?
COUZIN: Exactly, exactly. And so, they could really give us a hint at the origins of intelligence. Our particular study, which we published this year, you know, we showed that the body plan that they have really does behave very much like a bird flock or a fish school, with cells locally interacting with others and tending to align their direction of travel.
So they’re attracted to each other. They’re sort of connected together like an elastic sheet, but they tend to also be motile. They’ve got cilia, little cilia on their base, so they can flow along the environment. And the forces that they apply to their near neighbors cause them to align with each other.
And so, if we track these cells under a microscope, and we look at the alignment and we look at the attraction of the individuals, we use very much the same technologies, the same models, the same thinking that we use for collective behavior in bird flocks or fish schools or other types of groups but apply it to these animals.
And so, this is one of the things I find most remarkable about collective behavior, is that even though the system properties, whether you’re a cell or whether you’re a bird, are very different, when you look at the collective action, the collective properties, the mathematics that underlie this, actually can turn out to be very similar. And so we can find these, sort of, what are called universal properties that connect these different, apparently disparate systems.
STROGATZ: Well, of course, now you’re speaking my language, since, you know, that’s what drew me into my own fascination with collective behavior, is that there are those universal mathematical principles that seem to apply up and down the scale from cells to, well, of course, we always like to put ourself at the top.
But, so, okay, you’ve raised so many different issues for us to think about. Let me try to go back to the beginning, much as I’d love to stay with you here with the Placozoa.
So, for example, you mentioned words like “flocks” and “schools,” and sometimes we hear people talk about “swarms,” like with insects. Is there any reason we have three different words for the same thing? Are they not really the same thing when we talk about collective groups? Is there a reason we shouldn’t talk about, like, schooling birds or swarming fish?
COUZIN: No, I think we’ve developed these words, and different languages have different words. In German, which is a language replete with many words, they actually have relatively few. Whereas in English, we have many, many different words. Like, you know, for example, a group of crows is called a murder of crows.
[STROGATZ laughs]
You yourself earlier used a wonderful word, a “murmuration” of starlings. And I think it’s that, it’s the beauty, the captivating beauty of flocking and schooling and swarming, that’s given rise to these wonderful words that can be associated with particular examples.
And so, I think that’s a very useful thing, because earlier on I was emphasizing the commonalities, the mathematical commonalities, but there are also differences. There is a difference between a swarm of cells and a swarm of birds. And so, to understand these systems, we both have to consider the principles in common, but also those that differ between the systems. And in a way, language kind of captures some of that for us in the way that humans have naturally sort of segregated or divided these into different categories.
STROGATZ: Interesting. So, you mentioned “swarm of cells” and “swarm of insects,” I guess it was, and you said there could be some differences even though we use the same word. What are the things that we should distinguish between those examples?
COUZIN: Yeah, I think what’s really exciting is why there’s a commonality, because the differences are so profound. An animal has a brain. It’s taking in complex sensory information and trying to make decisions about its environment. Animals are capable of much more complex, sophisticated behaviors, on average, than cells.
But cells, of course, themselves have complex internal processes. But their interactions are dominated to a greater degree by physical forces, by the scale at which they’re acting and the tensions that form, the physical tensions that form within the cell aggregate.
Whereas the animals, the interactions between birds in a flock, they’re invisible. They have no physical form. And so one may initially think, well, then it’s only an analogy. In fact, I would say until about five to 10 years ago, I thought it was just an analogy too. I thought that these differences must be very important. But what we’re beginning to understand is that the common feature that they share is computation.
It’s that these elements are gathering together to compute about their environment in ways that they can’t compute on their own. Each individual, even if you’ve got a very complex human brain, and you’re walking around in the world, unless you have social interactions with others, or even more so, you know, build upon the cultural complexity that we inherit when we are born into our lives, then we’re very limited.
And so, there’s these deep, sort of very fascinating questions that we’re just beginning to address about computation and the emergence of complex life.
STROGATZ: Such an interesting point of view. I didn’t know what word you were going to say when you said there’s something they all have in common. I was — couldn’t guess, but I like it: computation.
So, you know, it makes me think of a famous thing that people may have seen movies of on YouTube or on television, where there’s a flock of birds — maybe it’s a starling — and a hawk or a falcon or something comes zooming in toward the flock. Maybe you should give us a visual description of what happens next, and why am I thinking that there’s anything to do with computation in this example?
COUZIN: Well, I mean, if you look at these groups, you know, when you have these predators present and attacking these groups, whether it’s a fish school or a bird flock, you see the group behaving as this sort of undulating fluid. You see these ripples of light crossing the group or ripples of density crossing the group.
And what this is indicative of is that the individuals can actually propagate information about the location of that predator very rapidly via social interactions. So individuals that see the predator, for example — maybe only a few of them initially see the predator. But by turning, then this behavior being copied by others, the change of density, the change of turning, is propagated extremely rapidly.
And if we use — I’m sure we’ll get to this later — if we use advanced imaging tools to quantify, to measure, these waves of turning, it results in a wave of propagation that’s around 10 times faster than the maximum speed of the predator itself. So individuals can respond to a predator that they don’t even see.
So, the group and the individuals in the group — because selection, natural selection, is acting on the individuals — typically, they can actually respond to stimuli that they don’t detect.
It’s a little bit like, you know, a neuron transmitting information via electrical signals. In this case, it’s not electrical signals. It’s really the density and the turning of the individuals that percolates across the group, but it gives those individuals afar information where the threat is, so they can begin to move away from it very quickly.
STROGATZ: So that is, I think, a very beautiful visual example of what computation would mean in this context. That we can see these waves of panic or avoidance flowing through the flock. It’s so interesting that it’s much faster than the individuals would be able to do on their own, and, I guess, faster than what the predator can muster on its own.
COUZIN: One of the reasons why this is likely to be, why we think this is, is because the group — the natural selection, even though it’s acting on the individuals, it’s their fitness that matters, there’s such a collective benefit to everybody if they behave in a certain way.
This again relates to what we’ve learned from physical systems, specifically physical systems close to a phase transition. So, a system that’s close to a transition between different states, such as between a solid and a liquid, you know, if you’re freezing water and it suddenly transitions into a solid, the collective behavior of that system is quite remarkable near that transition point, this bifurcation, which of course is your own area of study. And this is something that we now know, we now have very strong evidence, that natural selection pushes systems close to these bifurcation points because of the collective properties, the remarkable collective properties, that are exhibited.
When we first measured these properties, it seemed like the individuals were defying the laws of physics. The information was percolating so quickly.
And in the, sort of, early 1900s, Edmund Selous, who was a confirmed Darwinian, but, you know, also sort of captivated by the fascination with telepathy in the Victorian era, he assumed there must be thought transference, he described it, or telepathy between birds that allowed them to communicate so quickly.
And of course people, you know, think, “Well, that’s ridiculous, of course there can’t be telepathy.” But in actual fact, and this is maybe a little controversial, but in actual fact, I think we still don’t have a good grasp of the sensory modalities and the way in which this information percolates so exquisitely rapidly across the system.
I’m not suggesting there’s telepathy, of course. But I’m suggesting that by tuning a system, by tuning a collective system close to this critical point, close to this bifurcation point, it could give rise to remarkable collective properties that, to an observer, look fantastical, to an observer, look bizarre. Because the physics in these regimes is bizarre, is fantastical, is amazing, even though it’s understandable by science.
STROGATZ: So I’m just wondering, with now in the case of collective behavior, if nature tunes a flock to be near some kind of point of instability or criticality. Are you suggesting that’s part of what makes it so effective?
COUZIN: Yeah, that’s exactly what I’m suggesting. And so, for example, you know, again, a very recent paper within the last couple of years that we published, we asked, you know, what about getting the best of all worlds? What about if, you know, under general conditions you want to be stable, you want to be robust. But sometimes, you want to become hypersensitive. And so in natural selection, biological systems have to balance this amazing, sort of seemingly contradictory status of being both robust and sensitive. How can you be both robust and sensitive at the same time?
And so, we think that, you know, tuning the system close to this critical point, actually allows that to happen because if the system deviates, it actually stabilizes itself. But as it gets pushed towards that critical point, it becomes incredibly flexible and sensitive to inputs, so for example, inputs regarding that predator. So if a fish school is far away from that critical point — for example, if they’re very strongly aligned with each other — and they detect a predator, in actual fact it takes a lot of effort to turn all of these individuals. They’re so strongly responding to each other that it’s hard for that external input to change their behavior.
If, on the other hand, they’re very disordered and they’re all moving in different directions, then an individual changing direction can hardly be perceived by others and so it doesn’t propagate through the system.
And so at this sort of intermediate point, they can actually optimize their ability to behave as a group and to be flexible, but to transmit information. And this is a theory from physics that’s been longstanding, but it’s only really within the last few years using computer vision technology to track animals in groups and to ask, how do you change, you know, your interactions when, for example, the world gets more risky?
We would always think as biologists, “Well, if the world gets more risky and more dangerous, I will become more sensitive to inputs. I’ll be more jittery, I’ll be more likely to make a false alarm.” And that’s true of animals in isolation. It’s true of humans when we’re behaving in isolation. But we tested this in animal groups, groups that have evolved within the context of the collective, and we find that’s not true of them.
What they do is they change the network, the network of connectivity, of how the information flows through the system. And they tune it such as to optimize this sort of flexibility-robustness tradeoff, i.e., they take it into this critical regime as we had predicted.
STROGATZ: Which kinds of animals were these studies done on?
COUZIN: So we mostly work with small schooling fish because they have to solve the same kind of problems — avoiding predators, finding suitable habitat — yet they’re tractable within a laboratory environment. So fish actually have a chemical, which is called schreckstoff, which in German literally just means “scary stuff.” And schreckstoff is naturally released, if a predator attacks a fish, it has to release this chemical.
So we can put schreckstoff in the water, so there’s no location of a predator, but individuals’ judgment about this environment changes, the world has become more risky.
So what do you do, do you change what’s going on in your brain? Do you change how you interact with the environment? Do you become more scared, which is the natural thing we may think animals do?
Or, if you imagine, in a network system, in a collective system, do you change the topology of that network, the social network, the way you communicate with others? Because that can also change the responsiveness to threats, because of this wave of turning that we talked about before.
And so what we found was that individuals do not change. What happens is the network changes. The individuals move to change the structure of that network, and it’s that that causes the group to suddenly become more sensitive and more flexible.
People used to, for example, have a proxy, which is that individuals that are close to each other must be interacting more strongly. But, as you can think about in your daily life, you could be sitting beside a complete stranger on the bus, and not actually be socially strongly connected to them on average. So, the social network that individuals experience might be very different from the one that’s easy to measure.
So what we’ve done is — well, it’s quite complicated. But what we can do is we reconstruct the world from their perspective. And we use a technique that comes from video games and computer graphics called raycasting, where we cast rays of light onto the retina of the individuals so we can see a sort of computerized representation of what they see for each moment in time. But what we don’t know is, how on earth do they process that?
And so again, we can use machine learning methods, because every brain has evolved to do the same thing. It’s taken complex sensory information — like people listening to us today. It’s a complex acoustic information, but they may be driving or maybe cooking, so they’ve also got complex visual and olfactory information, but their brain has to take all this complexity and reduce it down into what’s called dimensionality reduction, into a decision or into “what am I going to do next?” And we’ve known very, very little about how real animals do this.
But we can reconstruct their visual fields, and then we can use the same types of techniques to reduce the dimensionality, to understand how does the brain reduce this complexity to movement decisions?
And the fish that we studied, they have a very small number of neurons in the back of the brain that dictates all of their movements. So the brain has to take in all of this complexity, and it has to reduce it down, and it has to make decisions. And I think it’s a wonderful question in biology as to how do brains do that?
STROGATZ: First of all, I can tell that I need to be reading your papers more frequently. You said something about shining lights on the retinas of the fish to then see what they’re seeing, or to have a feeling that you know what they’re looking at? Did I hear that right?
COUZIN: Yeah, it’s not literally shining a light, actually. It’s all digitally done. So imagine you have a fish school at a snapshot in time, a frozen moment in time. Our software tracks the position and also the body posture of each of those fish. And what we can do is we can now create a three-dimensional computer version of that scene, like in a video game. We can then ask, what does each individual see? So we can put cameras in the eyes of the individuals.
And so, raycasting is a bit like raytracing, which is used in computer graphics, which is just the pathways of light falling on the retina. And we do all this digitally, so we can create a digital analog of reality. We can then look to see how light would fall on the retina in that virtual scene, a sort of photorealistic virtual scene. And so that gives us the first layer: What is the information coming in to the individual?
And then, of course, the big question that we want to ask is, how does the brain process that? How does the brain take that complexity down, and how does it make decisions? How do, for example, fluid flocks and fish schools move so effortlessly and so beautifully with so few collisions, and yet cars on a highway tend to struggle to have collective motion? I mean, is there something we can learn from millennia of natural selection that we can then apply to vehicles and to robots?
So there’s also an applied element to trying to understand this. I want to understand it largely because I find it fascinating, but also, it does actually translate to real applications in certain cases.
STROGATZ: We’ll be right back.
[Break]
STROGATZ: Welcome back to “The Joy of Why.”
I’d like to return to something you said back in the introduction when you were going across the scales from cellular up to primates, and so on. People may not be so familiar with the locust example, and I wonder if we could talk about some of the — let’s call them real-world or even economic aspects of flocking, because locusts have a big impact on the world, bigger than I had realized. I mean, I’m looking at some statistics here in my notes that, during plague years, locusts invade more than one-fifth of the world’s land cover.
COUZIN: Yeah.
STROGATZ: Can you believe it? And affect the livelihood of one in 10 people on the planet. So could you talk to us a little about that kind of research and how it relates to questions of global food security?
COUZIN: Yeah, you’re absolutely right. And I find this quite astonishing. You know, as you just said, they impact one in 10 people on our planet through food shortage and food security. And they often do so in countries, you know, like Yemen and Somalia, that have major problems, major conflicts, and civil wars, and so on.
But also due to climate change, the range of locusts is expanding across much of its range. And so, I mean, at the moment, this year Afghanistan is facing a major crisis in its food basin. A couple of years ago, it was Madagascar. A year or two before that, it was Kenya having the largest swarm in 70 years.
So why, you know, with all of the modern technologies that we have for monitoring, why are the swarms getting more ferocious and more severe, you know? And one of the reasons is climate change. It’s that, you know, what happens with these swarms is — so locusts, it might be surprising for listeners to know this, but locusts actually don’t like being near each other. They are shy, cryptic green grasshoppers that like to be left alone. So if they have plenty of food, they’re just isolated from each other. They avoid each other. It’s only when they’re forced to come together do they transition.
So they’re normally what are called solitarious, because of their solitary lifestyle. But if they’re forced to come together, they’ve evolved to transition. They’re sort of the Jekyll and Hyde of the insect world. They’ve evolved to transition quite suddenly, within an hour, behaviorally, into a gregarious form, where they start marching towards each other, following each other.
Another thing people may not know is that locusts actually don’t have wings for the first several months of their lives. And so when locusts are born, they’re flightless. They’re these flightless nymphs. It’s only when they’re adults do they have wings.
And so, what’s happening here is that when rains come into Africa, for example, or into India, or into other areas, then you can have lush vegetation, and the small locust population can proliferate as these sort of cryptic grasshoppers, they can grow in population size. Now, as that population grows, they eat more and more and more, and often there can also be a drought coming.
Now, if you’ve got a high population density, and then suddenly the food disappears, then what the locusts do is, they’ve evolved to transition to this gregarious form, where they start marching together. They start all moving together. These swarms can be billions of individuals — as far as you can see, locusts all marching in unison, as if in a common purpose. And once they grow wings, they can take flight. And then it gets even worse, because they can access the trade winds or other, you know, environmental conditions, where they can transfer themselves as massive swarms over hundreds or even thousands of kilometers. And so, this is one of the biggest and most devastating collective behaviors we have on our planet.
STROGATZ: Whew, I can’t say that I’m very familiar with the idea of locusts marching. We’re used to thinking of them as these clouds, you know, swarming in the air. But tell us a little more about the marching, because I do vaguely remember some astonishing research of yours with the cannibalistic aspect of locusts, is that the right word to use?
COUZIN: Yeah, that was in 2008, and — but you’re right, you know, these huge flocks or swarms or clouds of locusts that transition over great distances, you know, we don’t know much about them because we did not have the technology to study that. In fact, we still don’t have the technology to study that. So it’s not that it’s not important, it’s incredibly important.
But we also know that what precedes these flying swarms — I mean, the flying swarm is a bit like a wildfire that’s already got out of control. Now you’re really going to have problems controlling it. But if you can control it before they grow wings, you know, when they’re forming these swarms in the desert or these environments before that, then there’s great potential.
And so, for practical reasons, we focused on these wingless swarms. And in actual fact, you know, even though you’re right, I started studying these in the mid-2000s, we’re now, I’m now returning to locusts, and I’m now studying them again.
We’ve just created the world’s first proper swarm within the laboratory environment ever, earlier this year, where we tracked 10,000 locusts in a 15-by-15-by-8-meter imaging environment that we built here specifically for the purpose, here in Konstanz. So it’s funny that you’re mentioning it, because my research is now kind of looping back to this same system.
But, yes, as you said, what we discovered was, you know, these insects, well, why are they marching together? Why are they — you know, and we initially thought it must be like fish schools and bird flocks. It must be about information. It must be about collective intelligence. Well, we were wrong. And so this is the great danger. If you see, you know, a swarm of ants that’s moving in a circle, moving in a sort of mill, and you see a fish school, for example, turning in a torus or a sort of doughnut-like pattern, or you see a whirlwind, these are all patterns that look the same, but they may be driven by very, very different phenomena.
And I think I was misled in thinking, you know, when you see collective motion, it must be similar processes that underlie it. But in the case of locusts, it was not this sort of information transfer hypothesis. It was actually the fact that in these desert environments, when the food suddenly becomes short, you’re desperately short of essential nutrients, especially in the desert: protein, salt and water.
And what is better for you in this sort of harsh environment than another individual? Because they are perfectly balanced nutritional composition. So what the individuals do is, they’re attracted to each other, and they tend to cannibalize each other. So they’ve evolved to follow those that are moving away, to try and bite them at their rear, at the rear of the abdomen, which is very hard to defend against. The head is heavily armored, but the rear of the abdomen is a weak point for obvious reasons, there has to be an orifice there.
And so they target that, but then they also avoid being targeted by others. And the outcome of following those that are moving away from you and moving away from those moving towards you results in the whole swarm beginning to march together across this desert environment.
And they also benefit by advecting, by moving out of nutrient-poor areas together. Because, you know, if you put a human in the desert, a human will tend to sort of get disoriented and tend to move around in circles. Same with a locust. But if you put them in a swarm, the collective alignment, the synchrony among the individuals, you know, hundreds of millions of individuals aligning with each other, they can march in a very directed fashion out of these nutrient-poor environments. And they can also swamp predators. You know, predators just can’t make much of an indent here.
STROGATZ: It makes me wonder, actually, as we talk about all these examples, how did you become interested in all of this, back in the old days? You mentioned this was back in 2008?
COUZIN: Yeah, that was that paper in 2008.
STROGATZ: Yeah, you were busy on this even before that, right?
COUZIN: Yeah, I did my Ph.D. in the late nineties on ants. I was fascinated by ant behavior. And to be honest, it started with a passion for nature and an obsession with just natural history and observing what was around us.
I thought, as a child, there must be an expert that understands why swarms form, why fish school, why birds flock. I thought this must be something that everybody studies.
I was an artist as a child. I was very interested in creative writing and poetry and art. And so, I was initially drawn in by the pure beauty, the fascination by the beauty of these.
And at high school, I was not a great student in science. I was doing pottery and I was doing painting. And when I went to university, I remember my dad said to me, “You know, son, you should do what you’re good at. Do English or art. You’re not a scientist, you’re a naturalist, you know?” And he was right. He was absolutely right.
And it was then later when I did do a biology degree, and I just knew in the very first lecture of my biology lecture, I knew this was the right thing for me, I just knew it. And I discovered that there’s this whole world of statistical physics. These papers came out in that time, and they blew my mind because they were authors that were seeing deep mathematical principles across systems.
My Ph.D. advisor said, you know, to get a job, you should become the world expert in one species of ant, and then you can be valuable. But I was reading this work of scientists that were doing the exact opposite. They were studying everything, from physical systems to biological systems, and they were seeing these principles. And also, the patterns and the structures and the results they were finding were just naturally beautiful. And so I thought, this has to be right. This has to be the right way to do science. And so, at that time, I just got drawn into the world of physics.
STROGATZ: Did you ever have the pleasure of talking to your dad afterward about your change in direction?
COUZIN: I never, ever thought my dad remembered this. And then when I got promoted from assistant professor to full professor at Princeton University, I got a phone call from the chair of the department that said, “Congratulations, Professor Couzin.” And, you know, I was just completely blown away, so of course I called my mom and dad, and my dad answered the phone, and then he said, “And to think I called you a naturalist.” That’s the only time, that’s decades later. I never knew he even remembered this discussion.
STROGATZ: Well, it’s a good story, it’s a really good story. We like to talk about big unanswered questions on this show, and so, what do you see as some of the biggest unanswered questions about flocks and schools and collective behavior generally?
COUZIN: Well, absolutely I do. And this is getting me onto the topic that I’m so excited about now. So again, earlier in my career, I thought, you know, the brain, of course, is a wonderful collective computation entity, one of the most beautiful examples, you know. How does the brain make decisions? And it’s a collection of neurons, and of course we have ant swarms, or locust swarms, or bird flocks, or fish schools, all of these different components interacting together. So, is there something deeply connecting these different systems, or not? And what I’m fascinated at the moment about is collective decision-making, and especially collective decision-making in space.
So, how does the brain represent space-time? And how does that matter in terms of decisions? And what on earth does that have to do with collective behavior of animals? What I realized about five years ago, is that I think there’s a deep mathematical similarity, and I think there are deep geometric principles, about how the brain represents space and also time.
And one of the most exciting things here is the use of mathematics again. You know, I dropped maths when I was 16 years old, and I’ve just spent a sabbatical at the Isaac Newton Institute for Mathematical Sciences at Cambridge University as a Distinguished Fellow. Yet, I can’t solve an equation, you know?
So I’m, but I love the fact that I can work with amazing mathematicians. And by working with physicists and mathematicians and biologists, and by conducting experiments on animals in virtual reality — we’ve built a suite of technologies here. So we can’t put a headset like a Meta Quest 3 on a fish that’s less than a centimeter long. But we can create virtual, immersive, holographic environments, so we can completely control the input. We can completely control the causal relationships.
If, you know, I’m influencing you and you’re influencing me, and then there’s a third individual, are they influencing me directly or via you? Or both? Or a fourth individual or fifth? And in our virtual reality environments, we can put these individuals into what we call the Matrix, like in the movie, where each individual is in its own holographic world and interacting in real time with holograms of other individuals.
But in this world, we can play around with the rules of physics. We can play around with the rules of space and time to understand better, how does the brain integrate these?
And so, this is really blowing my mind because we can show that the brain does not represent space in a Euclidean way. It represents space in a non-Euclidean coordinate system. And we can then show mathematically why this is so important, which is that when you start dealing with three or more options, then actually warping spacetime, making space non-Euclidean, can dramatically reduce the complexity of the world into a series of bifurcations. And close to each bifurcation, it amplifies differences between the remaining options. So there’s this beautiful internal structure.
And so, we think we have this universal theory of how the brain makes spatial decisions that we couldn’t have ever got at without looking at a range of organisms like fish and locusts and flies within these types of virtual reality environments, and so that’s what I’m super excited about.
[Theme plays]
STROGATZ: Well, I can’t wait to hear about all of this as you work it out. I could go on with you all day, but I think it is time to say thank you. We’ve been speaking with evolutionary ecologist Iain Couzin about flocking, swarming, schooling and all sorts of collective behavior. Iain, it’s been such a pleasure learning about what you’re up to and the wonders of nature that you’ve helped unravel for us all. Thanks very much.
COUZIN: It’s been a pleasure. Thanks, Steve.
[Theme continues to play]
STROGATZ: Thanks for listening. If you’re enjoying “The Joy of Why” and you’re not already subscribed, hit the subscribe or follow button where you’re listening. You can also leave a review for the show. It helps people find this podcast.
“The Joy of Why” is a podcast from Quanta Magazine, an editorially independent publication supported by the Simons Foundation. Funding decisions by the Simons Foundation have no influence on the selection of topics, guests or other editorial decisions in this podcast or in Quanta Magazine.
“The Joy of Why” is produced by PRX Productions. The production team is Caitlin Faulds, Livia Brock, Genevieve Sponsler and Merritt Jacob. The executive producer of PRX Productions is Jocelyn Gonzales. Morgan Church and Edwin Ochoa provided additional assistance.
From Quanta Magazine, John Rennie and Thomas Lin provided editorial guidance, with support from Matt Carlstrom, Samuel Velasco, Nona Griffin, Arleen Santana and Madison Goldberg.
Our theme music is from APM Music. Julian Lin came up with the podcast name. The episode art is by Peter Greenwood and our logo is by Jaki King and Kristina Armitage. Special thanks to the Columbia Journalism School and Bert Odom-Reed at the Cornell Broadcast Studios.
I’m your host, Steve Strogatz. If you have any questions or comments for us, please email us at [email protected]. Thanks for listening.