How the ‘Diamond of the Plant World’ Helped Land Plants Evolve
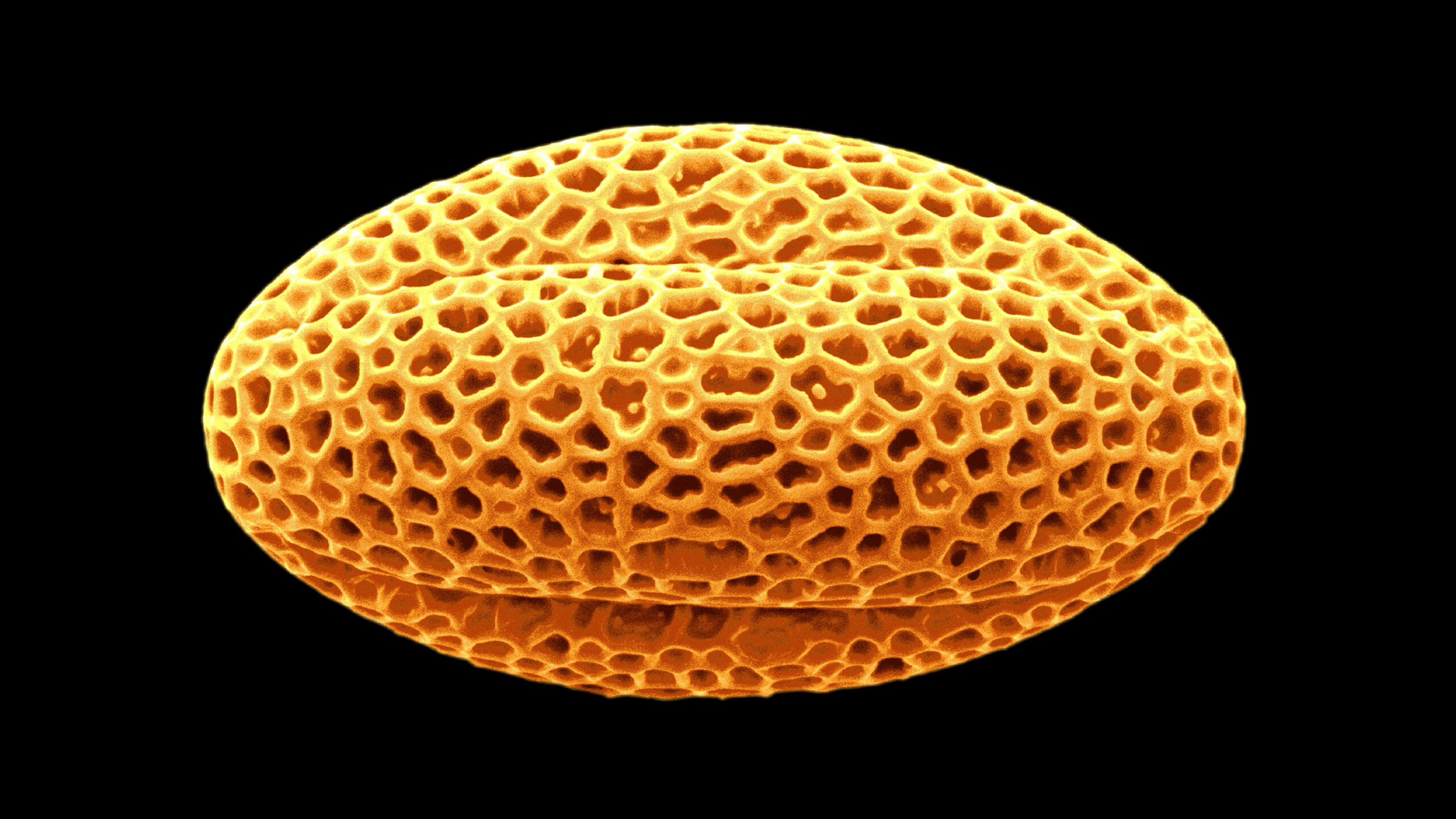
The latticed structure of the hard outer portion of this grain of radish pollen consists of sporopollenin, a remarkably hardy and inert material that defied thorough scientific analysis for two centuries.
Scimat / Science Source
Introduction
When Fu-Shuang Li, a biochemist and research scientist at the Whitehead Institute in Cambridge, Massachusetts, needed some pollen for his research, he knew just where to go. Every spring, the pitch pine trees ringing Walden Pond in Concord release clouds of golden pollen that coat the water and gather in galactic swirls against the shore. Henry David Thoreau, who spent two years living beside the pond in the 1840s, closes his famous account of the experience by describing so much pollen, “you could have collected a barrelful.”
Crouching at the pond’s edge in a black hoodie and sweatpants, Li dipped in a test tube, drawing out a few hundred milliliters of water, laden with pollen and whatever else was growing in it. It was far from a barrelful of the stuff, but it was more than enough for Li’s efforts to study the molecular structure of pollen’s outer shell. Called sporopollenin, the material that makes up the shell is so tough it has sometimes been called the diamond of the plant world.
For more than a century, scientists have tried to understand the chemical basis for sporopollenin’s unparalleled strength. Sporopollenin shields the DNA in pollen and spores from light, heat, cold and desiccation. Without it, plants would be unable to live on land. But sporopollenin’s toughness made it tough to study, even decades after the molecular structures of cellulose, lignin and other basic plant polymers had been puzzled out. “Nature evolved sporopollenin to resist any attack,” said Li. “Including by scientists.”
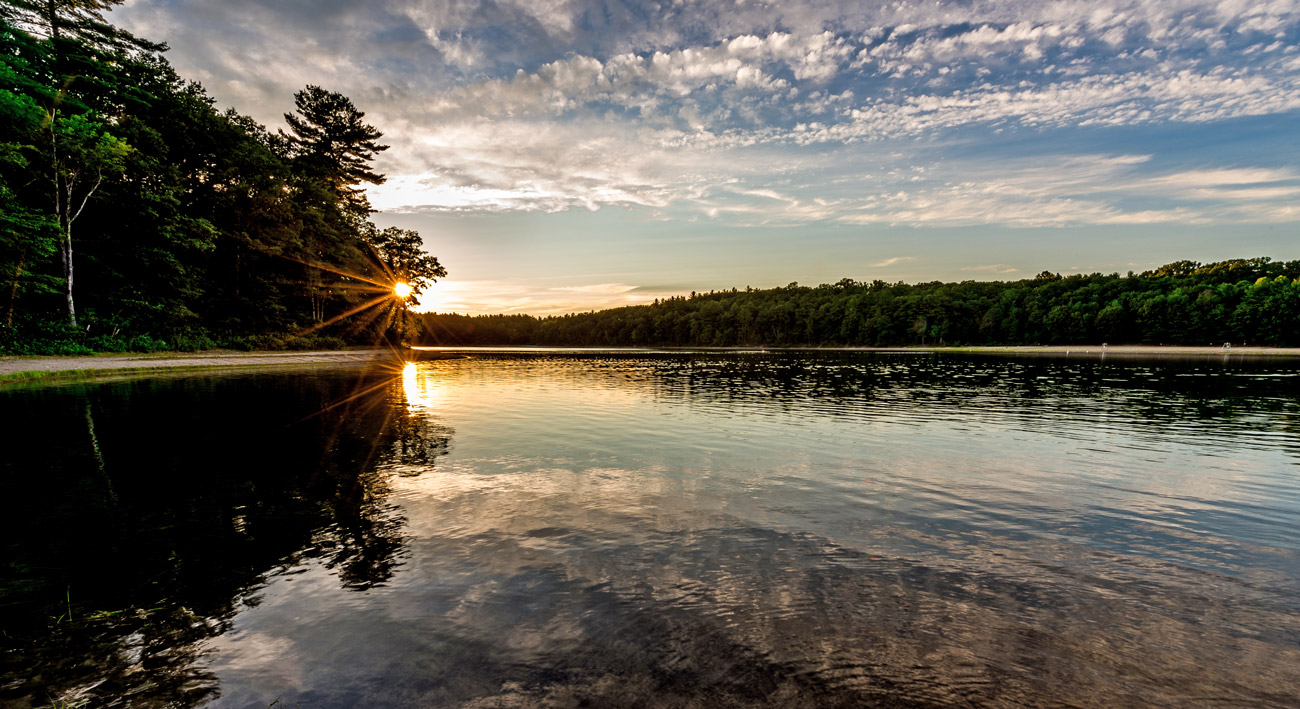
Walden Pond in Concord, Massachusetts.
Geoff Livingston
Recently, however, sporopollenin’s defenses may have been overcome. In 2018, Li and other researchers at the Whitehead, led by the plant biologist Jing-Ke Weng, published the first complete structure of sporopollenin. Subsequent work by the team, some of it not yet published, has filled in more details about how various groups of plants fine-tuned that structure to better meet their needs. Their proposed structure and the improved view of sporopollenin it offers is not without controversy, but it has clarified the molecule’s essential role in helping plants conquer the land.
The Inert Enigma
All seeding plants make pollen; other land plants, such as moss, produce spores. Carrying half the genetic information that the plants need to reproduce, pollen and spores move through the environment on the wind or on a helpful animal, to reach another plant of their species and fertilize its egg cell. But along the way, pollen and spores must contend with dangers that range from dehydration to the sun’s ultraviolet rays to hungry insects. Since plants first found purchase on land around 470 million years ago, keeping the genetic information within pollen and spores safe during their journey to fertilization has been vitally important.
The main strategy that plants employ to protect that DNA is to encase it in a specialized shell of sporopollenin, which is impervious to the elements and among the toughest materials produced by any living thing. It has been found intact in half-billion-year-old rocks. A 2016 paper found that because of the robustness of sporopollenin, spores maintained their stability in diamond anvils at pressures of 10 gigapascals, or 725 tons per square inch.
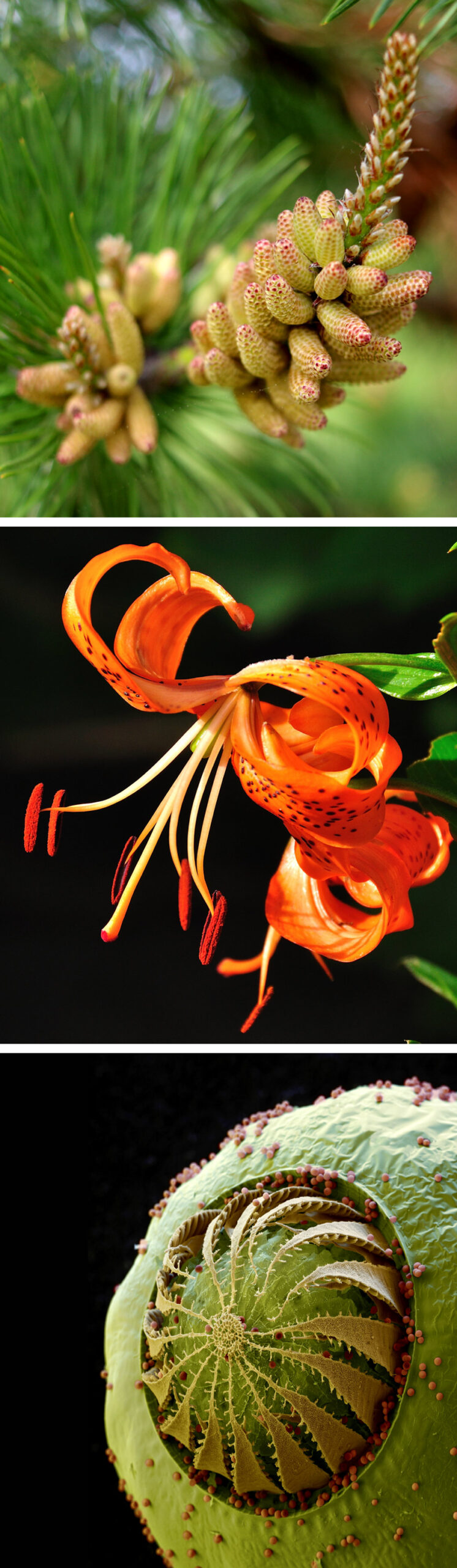
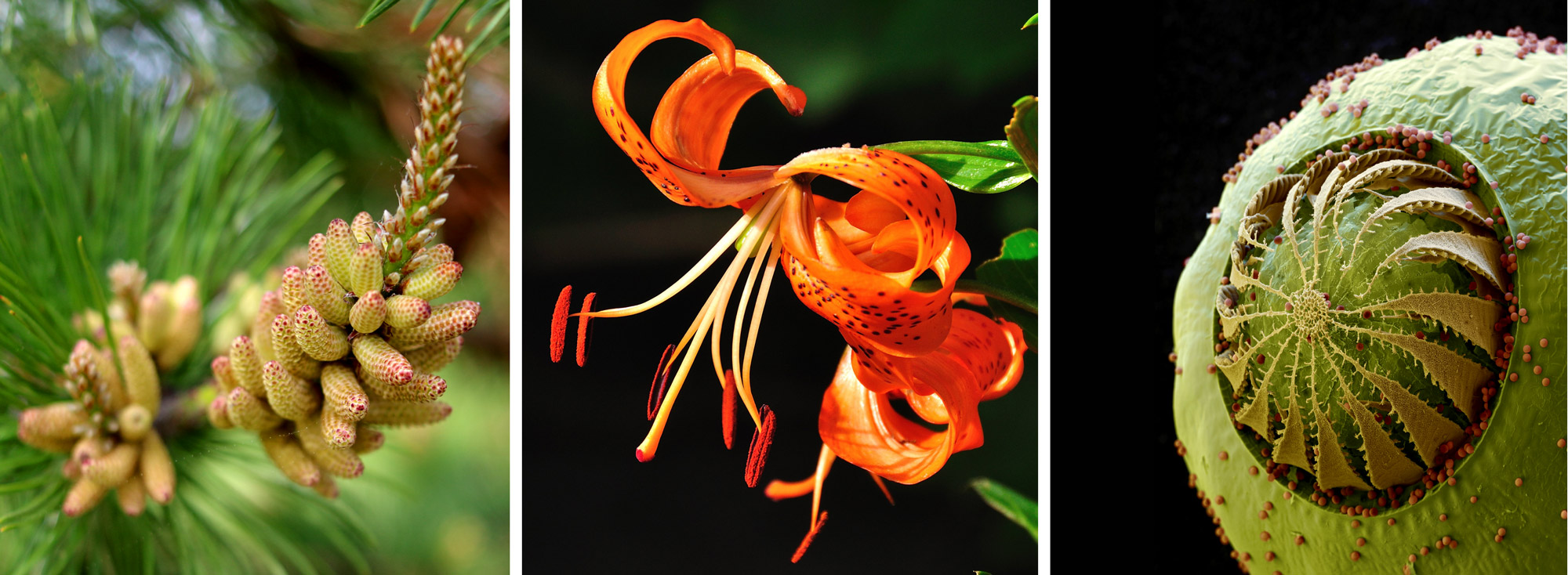
All sexually reproducing land plants have structures for releasing spores or pollen, such as (from top) the male cones on pines, the anthers on lilies and structures on club moss.
(from top) Dan Keck; Neil Roger; Eye of Science / Science Source
Sexually reproducing land plants have structures for releasing spores or pollen, such as (from left) the male cones on pines, the anthers on lilies and spore capsules on mosses.
(from left) Dan Keck; Neil Roger; Eye of Science / Science Source
Researchers have known and wondered about sporopollenin since at least 1814. They observed that even after the rest of a pollen grain or spore was chemically dissolved away, a strange substance always remained. For most of the next century, those studying it in spores and pollen worked separately, referring to it exclusively as either sporonin or pollenin. It was dubbed sporopollenin in 1931 to appease both communities.
For decades thereafter, knowledge about the molecule largely ended with the name. Researchers recognized that sporopollenin could be key to understanding how plants conquered nearly every habitat on Earth, and they dreamed of using the material for everything from coating the hulls of ships to protecting fragile proteins in oral vaccines. But getting the structure and chemical composition of sporopollenin was a prerequisite for any further work, and sporopollenin frustrated every effort.
Chemists usually determine the structure of a complex molecule by breaking it down into its constituent parts, finding the structure of those, then piecing them back together. But sporopollenin was too inert for the usual chemical agents to digest it. Starting in the 1960s, new biochemical methods and mass spectrometry made some progress on the structure and chemical composition, and biologists later even inferred some details from knowledge of the genes and enzymatic processes that synthesize sporopollenin.
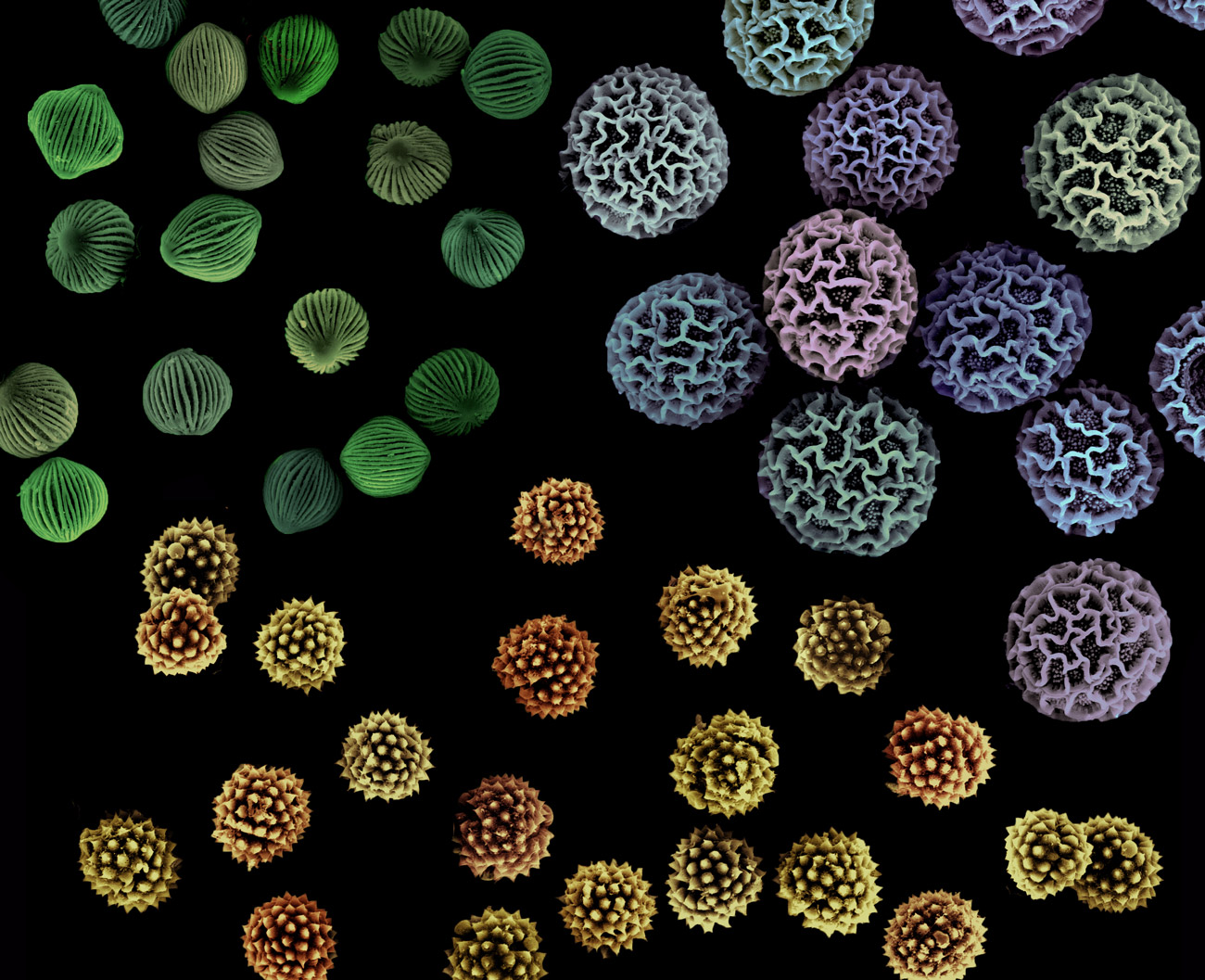
Pollen grains and spores are produced in a fantastic range of shapes and sizes, but all of them are encased in a protective shell of sporopollenin that helps them survive.
Asja Radja
None of these methods, however, could deliver a full picture of the molecule. Sporopollenin seemed to have two parallel backbones made of molecules called polyketides, not unlike the sugar backbones in the double helix of DNA. These backbones appeared to be connected by a weave of linkages of different types. But this sketch was incomplete, and some of the findings from the biochemical and genetic methods conflicted with one another.
“The only thing that everyone agreed on was the empirical formula for the composition of carbon, hydrogen, oxygen,” said Joseph Banoub, a professor of chemistry and biochemistry at Memorial University of Newfoundland in Canada.
Pitch Pine Perfect
Li started working on sporopollenin soon after he joined Weng’s lab at the Whitehead Institute as a postdoc in 2014. In the Cambridge neighborhood of Kendall Square, where biomedical research is the primary obsession, the lab is one of the few places where people study plants, with a research focus on the galaxy of botanical molecules that remain uncharacterized.
Sporopollenin was an irresistible challenge for Li. Its function was well known, and the genes for making it were in every seed- and spore-producing plant, which implied that sporopollenin was a basic adaptation enabling plants to live on land at the very beginning of their escape from the oceans. (Some species of algae also make a sporopollenin-like substance, which suggests that land plants adapted the biosynthesis of that molecule during their evolution.) Yet the chemistry behind that ability remained blurry.
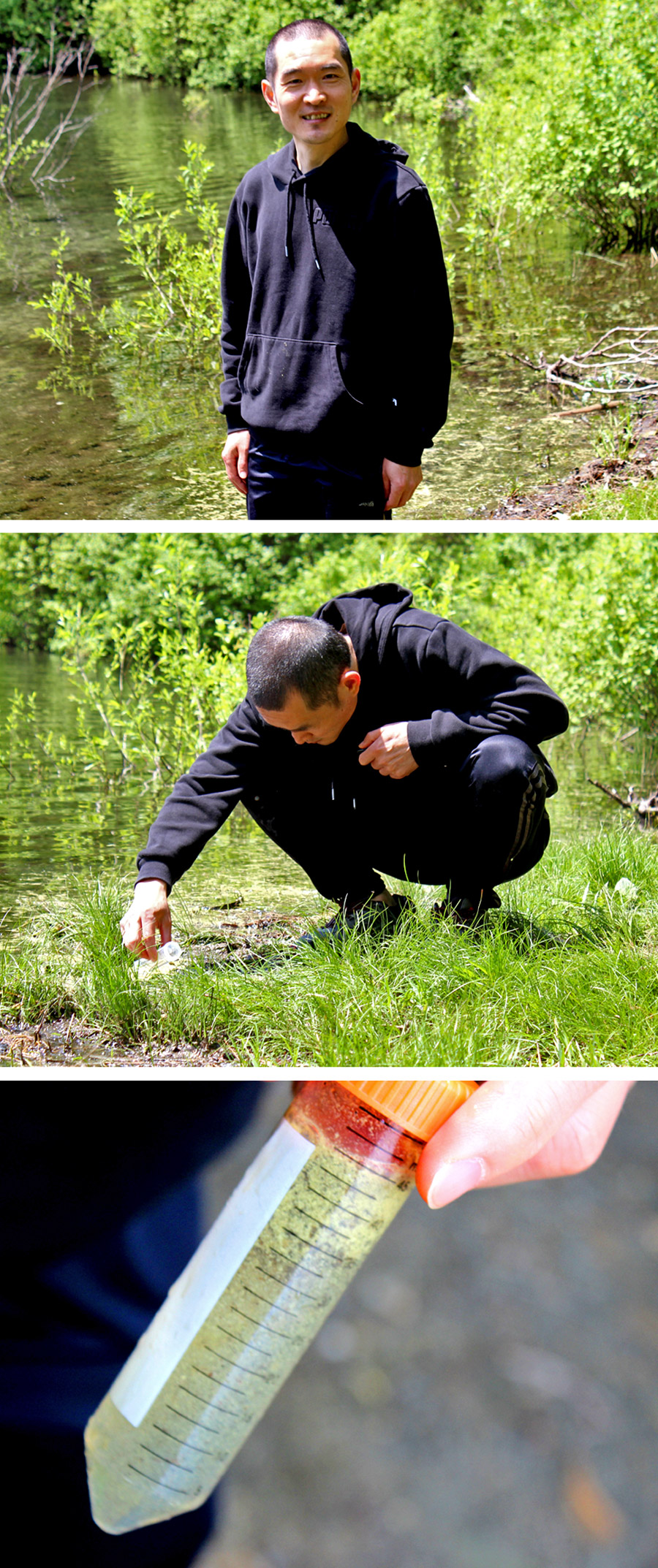
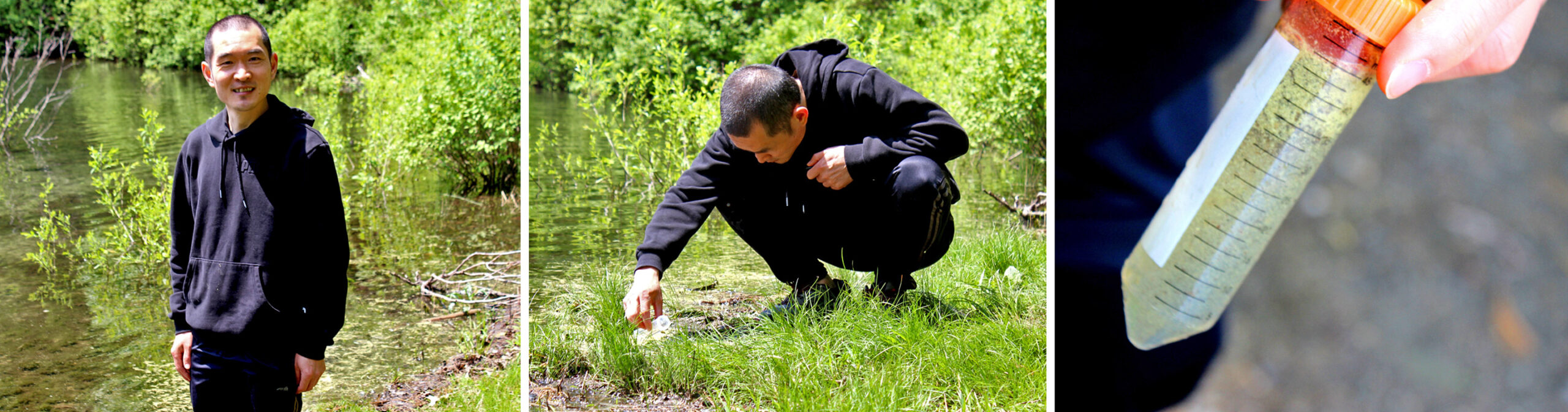
Fu-Shuang Li, a research scientist at the Whitehead Institute, went to Walden Pond to collect pine pollen for some of his experiments.
James Dinneen
It would have been poetic if Li’s early work on sporopollenin had used pollen collected from the waters of Walden Pond. But convenience trumped romance: The pollen his team initially studied was ordered from Amazon. (Pollen from pitch pine, which copiously produces the stuff, is widely sold as a health supplement.) The rest came from Cape Cod.
For months, Li and his collaborators ran trial-and-error testing on compounds that can degrade other tough biopolymers. Eventually, they developed a new multistep process that could take samples of pollen, pummel them in a ball milling machine, and chemically fracture the contained sporopollenin molecules. Half of each molecule broke down into six distinct pieces that could then be characterized by mass spectrometry.
The other half of the molecule, which they called the R group (for “recalcitrant”), only broke down when mixed with another dissolving agent. They could get a partial view of R this way, but the process degraded other features of the molecule, so Li’s group resorted to a more exotic technology, solid-state nuclear magnetic resonance spectroscopy, to characterize it.
Flowers Made a Difference
The fruit of that work, a paper published in Nature Plants in December 2018, proposed the most complete molecular structure of sporopollenin to date.
In conversation, Li used his hands to describe the intricate shape of the structure. With his thumb and forefinger, he showed how aromatic molecules hang off the backbone in alternating L-shapes. He demonstrated how the backbone is bound with the cross-linkages by pointing one flattened hand into the other at an angle, as if engaging in some strange form of prayer. These basic units link together to form the complete exine shell, which takes on radically different shapes in different plants, though the basic molecular subunits are fundamentally similar.
The structure gave credence to the idea that the hardiness of sporopollenin arises from the varied, braided linkages between the backbones. These ester and ether linkages are resistant, respectively, to basic and acidic conditions; together they resist both. The structure that Li’s group proposed also included several aromatic molecules known to be resistant to ultraviolet light, which accounted for sporopollenin’s ability to guard DNA from the elements.
“Without these metabolic innovations, plants would not have been able to migrate from water to land in the first place,” Weng wrote in an email to Quanta.
Merrill Sherman/Quanta Magazine
Recently, Li and his colleagues used their method to characterize sporopollenin from more than 100 diverse land plant species collected from botanic gardens around the northeastern United States. According to Li, who is preparing to submit the results of the study for publication, the structure of sporopollenin varies across plant types in a curious pattern.
They found that gymnosperms, the land plant group that includes cycads and conifers like pitch pine, and the so-called lower land plants like mosses and ferns tend to have long, similar sporopollenins. This makes sense because these plants disseminate their pollen willy-nilly on the wind; they need long-chain sporopollenin to protect it.
But among angiosperms, or flowering plants, the situation is more complex. Their flowers shade their pollen from sun and desiccation, and insects efficiently move pollen from flower to flower, minimizing the exposure to other risks. Consequently, angiosperms don’t need their sporopollenin to be so uniformly robust.
And making long-chain sporopollenin is an energy-intensive process, Li said, so “when flowers evolved, they did not want to produce pine-like sporopollenin anymore.” According to Li and Weng, significant differences seem to have evolved between the sporopollenins produced by the two major categories of angiosperms, monocots and dicots, which diverge in the structures of their embryos, vasculature, stems, roots and flowers.
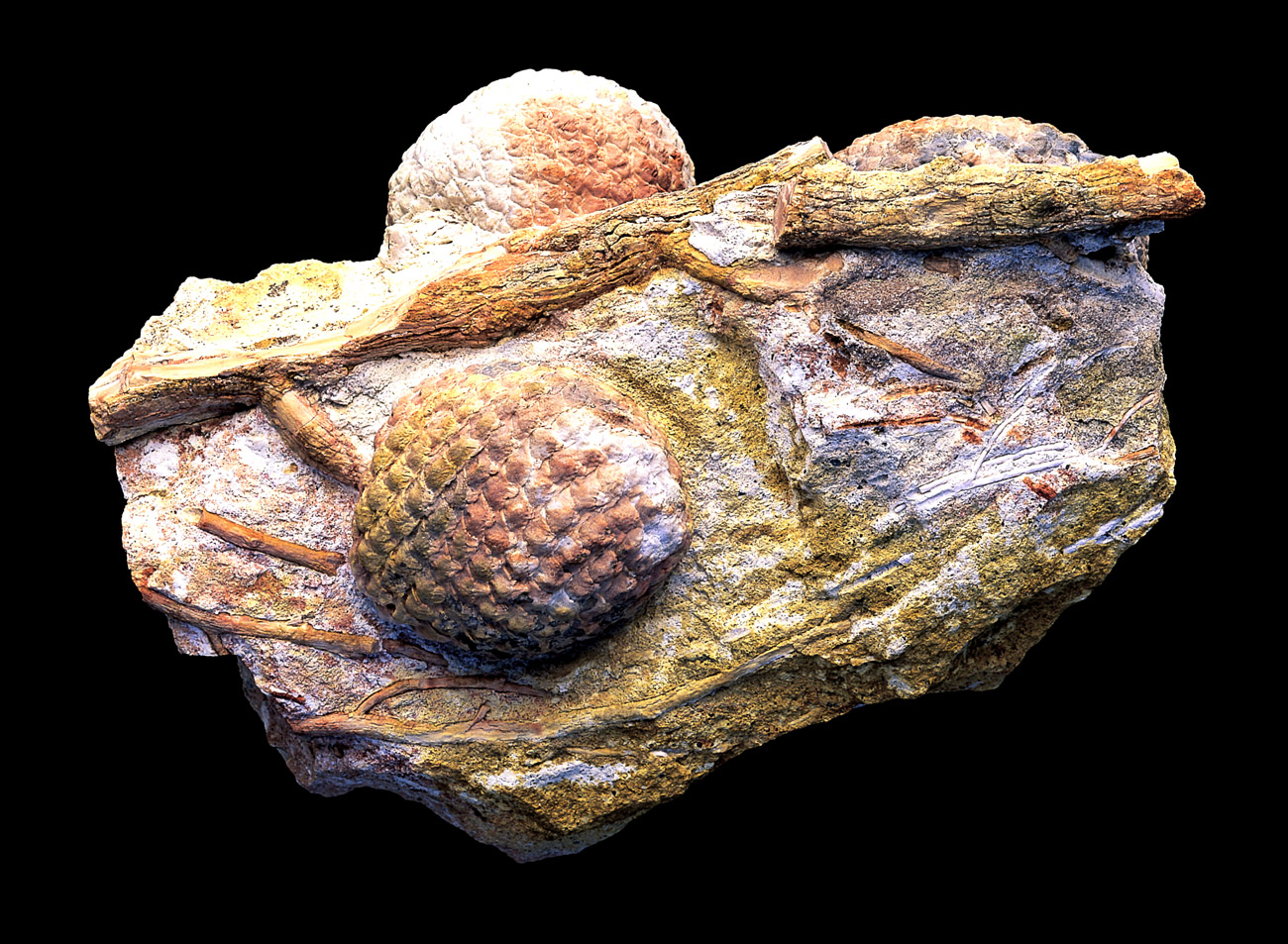
Fossils show that plants have been making spores and pollen ever since they first moved to dry land.
Francois Gohier / Science Source
Of course, the distinctions aren’t absolute. Some flowering plants do produce sporopollenin with a pine-like structure, said Li. “Maybe if we had another 6 million years, they may lose the function of those,” or maybe there are other ecological checks and balances at play preserving that sporopollenin structure for certain groups of plants.
“Evolution is not a line,” said Li. “Like the whales. At one point they lived on land; now they live in the ocean.” Yet whales still have some land animal characteristics. Perhaps some flower pollens retain obsolete traces of their own history.
The Mysterious Polymer
Other plant researchers agree that Li and Weng’s structural work on sporopollenin has improved our knowledge of the molecule. But not all of them are persuaded that their proposal is correct or that it concludes the century-long search for the structure of sporopollenin.
“It was much clearer than before,” said Zhong-Nan Yang, a biologist who studies sporopollenin at Shanghai Normal University. “But it needs to be verified.” He said Li and his colleagues still must identify the genes responsible for the enzymes needed to make certain features of pine sporopollenin.
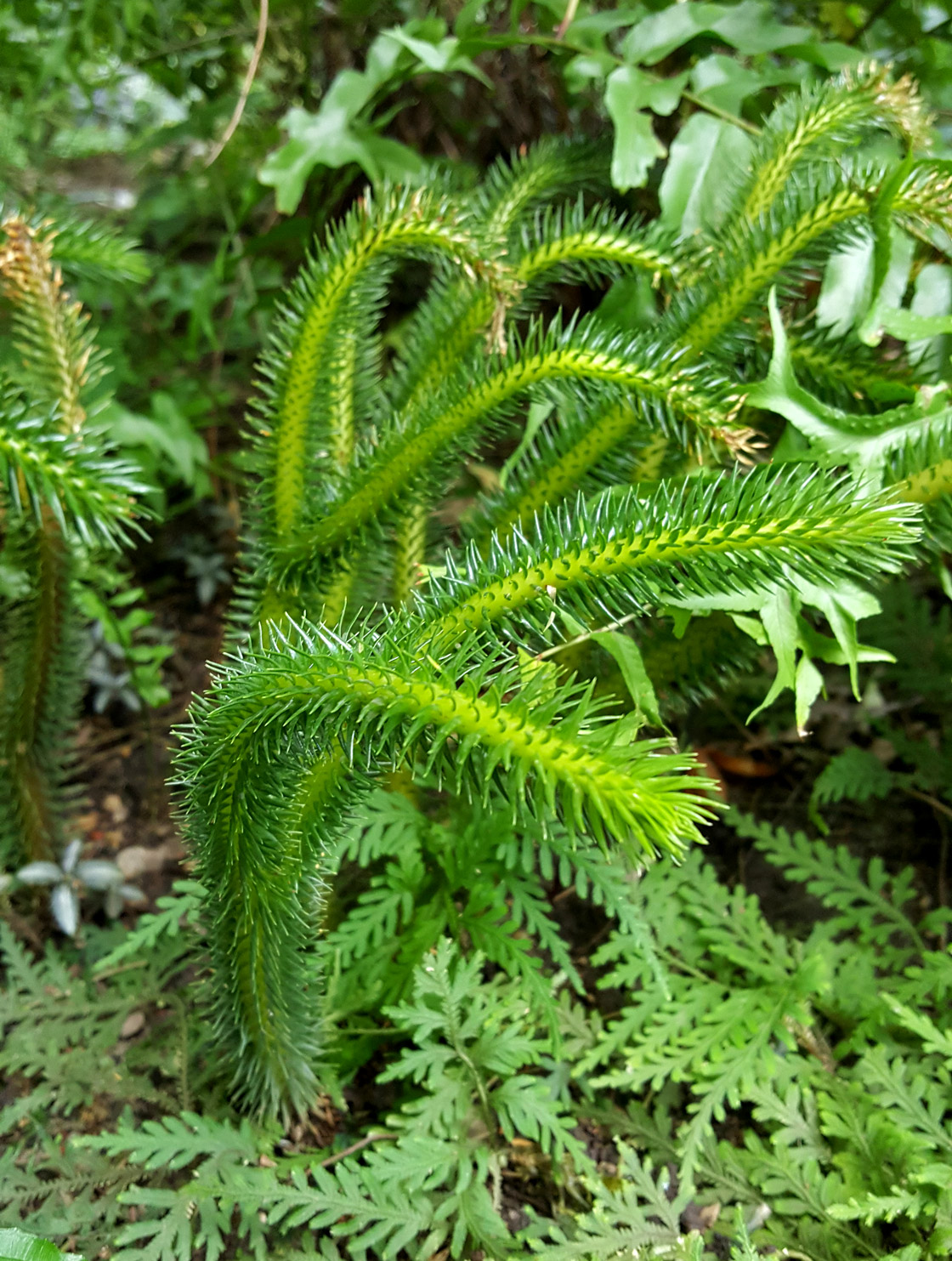
The structure of sporopollenin in club moss pollen has been reported to be different from that of pine pollen sporopollenin, but scientists still disagree about the reasons for that difference.
Protopian Pickle Jar
A 2020 study aimed at “demystifying and unravelling” the molecular structure of sporopollenin posed a more direct challenge. Using a bevy of methods and working on sporopollenin from club moss rather than pine, Banoub’s group at Memorial University arrived at a structure that differed in several important ways from the one proposed by Li and Weng. Most importantly, Banoub said, “We have proved there are no aromatic compounds within the sporopollenin.” The disparity, he thinks, might be explained by differences between sporopollenin in pine and club moss.
“My personal view is they are not correct,” said Li, but he prefers not to comment further until some relevant results from his lab are ready for publication.
“It is still quite the mysterious polymer,” commented Teagen Quilichini, a plant biologist at Canada’s National Research Council who has studied sporopollenin, in an email. “Despite what some reports suggest.”
Tough but Still Edible?
Notwithstanding the controversies over their structure for sporopollenin, Li and others in the Weng lab have moved on to another evolutionary question: Has nature figured out how to take apart this nearly indestructible material it put together?
As he hiked around Walden Pond in search of other pollen-coated inlets, Li compared sporopollenin to lignin, the plant polymer that strengthens wood and bark. After woody plants first evolved about 360 million years ago, the geological record shows an abundance of fossilized lignin in strata for tens of millions of years. Then suddenly about 300 million years ago, the lignin vanishes. Its disappearance marks the moment when a fungus called white rot evolved enzymes capable of degrading lignin and ate much of it before it could fossilize.
Sporopollenin, Li reasoned, must also have a fungus or other microbe capable of breaking it down. Otherwise we’d be drowning in the stuff. Li’s back-of-the-envelope calculations are that 100 million tons of sporopollenin are produced in forests every year. That doesn’t even account for the sporopollenin produced by grasses. If nothing is eating it, where does it all go?
This is why, as a source for his latest sample of pollen, Li opted to forgo Amazon Prime in favor of a day at Walden Pond. Observations by his team suggest that some microorganisms grown in petri dishes can survive when fed nothing but sporopollenin and nitrogen. Samples from Walden, which are naturally full of lake microbial communities, should help Li determine whether populations of fungi and other microbes in the wild can unlock the nutrients in sporopollenin’s seemingly unbreakable molecules.
As we snacked on seaweed and granola bars by the pond’s edge, it was easy to see the whole situation from the fungi’s perspective. Nature hates to waste a meal — even one so tough to chew.
Correction: July 20, 2022
The grain of pollen in the opening micrograph was originally misidentified as olive. It is radish.