To Heal Some Wounds, Adult Cells Turn More Fetal
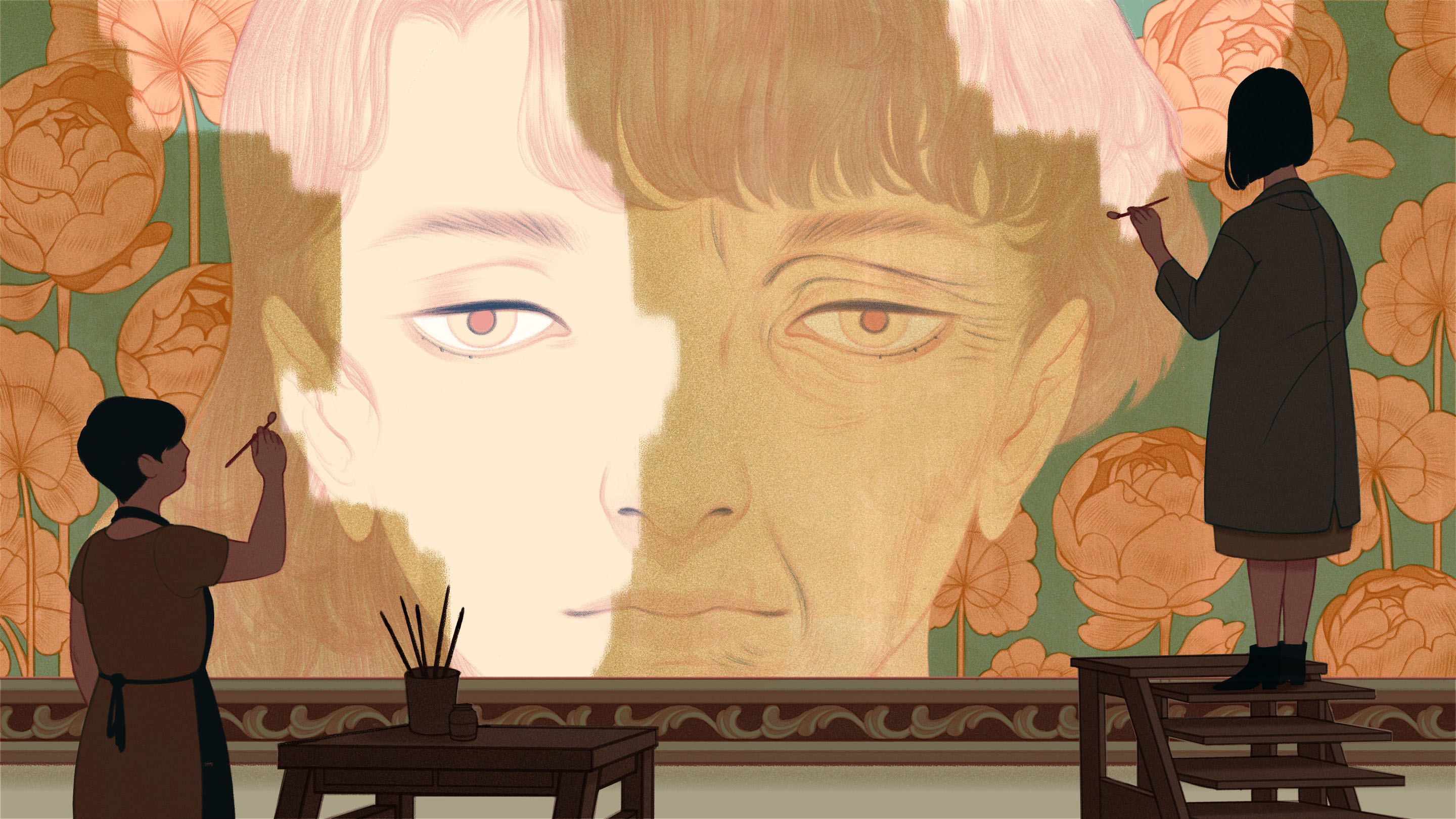
To repair and restore themselves after damage, body tissues need new cells. To get them, researchers are discovering, tissues sometimes recruit ordinary mature cells and revert them to a highly proliferative state usually associated with fetuses.
Rachel Suggs for Quanta Magazine
Introduction
An embryo starts out as just a single cell. It’s not long before it divides into two cells, then four, then eight, and so on — a process marked by rapid growth, in which these early, unspecialized cells proliferate wildly to start building all the tissues of the body. As development proceeds, these embryonic (and later fetal) stem cells become more specialized, differentiating into the precursors of various cell lineages, which in turn give rise to more mature cells: blood cells, nerve cells, muscle cells, intestinal cells. Major functional changes in these tissues continue to take place after birth, as the organism adapts to life outside the uterus, for the first time using its lungs to breathe air and its digestive system to process food.
A few cell populations retain some of that early plasticity as adult stem cells, helping both to maintain tissues on a day-to-day basis and to heal wounds. In recent years, moreover, it’s become clear that those aren’t the only cells that stay flexible: Sometimes, when the repair process calls for it, more specialized cells can take a few steps back, or “de-differentiate,” to re-enter a stemlike state, too.
But new results suggest that that plasticity may go even deeper than scientists have thought. Three research teams have observed that during tissue regeneration, the typical solutions offered by adult stem cells (and the de-differentiated cells resembling them) aren’t enough. Instead, the cells of the damaged tissue turn the clock back all the way to a more fetal state, tapping into the proliferative power that once characterized development — and a program thought to have long gone silent.
Atom Bombs and Self-Renewing Cells
In the early 1900s, scientists theorized that the specific blood cell types they’d learned to distinguish from one another under a microscope — red blood cells, white blood cells and platelets — came from a common, more primitive source: a stem cell. But it wouldn’t be until the 1950s and ’60s that researchers could offer definitive proof of their existence and begin to delineate their unique properties.
The discovery of the first stem cells came about indirectly from the atomic bombings of Hiroshima and Nagasaki in 1945. Medical workers observed that exposure to radiation caused a precipitous drop in the survivors’ white blood cell counts, and experiments in mice showed that bone marrow transplants could offset those losses. Work over the following decades gradually revealed why: A population of cells in the marrow could both self-renew and differentiate into various, more specialized blood cell lineages. These were the blood-making stem cells.
They departed from the behavior of more specialized cells in several key ways. When a differentiated cell divided, it produced two copies of itself — and depending on the cell type, the number of times it could do so was limited. That wasn’t the case with the stem cells isolated from the bone marrow. When they divided, they did so over extremely long periods of time, in a process known as proliferation. Moreover, those divisions were asymmetric: Each stem cell produced not only a copy of itself but also a daughter cell fated to become a specific type of blood cell. For those daughter cells that gained a differentiated identity, there was generally no going back.
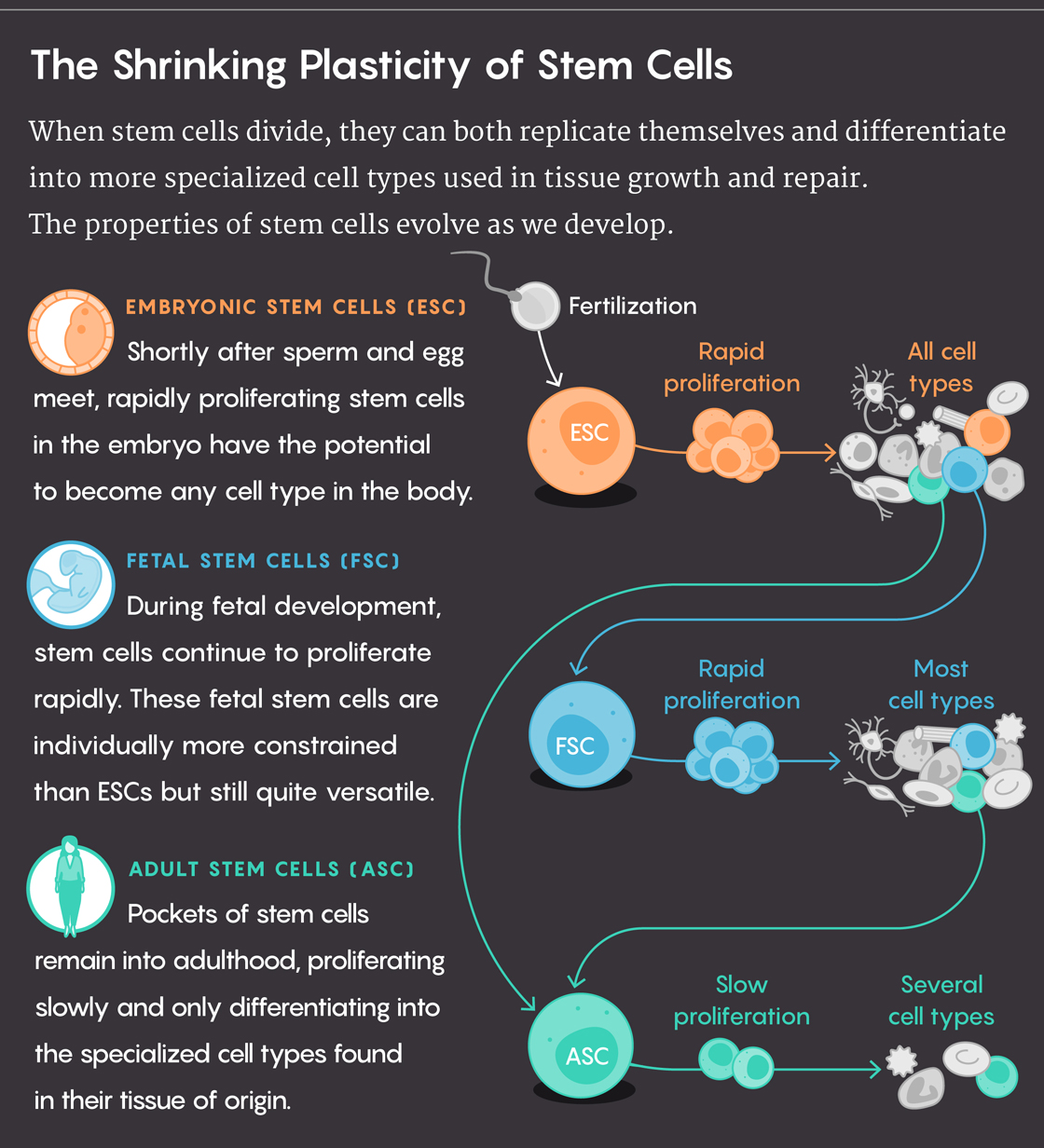
Lucy Reading-Ikkanda/Quanta Magazine
As stem cell populations were later found in other organs as well, that “paradigm … serve[d] as a template to interpret experimental observations on any other mammalian tissue,” Hans Clevers, a molecular geneticist at the Hubrecht Institute in the Netherlands and one of the world’s top experts on stem cells, wrote in 2015. But that wasn’t necessarily a good thing. “Attempts to fit observations on solid tissues into the [blood stem cell] hierarchy mold,” Clevers continued, “have led to confusing theories, terminologies, experimental approaches and heated debates, many of which remain unresolved.”
The Plasticity of Everything
Still, by the time Clevers penned those words, the conception of what it meant to be a stem cell was already undergoing a massive overhaul. In the late 1990s, stem cells from human embryos were isolated and cultured for the first time, revealing that unlike adult stem cells, which could give rise only to cell types found in their tissue of origin (a blood stem cell in the bone marrow might generate a neutrophil, for instance, but wouldn’t differentiate into a nerve cell in the brain), embryonic stem cells harbored the potential to become any cell type in the body.
Meanwhile, adult stem cells found in tissues other than bone marrow didn’t always seem to act similarly to the blood stem cells. Ones discovered in the intestine and characterized throughout the 1990s and 2000s indicated that certain stem cell populations could replicate far more vigorously than those residing in the bone marrow, and could sometimes divide symmetrically. Several organs, including the pancreas and kidney, didn’t seem to have populations of cells that functioned exclusively as stem cells at all — implying that other cells in those tissues might have to assume stemlike duties in certain cases. In Clevers’ words: “The search for stem cells as a physical entity may need to be replaced by the search for stem cell function.”
The real turning point in demonstrating clear evidence of such plasticity came in 2006, when Shinya Yamanaka and Kazutoshi Takahashi at Kyoto University in Japan took connective tissue cells from adult mice and, by introducing just four genes to them, succeeded in essentially wiping the slate clean and transforming them into embryonic-like stem cells. (The work eventually won Yamanaka a Nobel Prize.)
Scientists rapidly followed up with investigations into whether this might be occurring naturally, too. It certainly seemed to happen in the formation of tumors — cancers have stem cells, as well as differentiated cells driven by mutations to a more stemlike state — but could such a process also represent something ordered, something healthy? The answer turned out to be yes. Throngs of cell types throughout the body — in the skin, in the lung, in the stomach — can de-differentiate when exposed to an injury that causes inflammation and damage to normal stem cells. While cells that have differentiated more recently are particularly prone to regaining their stem cell origin in these situations, research is also beginning to show that cells even further down the specialization path can go back.
Just yesterday, a group of researchers led by Riccardo Fodde, a geneticist at Erasmus University Medical Center in the Netherlands, reported that one such cell type — the paneth cells in the intestine, which secrete molecules that control the gut’s bacterial composition and digestive health — loses its normal gene expression patterns in favor of stemlike ones after injuries. These cells do not normally divide at all, but once they are coaxed into this stemlike state, they proliferate rapidly like stem cells, giving rise both to copies of themselves and to more differentiated cells.
Similar results have been demonstrated in other cell lineages as well. Some labs are even trying to capture cells in the act of de-differentiating. “Our cells are much more plastic, much more capable of responding to injury, than we ever thought,” Fodde said. So much so, added Simon Buczacki, a cancer researcher at the University of Cambridge, that “what everyone is saying at the moment is that everything is plastic, everything can become a stemlike cell if it’s pushed.”
Return to a Fetal State
But what did that transition look like at a more molecular level, exactly? Particularly given how complex the concept of a stem cell has turned out to be, what did the “stemlike” state of a de-differentiated cell really entail?
A few recent papers, one published in 2016 and two others within the last year, provide what many researchers consider to be compelling evidence that at least some differentiated cells can transiently express a developmental gene program that rewinds not just back to an adult stem cell state but all the way back to a state similar to that of a developing fetal organ.
In retrospect, the findings might not come as too much of a surprise: Researchers who study salamanders and other amphibians, the paradigms for tissue regeneration, see this happening on a far grander scale all the time. Those organisms can regrow entire limbs — bone, muscle, cartilage and all — by recapitulating a developmental program from a bud-like structure that forms on the injury site. But humans and most animals aren’t capable of this kind of tissue generation.
At most, scientists have hypothesized that the de-differentiation process implicated in both tissue regeneration and cancer involves the activation of some sort of embryonic or developmental pathway. But studies of embryonic gene activity in such cells that could support that conjecture have had mixed results. “It’s an attractive idea,” said Andrew McMahon, a stem cell researcher who studies the kidney at the University of Southern California. “But frankly, the evidence for that isn’t there.”
Part of what is significant about the newest findings, then, is that they suggest researchers studying regeneration in humans and other animals might have been looking for the wrong signs: Rather than embryonic genes, perhaps they should have been searching for the fetal markers that emerge a little later in development.
That’s not what Richard Locksley and Ophir Klein, researchers at the University of California, San Francisco, initially set out to do. Locksley, an immunologist seeking to gain a better understanding of allergies and the immune system, wanted to track the role stem cells played in the intestine’s response to damage from parasitic worms in mice.
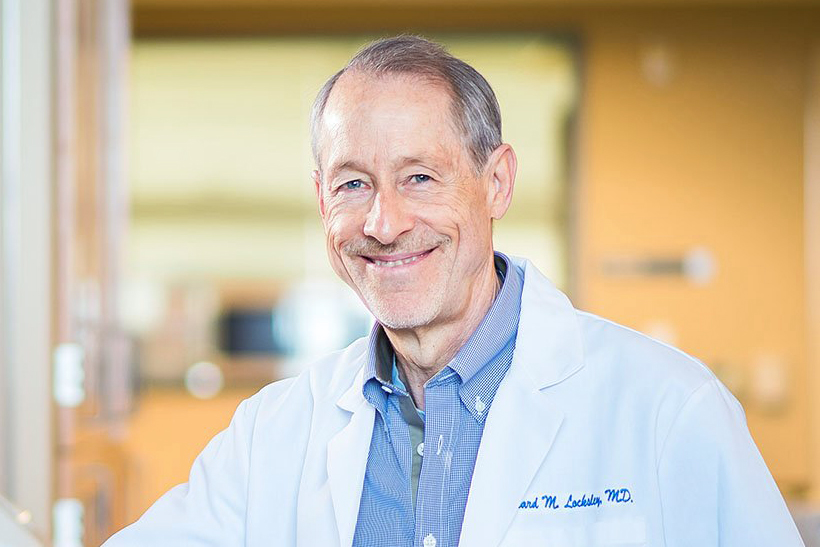
Richard Locksley of the University of California, San Francisco, observed a new kind of wound healing in the intestines of mice. In response to damage by parasites, the intestine’s adult stem cell program switched off in favor of a more fetal one.
Courtesy of Richard Locksley
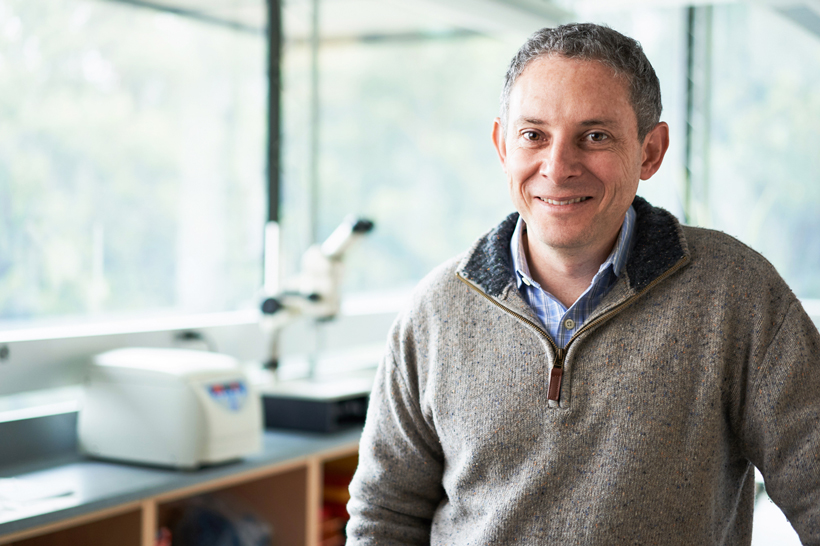
Ophir Klein, PhD, MD, chair of the Division of Medical Genetics in his lab at UCSF Parnassus.
Steve Babuljak
Richard Locksley (left) and Ophir Klein (right) of the University of California, San Francisco, observed a new kind of wound healing in the intestines of mice. In response to damage by parasites, the intestine’s adult stem cell program switched off in favor of a more fetal one.
Courtesy of Richard Locksley; Steve Babuljak
“But we quickly saw the whole thing go sideways,” Locksley said. He’d expected stem cells located close to where the worms had burrowed into the tissue to become more active, generating new lineages and making the necessary repairs. Instead, the genetic markers used to identify those stem cells disappeared entirely. Yet, even with that population depleted, the cells around the wounds began dividing more rapidly than usual. “It suggested that maybe the cells had shifted into a new, injury-responsive state,” said Ysbrand Nusse, the lead author of the Nature paper the team published in June about these findings.
It would take years for them to figure out what was controlling that shift. Locksley started collaborating with Klein, his UCSF colleague and a stem cell biologist: The unanticipated result had piqued his interest. They found that a particular immune response was activated, and that the gene Sca-1 was being expressed at high levels in the damaged tissue. When cultivated in a dish, those Sca-1 cells formed blobs of tissue that looked more fetal than adult — a connection made possible only a few years earlier, when scientists first started describing the development of the fetal intestine in molecular terms.
Klein and Locksley’s team found that a slew of other genes expressed during development had been transiently reactivated. The same cellular reprogramming occurred after irradiation and other kinds of injuries, too. In response to various types of damage related to inflammation, then, the cells seemed to be invoking some kind of fetal memory (though the researchers are careful to point out that this doesn’t represent a complete return to a fully fetal state). That implies that “adult cells can reactivate the same pathways that are normally used during the patterning of the tissue in the first place,” said Kelley Yan, a gastroenterologist at the Columbia University Irving Medical Center in New York, who was not involved in the study.
Two other research groups have uncovered a very similar phenomenon. In 2016, a team led by Marie-Isabelle Garcia, a biochemist at the Université Libre de Bruxelles in Belgium, published work in Development showing that injured stomach tissue in mice re-expressed a protein marker identified previously in progenitor cells from the fetal stomach.
More recently, researchers led by Kim Jensen, a molecular biologist at the University of Copenhagen, found further support for this process in the colon of mice with inflamed bowels. Not only did the regenerating tissue express the Sca-1 marker and other signatures of a fetal program, but Jensen and his colleagues also implicated a potential mechanism: They reported in Cell Stem Cell last December that mechanical forces from the extracellular matrix surrounding and supporting the cells activated a signaling pathway that initiated the repair. The matrix stiffened — something Jensen is now hoping to show is also indicative of a fetal state.
These results “point to this being a generalized process when there’s been an inflammatory or lesion-like injury,” Garcia said. “It’s like a security system” that the body has left in place since its developmental period.
A Real Estate Problem
That makes sense, according to Locksley, given how that system most likely functions. “Fetal systems very quickly build real estate,” he said. “You just want to lay out the organ: where the streets go, the conduits of electricity, the plumbing.” In repair, too, the name of the game would be about quickly amplifying the number of cells and making them as flexible and mobile as possible. All that matters is patching up the breach to the tissue. It’s like “throwing asphalt on top of a hole,” Locksley said, positing that it’s the most energetically efficient way to repair damage, whether in the myocardium after a heart attack or in the skin after a major burn. “If there’s a way to access an already less-costly way to cover the real estate, then who cares if you make a heart using fetal heart cells? You need it rebuilt first, and can let it differentiate later.”
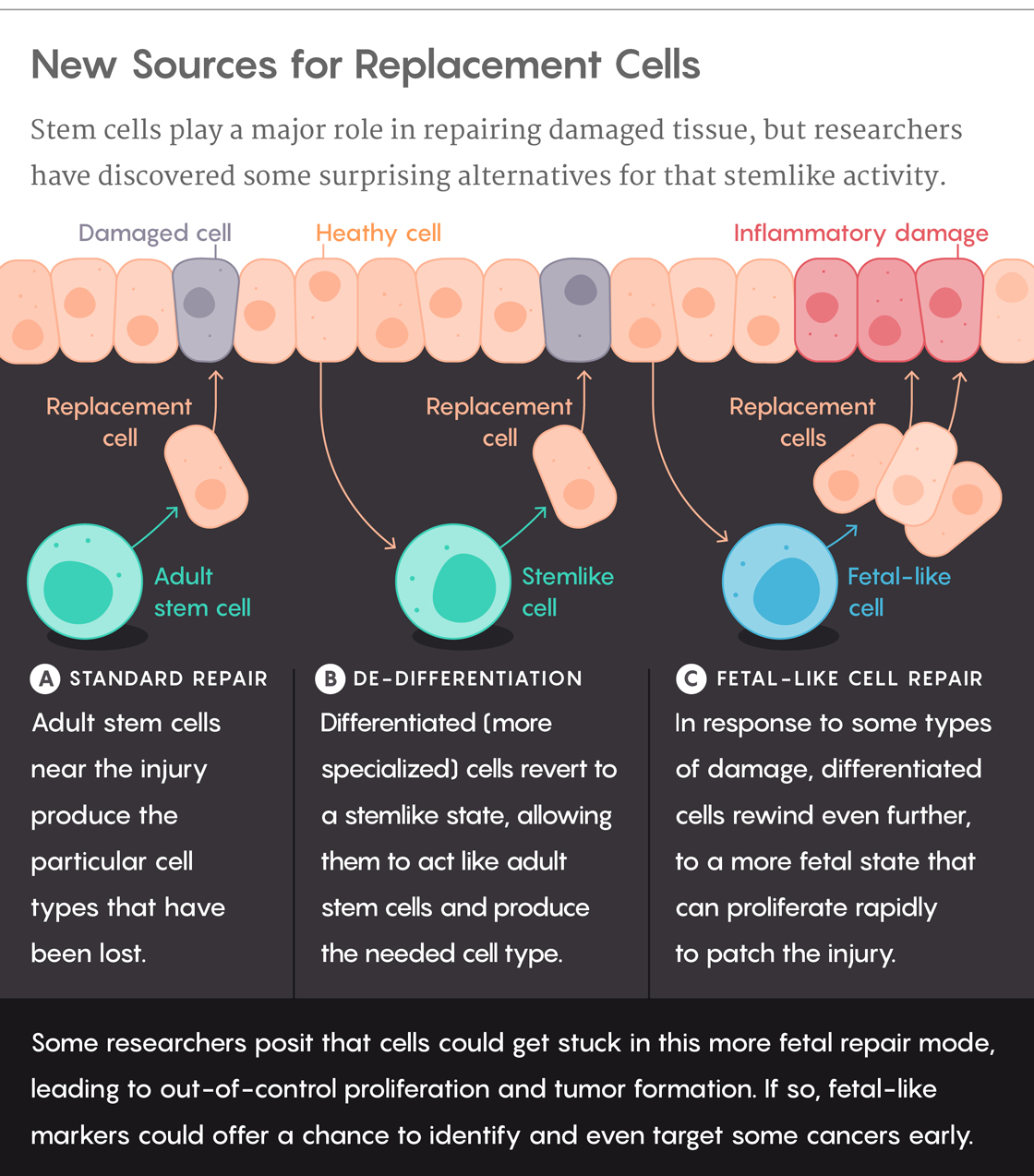
Lucy Reading-Ikkanda/Quanta Magazine
“This happens again and again in biology,” Nusse added. “Certain principles get used over and over and over again in different contexts. So it would make sense that to repair the tissue, you’d use the same pathways and patterns that were used to initially build that tissue.” Amphibians quite clearly do that. Now, “it seems that something sort of like that, in a primitive way, is happening in the intestine” of mammals, too, Nusse said.
Unfortunately, experts posit that cancer — which is “basically a disease of chronic inflammation,” Buczacki said — can develop when something goes awry during this regenerative process. When mature cells revert back to a long-lived, proliferative state like that of fetal cells, their opportunities to acquire mutations increase. Perhaps one or more of those mutations might eventually cause the cells to get stuck in their more fetal repair mode, preventing them from differentiating back into their specialized identities and leading to the out-of-control proliferation characteristic of tumors.
There’s some support for that theory: Cancer risk increases in patients with inflammatory bowel disease and certain types of physical trauma.
Now, other experts who have been studying cells throughout the body capable of de-differentiating during regeneration or cancer can return to their data, this time with the express goal of uncovering whether a more fetal state was invoked to facilitate the process. “I don’t think they’ve looked for fetal markers, but I would bet they’re there,” said Jason Mills, a biologist at Washington University in St. Louis. “I don’t think anybody’s made the connection yet.”
A New Cellular Process
In fact, Mills is one of the leaders of a recent effort to cement that connection: He’s working to bring together experts on regeneration and cancer in different organs, in hopes of digging deeper into the potentially conserved mechanism underlying the de-differentiation process. (He’s planning the first conference on the topic, which will meet in Colorado in January.)
“The further back you go toward an embryonic state, the more cells start to look alike,” he said. “So we could find global markers of regeneration or cancer by examining this process.” Mills likens it to other conserved cellular processes, like mitosis (cellular division) and apoptosis (programmed cell death). In the same spirit, he and his colleagues coined a word for de-differentiation events in tissue repair and cancer: “paligenosis,” or “a return to a generative state.”
In a paper published in February in The EMBO Journal, for instance, his team outlined how cells can revert to a more primitive, rapidly dividing state through what seems to be a conserved sequence of pathways. Gastric chief cells in the stomach and mature acinar cells in the pancreas, which both perform secretory functions in their respective tissues, undergo similar changes in response to injury: They lose the same markers, express similar genes and become smaller and more similar to embryonic cells. In both cases, the cells first degrade the features that characterize their differentiation, then reallocate their energy to support replication rather than secreting behavior. These cells have also been implicated as cells of origin in stomach and pancreatic tumors, which is consistent with the idea that cancer may be the result of paligenosis gone wrong. Mills and others have identified a few of the genes and pathways involved, but there’s still much more work to be done.
Until now, Mills said, he has mostly been focused on the cell biology and the structural and signaling mechanisms in each cell, “not the fetal side of things.” As he and his colleagues seek to more fully characterize the process of paligenosis, work like that done by Locksley and Klein can guide them toward new places to look.
There’s still a long way to go toward cementing these ideas, of course. Klein, Locksley, Jensen and Garcia, for instance, need to figure out what triggered the fetal-like response they observed in their various experiments — whether it comes from a specific cell type or all types, and how related those instigating cells are to the original stem cells that got suppressed. Garcia is particularly interested in what happens after these events: how the re-differentiation phase of regeneration works.
They’ll also have to find out how this all works in humans, not mice, and definitively test the functional necessity of the reversion. It makes sense that it’s to cover a region with cells as quickly and efficiently as possible, but the researchers need to prove it.
After that, it should become possible to distill the mechanisms employed throughout the process, and perhaps eventually exploit them for growing better tissues in the lab and learning ways to prompt healing after the onset of an injury or disease. It’s something to add to the “toolbox in which you can promote repair or prevent that damage from occurring in the first place,” Yan said. “As a physician-scientist, that’s what interests me.”
Correction (August 30, 2018): In a previous version of this article, the credit for the photograph of Jason Mills by Indira Mysorekar was misattributed to Matt Miller.