In the Ticking of the Embryonic Clock, She Finds Answers
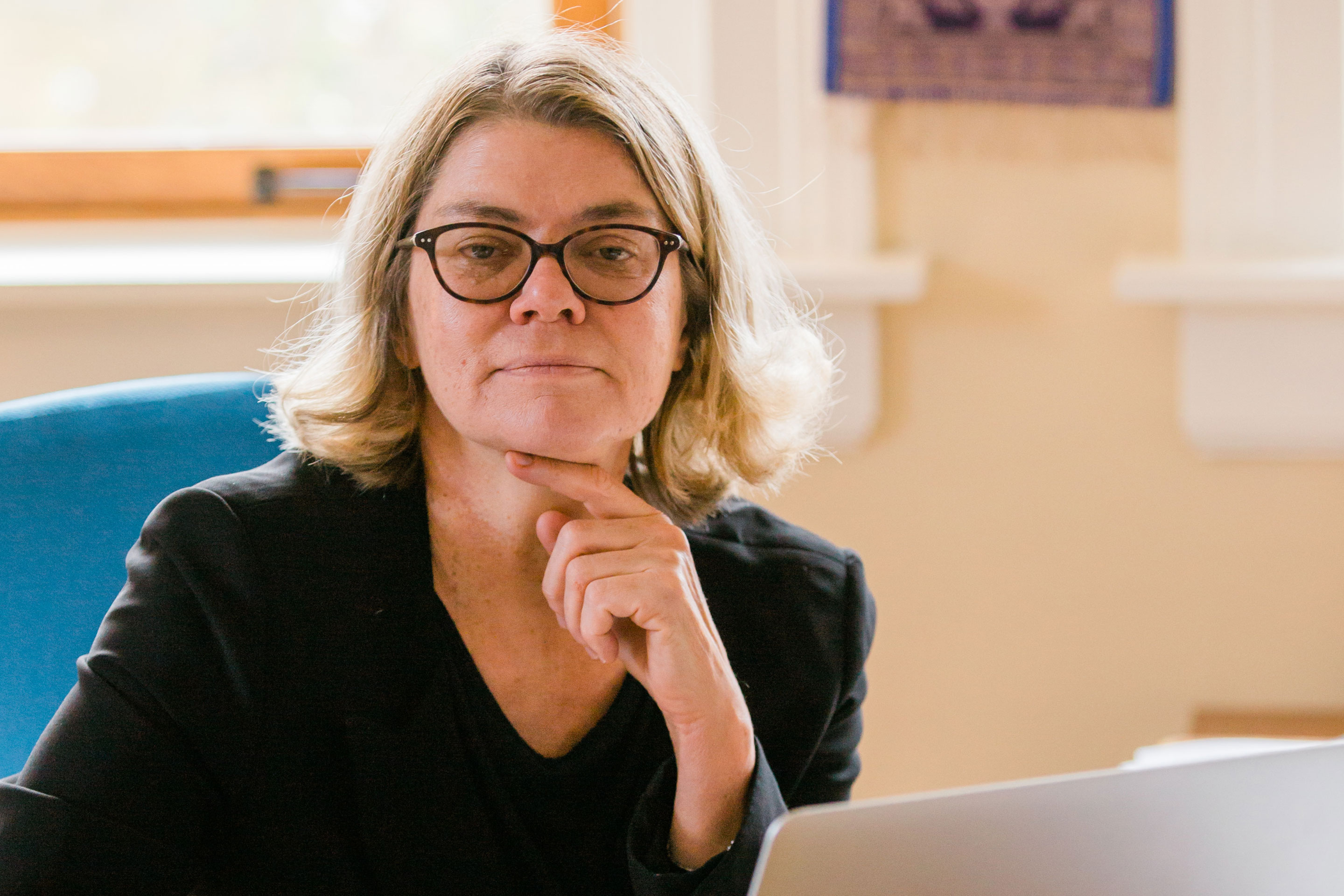
The stem cell biologist Renee Reijo Pera in her office at Montana State University, where she is also vice president of research and economic development. She has dedicated her career both to uncovering the intimate link between the timing of events in early embryos and later health, and to encouraging scientists to share their own stories as part of their work.
Kristine Paulsen for Quanta Magazine
Introduction
“Do you know anything more fascinating than when a sperm and an egg come together to make a human?” the stem cell biologist Renee Reijo Pera asked, with genuine incredulity, as she delivered a keynote address to hundreds of scientists at the Society for Developmental Biology’s annual conference in July. After decades of studying the subject — the genes and other information responsible for the emergence of germ cells, the carefully orchestrated molecular balancing act that produces them, the factors that predict an embryo’s health — her awe has only grown. “It reminds me of the Big Bang,” she later explained: So many conditions had to be just right, and with every answered question, it was always possible to take another step back and ask, “But how did that happen?”
“It’s kind of surprising,” she said. “We really don’t know very much.”
In her efforts to illuminate the bigger picture, a common theme has emerged: Something as deceptively simple as timing during the earliest stages of development can have an enormous impact on health and disease, even decades after birth. More recently, she’s used that insight to expand the scope of her work — investigating how, for instance, cell fate decisions in the embryo might contribute to the causes of neurological diseases like Parkinson’s.
But today, Pera spends more of her time outside the lab, devoting herself to a very different challenge. “Can we bring science to the masses?” she asked the conference audience from the podium. To do so, she said, “we need to tell stories” — stories that entwine the scientists themselves with their work, stories that make them vulnerable, easier to identify with.
Her own path into science, she said, was something she would never have predicted. She grew up poor in a small Wisconsin town, the youngest of six children and the first in her family to finish a four-year degree. Her mother worked several jobs to singlehandedly support her and her siblings, and her rarely present father was a former medic who contended with alcoholism and homelessness throughout most of her childhood; both died while Pera was still in her 20s. She ended up pursuing a career in science almost by accident, but went on to become an expert in embryonic stem cells and fertility, and director of Stanford University’s Center for Human Embryonic Stem Cell Research and Education.
Yet in 2013, she decided to leave all that behind to become vice president of research and economic development at Montana State University, where she feels she can have a bigger impact in science education.
Quanta Magazine recently spoke with Pera about her exploration of the link between timing, cell fate and health; the surprising role retroviral DNA plays in early development; and her hopes for the future of science education. The interview has been condensed and edited for clarity.
You grew up in the small town of Iron River, Wisconsin. What was that like, and what ultimately led you to a career in science?
Iron River is a town of some 1,000 people — even fewer when I was a child — located around 20 miles from the south shore of Lake Superior. And as in many small towns in the United States, kids go to grade school and high school, but there isn’t much aspiration when it comes to college or careers. I never thought I would go to college. My mother was a great person — she really wanted my siblings and me to be happy — but there were six kids and my father wasn’t around, so she was awfully busy. No one really talked to me about college.
After graduating early from high school, I got a job as a bookkeeper at the Ford car dealership, where I worked for several months. But I realized that day after day after day of that would be a long life. For the first time, I started thinking about what I might want to do, and got financial aid to enroll as a business major in the University of Wisconsin-Superior. There were times throughout my freshman and sophomore year when I quit, because I didn’t really get a sense of where it was all going. But I always went back the same semester.
Then I took a class called Human Genetics for Non-Majors, and just fell in love. I really buckled down after that.
What motivated you to focus your work on embryonic development and fertility?
I was interested in those questions on a personal level because my sister and I both had ovarian cancer early. So I never had kids. But I was also drawn to the miraculous nature of it all. I want to understand cell fate decisions, to answer questions like: What is it about an egg and a sperm that allows them to pass information from one generation to the next? How are the patterns set up so that after fertilization, it always takes 11 hours until the next cell division? It’s incredible just how little we understand about what an egg and sperm really are.
I’m still amazed that a sperm and an egg come together and you get a human. It’s crazy, isn’t it?
Just how little do we know about that?
We’re still very much in a descriptive phase. Even getting video images of fertilization and embryonic development is recent history. The same goes for molecular events, but we’ve been adding categories of description — like the study of inherited epigenetic markers. Now, with single-cell analysis, we can really start to probe those categories. We’re just beginning to understand which gene programs are on and off, and what those programs are doing. It’s turned out, for instance, that some of the fundamental epigenetic programs [like histone modification and the activation of transcription] in human and mice embryos are surprisingly different from each other, which makes me think that epigenetic factors are determining species-specific processes.
What has your work told you about the uniqueness of human reproduction, then?
That the timing is unique. Our research shows that the timing in embryonic development is very precise and human-specific, which makes perfect sense, since it has to match the timing in uterine development. We’ve broken that down in terms of how much time passes until the first cell division, the second, the third … and what that means for the health of the embryo. One result that’s emerged is a relationship between these timing parameters and chromosome number — too fast or too slow correlates perceptibly with embryos having the wrong number of chromosomes.
What are these timing parameters, exactly, and why are they important?
We combined imaging and gene expression measurements to look at how the embryonic clock ticks. Just after the egg and sperm fuse, there’s very little transcription [because the embryo uses a stockpile of maternal RNA from the egg to make essential proteins]. Then on the third day, the embryonic genome gets turned on, at which point there’s a major wave of transcription. We really want to know what programs drive those first three days of development. According to our work, it’s precisely timed cell divisions, coordinated with epigenetic factors and this switch from maternal to embryonic gene expression.
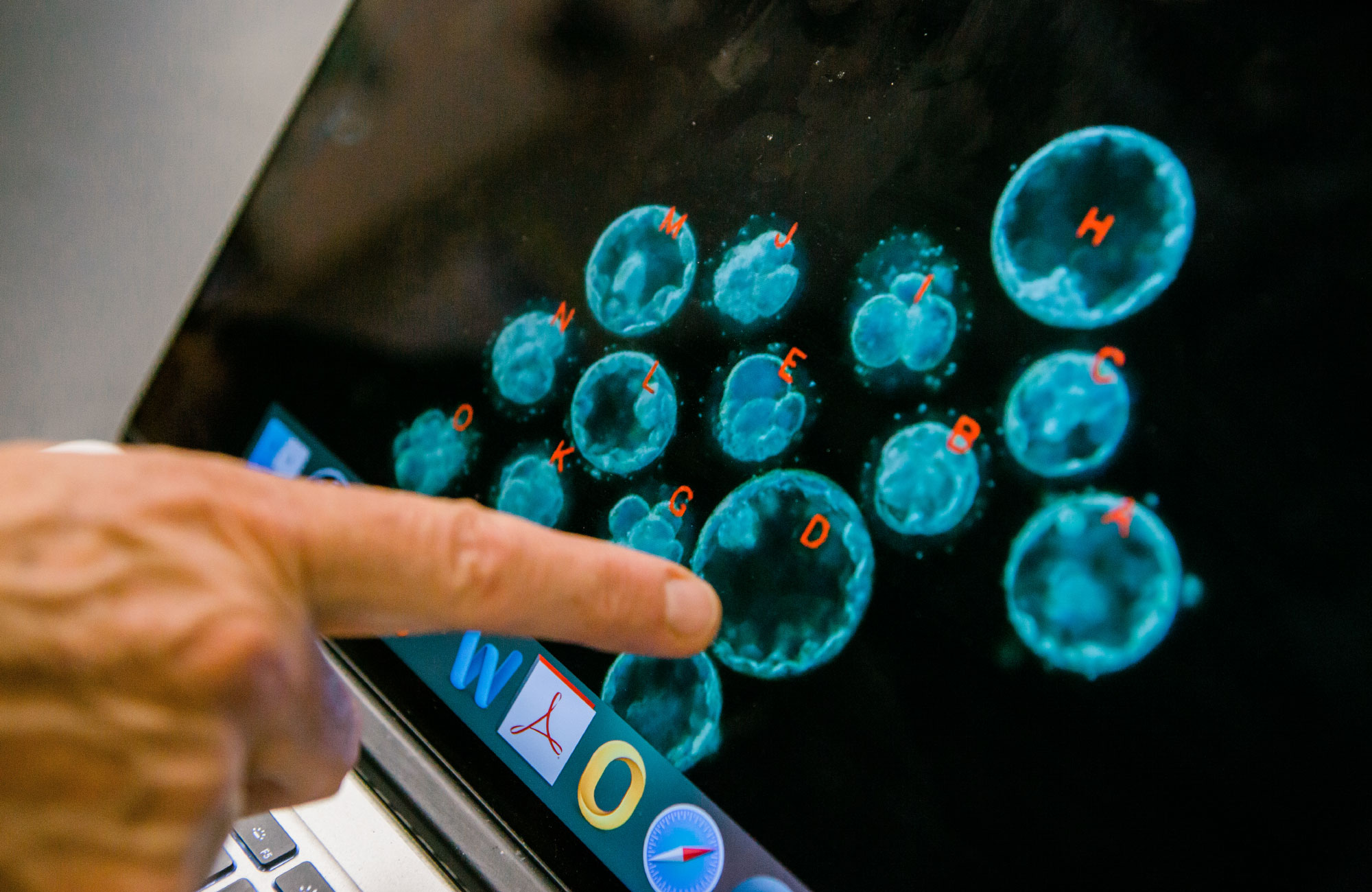
Pera points to a time-lapse video of early cell divisions in a series of human embryos. Her work has helped to show that the fate of these early cells depends in part on their expression of retroviral genes integrated into our DNA.
Kristine Paulsen for Quanta Magazine
The timing parameters relate to the first three cell divisions, which are important for the survival of the embryo before the embryonic genes even start getting expressed.
First, it takes about 30 minutes, plus or minus 10, for the first cell in an embryo to form a waist, or cleavage furrow. We found that this can to some extent be a good metric of chromosome number. When the timing is off, there’s a greater chance the embryo will have three sets of chromosomes instead of two. It may start to fragment, forming cleavage furrows around the other chromosomes, or fail to divide at all.
The next cell division, from two cells to three cells, should take 11 hours, plus or minus four. The timing of the second division really reflects how mature the egg is.
Finally, the fourth cell should appear pretty soon after the third. That reflects how evenly divided the nutrients of the first two cells are: If one of them gets all the nutrients, it will divide faster while the other one lags behind.
But developmental timing is important not just for the immediate survival of the embryo, but for health and disease further down the line, right?
Yes, that’s one of our big hypotheses, because of the role timing plays in deciding the fates of cells. The idea is that development starts with a certain timeline. If a cell does not make it to a certain stage on time, its cell fate can change, and it’s typically thrown out of the tissue. It’s expelled and it dies.
In a paper in Nature Genetics in 2016, for instance, we identified nearly 150 new genes, many of which were retroviral, that get expressed very early on in human stem cells. Then, when we turned some of the retroviral genes off, we found it changed the fate of the cells. Instead of becoming embryonic stem cells — which ultimately give rise to the fetus — the cells could only contribute to the trophectoderm layer, which gives rise to the tissues that attach the embryo to the uterus. The trophectoderm is made up in part of “bad” cells that won’t make it as part of the fetus.
Which means that part of the puzzle on timing and which cells become part of the embryo and which become part of the extra-embryonic tissue depends on human-specific sequences that we inherited from retroviral integration.
That seems surprising — that viral DNA would play such an important role so early in development.
Over the course of evolution, viruses have been in us and on us and changing us continually. We wrote another paper in Nature with Joanna Wysocka at Stanford, suggesting that these viral sequences have another, second effect: that they not only contribute to cell fate but also help the embryo get rid of viral infections.
In both cases, then, it seems like we have a cleanup system early in development that’s viral-based.
You also study factors that determine whether or not cells will become germ cells. What’s at play there?
In a paper published in Nature Cell Biology in April, we identified a triad of proteins that interact to help form primordial germ cells and maintain the primordial germ cell fate.
We knew that the protein OCT4 is one of the master regulators of embryonic development and cell fate. But how do you tell if an embryonic cell will form a primordial germ cell, given that both express OCT4? We thought OCT4 could be acting with different partners in specific ways to enable the birth of the germ line.
That’s what a decision looks like in cells. You can think of it as a fulcrum: The stem cell sits in the middle, and if OCT4 and the other proteins are acting in concert, that fulcrum will tilt toward the germ line. If one of the proteins isn’t present in the right amount, we found that the cells instead become ectoderm, embryonic tissue that typically gives rise to neurons. It’s a delicate balance. We’re now starting to unpack each protein’s actual function in embryonic and germ cell development, and we’re studying how other transcription factors might be involved.
So going back to your question about how development and disease are related: There has to be an error rate in these cell fate decisions — where you may get fewer primordial germ cells or more neurons depending on how this axis worked in, say, the 10th week of fetal life — but we don’t really know what that error rate is or what its consequences are.
Can you give an example?
Generally, if cell type A has to form before cell type B can, but too few of A have been made, then that has a ripple effect on the formation and number of B. A basal sheet of cells has to be present before neurons can develop, for example. In a disorder like Parkinson’s disease, patients have approximately 75,000 fewer dopamine-producing neurons. You can imagine this loss happening for one of two reasons: They start out with fewer neurons — maybe with only 50,000 — or they lose that particular class of neurons at a greater rate.
We know that exposure to some pesticides and chemicals can cause the latter, but still don’t know whether Parkinson’s is related to a natural inheritance of fewer dopamine-secreting neurons. This is a hypothesis I’ve been working on for a while: that our genetic makeup is linked to disease not just through environmental cues, but also because we are endowed with a certain cell number at birth, and early development can affect what happens many, many years later.
Something similar has been shown in menopause, for instance. In some conditions, women are born with fewer oocytes and experience premature ovarian failure. There are other examples in neurology, too, particularly in mice. But what part that plays in human disease hasn’t really been explored much, which is why we’re now looking for whether this is happening in Parkinson’s.
You gave up a directorship position at Stanford University to work at Montana State in 2013, prompted in part by a wish to have more of an impact in science education. Why?
I think most Americans aren’t all that interested in science or scientific research. My family, for example, doesn’t have much science background. Among students at Stanford, though, that wasn’t really the case. The problem that we as scientists have in the U.S. is not in convincing those students that science is important. It’s about convincing students, and the parents of students, at large public universities like Montana State, who haven’t necessarily been exposed to the importance of science.
We’re missing a kind of message that used to be much more common.
I remember as a young girl, sitting with my mother, who was a cook at the elementary school, and waiting to see people land on the moon. We’d spent all week making food, preparing ourselves to sit down and watch the landing. This was a big deal.
Things that are every bit as great happen every day in scientific labs, and no one cares. Maybe as a society we’ve become anesthetized to science — when really, it’s so exciting. We have so much to gain as a country if we invest in science and knowledge and understanding. I don’t blame the public for not understanding, though, or even legislators for sometimes not wanting to invest. They all look so much like my family.
What’s missing from science education, and how do we address that?
I think that the scientists themselves are an important part of the story, that who they are can help portray the science in a valid way that remains exciting. Will the public find the regulatory network I study as exciting as I do? With the right background to understand it, maybe. But I think what’s exciting to someone without that knowledge is how my story connects with my work: I grew up the youngest of six kids, my mother didn’t even graduate from high school, and I ended up in an endowed chair at Stanford, leading what I think is a great life, full of exploration. I wasn’t special, I was just trying to make a living, but still I’m in science.
We haven’t made the story show that science, no matter how fundamental, is about you, about your family, about me, about our journey. I grew up in a place where you sit around and tell stories all winter, then disperse in the summer — only to come back the following winter and tell the same stories again, and maybe add a few more. We’re not telling these [science] stories over and over again. We’re not acting proud of them, like we were with the landing on the moon. We have to make it about those stories again.