Intelligence Evolved at Least Twice in Vertebrate Animals
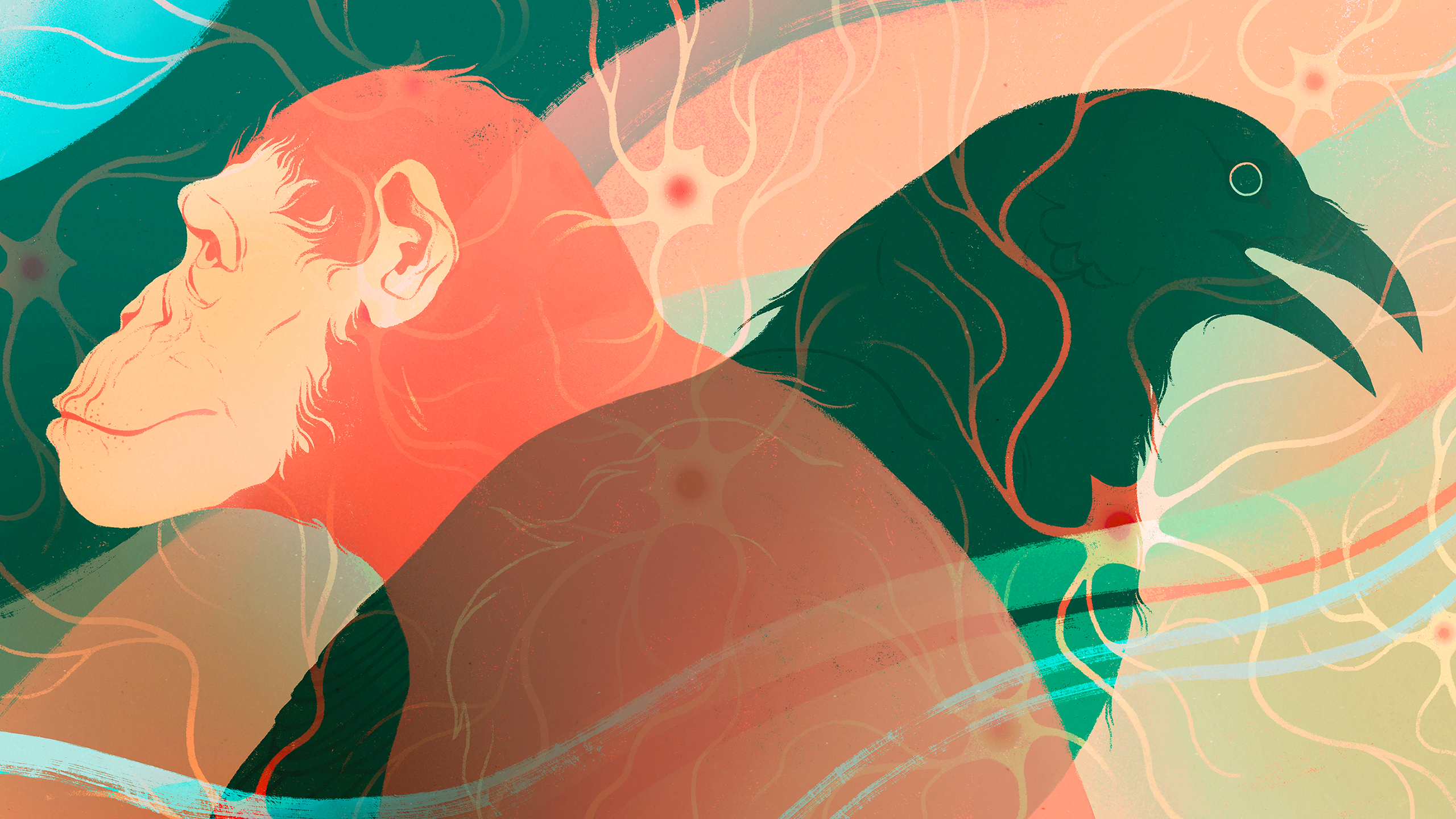
Did the neural circuits that support intelligence evolve once in vertebrates, or independently in birds and mammals?
Samantha Mash for Quanta Magazine
Introduction
Humans tend to put our own intelligence on a pedestal. Our brains can do math, employ logic, explore abstractions and think critically. But we can’t claim a monopoly on thought. Among a variety of nonhuman species known to display intelligent behavior, birds have been shown time and again to have advanced cognitive abilities. Ravens plan for the future, crows count and use tools, cockatoos open and pillage booby-trapped garbage cans, and chickadees keep track of tens of thousands of seeds cached across a landscape. Notably, birds achieve such feats with brains that look completely different from ours: They’re smaller and lack the highly organized structures that scientists associate with mammalian intelligence.
“A bird with a 10-gram brain is doing pretty much the same as a chimp with a 400-gram brain,” said Onur Güntürkün, who studies brain structures at Ruhr University Bochum in Germany. “How is it possible?”
Researchers have long debated about the relationship between avian and mammalian intelligences. One possibility is that intelligence in vertebrates — animals with backbones, including mammals and birds — evolved once. In that case, both groups would have inherited the complex neural pathways that support cognition from a common ancestor: a lizardlike creature that lived 320 million years ago, when Earth’s continents were squished into one landmass. The other possibility is that the kinds of neural circuits that support vertebrate intelligence evolved independently in birds and mammals.
It’s hard to track down which path evolution took, given that any trace of the ancient ancestor’s actual brain vanished in a geological blink. So biologists have taken other approaches — such as comparing brain structures in adult and developing animals today — to piece together how this kind of neurobiological complexity might have emerged.
A series of studies published in Science in February 2025 provides the best evidence yet that birds and mammals did not inherit the neural pathways that generate intelligence from a common ancestor, but rather evolved them independently. This suggests that vertebrate intelligence arose not once, but multiple times. Still, their neural complexity didn’t evolve in wildly different directions: Avian and mammalian brains display surprisingly similar circuits, the studies found.
“It’s a milestone in the quest to understand and to integrate the different ideas about the evolution” of vertebrate intelligence, said Güntürkün, who was not involved in the new research.
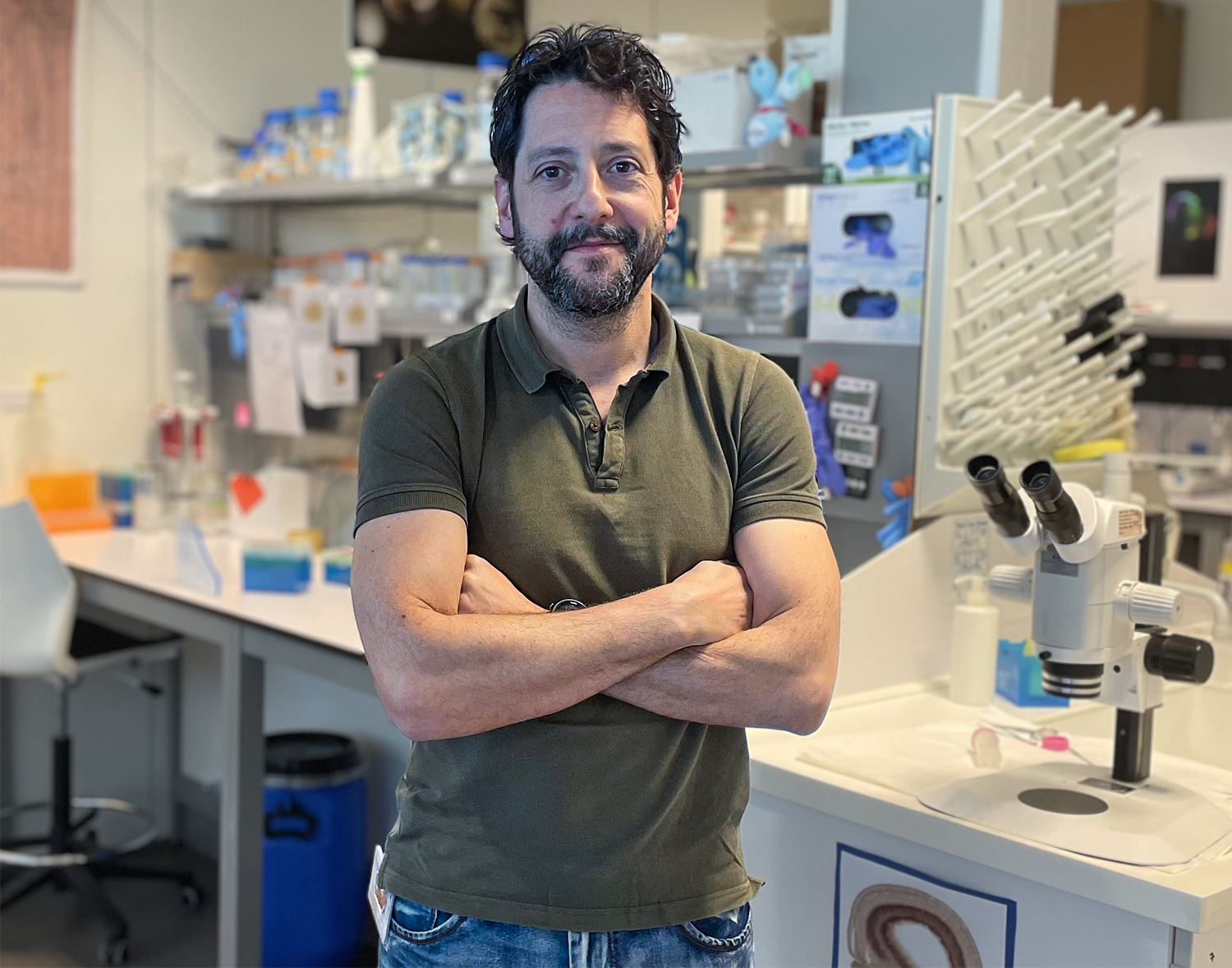
When Fernando García-Moreno started his lab at the Achucarro Basque Center for Neuroscience, he knew he wanted to probe how the pallium region of the vertebrate brain evolved using a breadth of different methods.
Tatiana Gallego Flores
The findings emerge in a world enraptured by artificial forms of intelligence, and they could teach us something about how complex circuits in our own brains evolved. Perhaps most importantly, they could help us step “away from the idea that we are the best creatures in the world,” said Niklas Kempynck, a graduate student at KU Leuven who led one of the studies. “We are not this optimal solution to intelligence.”
Birds got there too, on their own.
Pecking Disorder
For the first half of the 20th century, neuroanatomists assumed that birds were simply not that smart. The creatures lack anything resembling a neocortex — the highly ordered outermost structure in the brains of humans and other mammals where language, communication and reasoning reside. The neocortex is organized into six layers of neurons, which receive sensory information from other parts of the brain, process it and send it out to regions that determine our behavior and reactions.
“For the longest time, it was thought that this is the center of cognition, and you need this kind of anatomy to develop advanced cognitive abilities,” said Bastienne Zaremba, a postdoctoral researcher studying the evolution of the brain at Heidelberg University.
Rather than neat layers, birds have “unspecified balls of neurons without landmarks or distinctions,” said Fernando García-Moreno, a neurobiologist at the Achucarro Basque Center for Neuroscience in Spain. These structures compelled neuroanatomists a century ago to suggest that much of bird behavior is reflexive, and not driven by learning and decision-making. This “implies that what a mammal can learn easily, a bird will never learn,” Güntürkün said.
The conventional thinking started to change in the 1960s when Harvey Karten, a young neuroanatomist at the Massachusetts Institute of Technology, mapped and compared brain circuits in mammals and pigeons, and later in owls, chickens and other birds. What he found was a surprise: The brain regions thought to be involved only in reflexive movements were built from neural circuits — networks of interconnected neurons — that resembled those found in the mammalian neocortex. This region in the bird brain, the dorsal ventricular ridge (DVR), seemed to be comparable to a neocortex; it just didn’t look like it.
In 1969, Karten wrote a “very influential paper that completely changed the discussion in the field,” said Maria Tosches, who studies vertebrate brain development at Columbia University. “His work was really revolutionary.” He concluded that because avian and mammalian circuits are similar, they were inherited from a common ancestor. That thinking dominated the field for decades, said Güntürkün, a former postdoc in Karten’s lab. It “sparked quite a lot of interest in the bird brain.”
A few decades later, Luis Puelles, an anatomist at the University of Murcia in Spain, drew the opposite conclusion to Karten. By comparing embryos at various stages of development, he found that the mammalian neocortex and the avian DVR developed from distinct areas of the embryo’s pallium — a brain region shared by all vertebrates. He concluded that the structures must have evolved independently
Karten and Puelles were “giving completely different answers to this big question,” Tosches said. The debate continued for decades. During this time, biologists also began to appreciate bird intelligence, starting with their studies of Alex, an African gray parrot who could count and identify objects. They realized just how smart birds could be.
However, neither group seemed to want to resolve the discrepancy between their two theories of how vertebrate palliums evolved, according to García-Moreno. “No, they kept working on their own method,” he said. One camp continued to compare the circuitry in adult vertebrate brains; the other focused on embryonic development.
In the new studies, he said, “we tried to put everything together.”
Same but Not the Same
Two new studies, which were conducted by independent teams of researchers, relied on the same powerful tool for identifying cell types, known as single-cell RNA sequencing. This technique lets researchers compare neuronal circuits, as Karten did, not only in adult brains but all the way through embryonic development, following Puelles. In this way, they could see where the cells started growing in the embryo and where they ended up in the mature animal — a developmental journey that can reveal evolutionary pathways.
For their study, García-Moreno and his team wanted to watch how brain circuitry develops. Using RNA sequencing and other techniques, they tracked cells in the palliums of chickens, mice and geckos at various embryonic stages to time-stamp when different types of neurons were generated and where they matured.
They found that the mature circuits looked remarkably alike across animals, just as Karten and others had noted, but they were built differently, as Puelles had found. The circuits that composed the mammalian neocortex and the avian DVR developed at different times, in different orders and in different regions of the brain.
Mark Belan/Quanta Magazine;
source: Science 387, 732 (2025)
At the same time, García-Moreno was collaborating with Zaremba and her colleagues at Heidelberg University. Using RNA sequencing, they created “the most comprehensive atlas of the bird pallium that we have,” said Tosches, who wrote a related perspective piece published in Science. By comparing the bird pallium to lizard and mouse palliums, they also found that the neocortex and DVR were built with similar circuitry — however, the neurons that composed those neural circuits were distinct.
“How we end up with similar circuitry was more flexible than I would have expected,” Zaremba said. “You can build the same circuits from different cell types.”
Zaremba and her team also found that in the bird pallium, neurons that start development in different regions can mature into the same type of neuron in the adult. This pushed against previous views, which held that distinct regions of the embryo must generate different types of neurons.
In mammals, brain development follows an intuitive path: The cells in the embryo’s amygdala region at the start of development end up in the adult amygdala. The cells in the embryo’s cortex region end up in the adult cortex. But in birds, “there is a fantastic reorganization of the forebrain,” Güntürkün said, that is “nothing that we had expected.”
Taken together, the studies provide the clearest evidence yet that birds and mammals independently evolved brain regions for complex cognition. They also echo previous research from Tosches’ lab, which found that the mammalian neocortex evolved independently from the reptile DVR.
Still, it seems likely there was some inheritance from a common ancestor. In a third study that used deep learning, Kempynck and his co-author Nikolai Hecker found that mice, chickens and humans share some stretches of DNA that influence the development of the neocortex or DVR, suggesting that similar genetic tools are at work in both types of animals. And as previous studies had suggested, the research groups found that inhibitory neurons, or those that silence and modulate neural signals, were conserved across birds and mammals.
The findings haven’t completely resolved Karten and Puelles’ debate, however. Whose ideas were closer to the truth? Tosches said that Puelles was right, while Güntürkün thought the findings better reflect Karten’s ideas, though would partly please Puelles. García-Moreno split the difference: “Both of them were right; none of them was wrong,” he said.
How To Build Intelligence
Intelligence doesn’t come with an instruction manual. It is hard to define, there are no ideal steps toward it, and it doesn’t have an optimal design, Tosches said. Innovations can happen throughout an animal’s biology, whether in new genes and their regulation, or in new neuron types, circuits and brain regions. But similar innovations can evolve multiple times independently — a phenomenon known as convergent evolution — and this is seen across life.
“One of the reasons I kind of like these papers is that they really highlight a lot of differences,” said Bradley Colquitt, a molecular neuroscientist at the University of California, Santa Cruz. “It allows you to say: What are the different neural solutions that these organisms have come up with to solve similar problems of living in a complex world and being able to adapt in a rapidly changing terrestrial environment?”
Octopuses and squids, independently of mammals, evolved camera-like eyes. Birds, bats and insects all took to the skies on their own. Ancient people in Egypt and South America independently built pyramids — the most structurally efficient shape that will stand the test of time, García-Moreno said: “If they make a tower, it will fall. If they make a wall, it won’t work.”
Similarly, “there’s limited degrees of freedom into which you can generate an intelligent brain, at least within vertebrates,” Tosches said. Drift outside the realm of vertebrates, however, and you can generate an intelligent brain in much weirder ways — from our perspective, anyway. “It’s a wild west,” she said. Octopuses, for example, “evolved intelligence in a way that’s completely independent.” Their cognitive structures look nothing like ours, except that they’re built from the same broad type of cell: the neuron. Yet octopuses have been caught performing incredible feats such as escaping aquarium tanks, solving puzzles, unscrewing jar lids and carrying shells as shields.
It would be exciting to figure out how octopuses evolved intelligence using really divergent neural structures, Colquitt said. That way, it might be possible to pinpoint any absolute constraints on evolving intelligence across all animal species, not just vertebrates.
Such findings could eventually reveal shared features of various intelligences, Zaremba said. What are the building blocks of a brain that can think critically, use tools or form abstract ideas? That understanding could help in the search for extraterrestrial intelligence — and help improve our artificial intelligence. For example, the way we currently think about using insights from evolution to improve AI is very anthropocentric. “I would be really curious to see if we can build like artificial intelligence from a bird perspective,” Kempynck said. “How does a bird think? Can we mimic that?”