Magnetism May Have Given Life Its Molecular Asymmetry
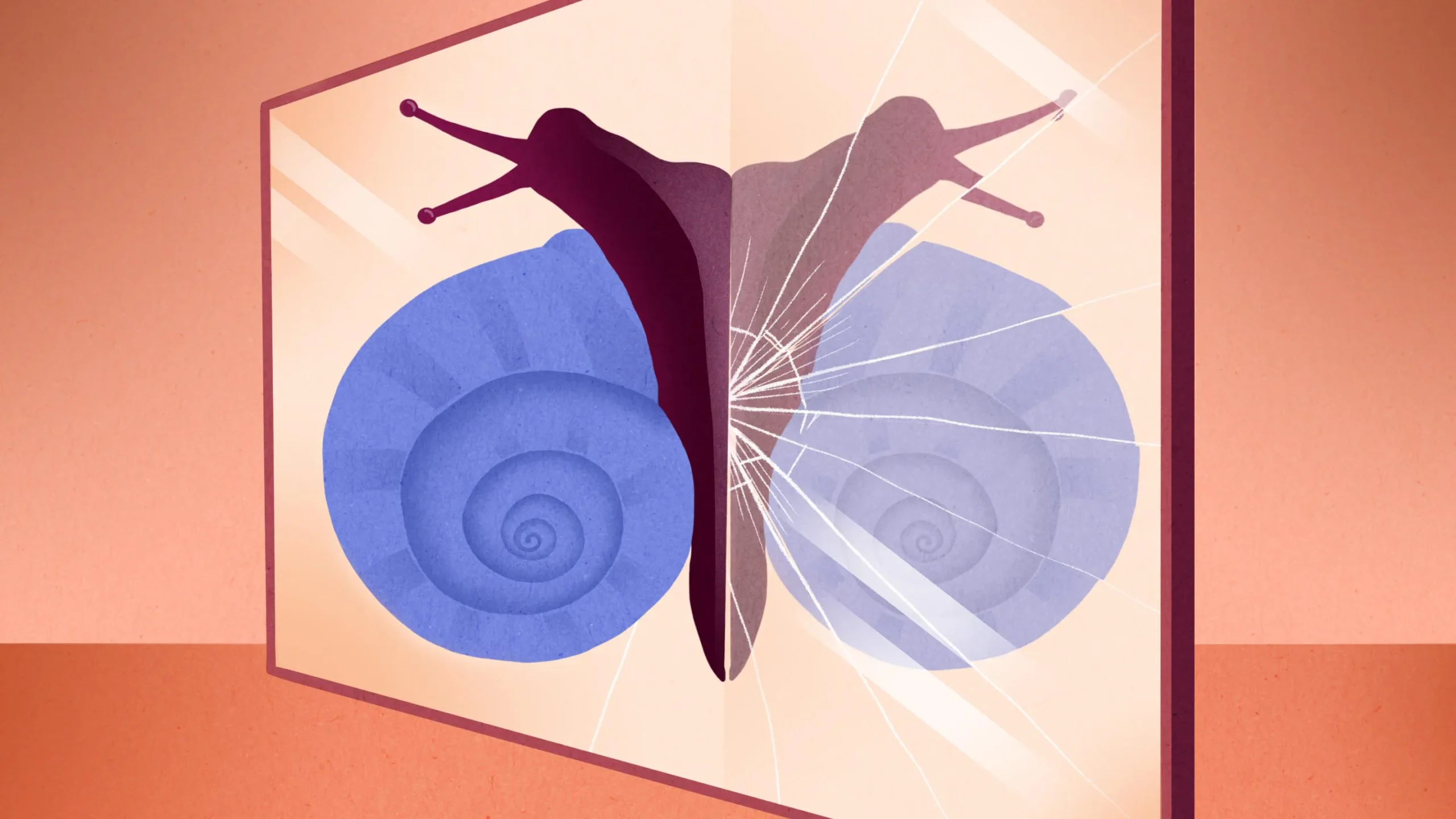
Living things are asymmetrical, even at the molecular level: Although many essential biomolecules exist in distinct mirror-image forms, cells tend to use just one of those forms exclusively.
Lou Kiss for Quanta Magazine
Introduction
In 1848, when Louis Pasteur was a young chemist still years away from discovering how to sterilize milk, he discovered something peculiar about crystals that accidentally formed when an industrial chemist boiled wine for too long. Half of the crystals were recognizably tartaric acid, an industrially useful salt that grew naturally on the walls of wine barrels. The other crystals had exactly the same shape and symmetry, but one face was oriented in the opposite direction.
The difference was so stark that Pasteur could separate the crystals under a magnifying lens with tweezers. “They are in relation to each other what an image is, in a mirror, in relation to the real thing,” he wrote in a paper that year.
Though Pasteur didn’t know it, in the crystallized dregs of that wine, he had stumbled across one of the deepest mysteries about the origins of life on Earth.
What he was seeing was a mixture of tartaric acid molecules that had identical atomic compositions and mirror-image arrangements of those atoms in space. They had the property later called “chirality” after the Greek word for “hand”: Just as our left and right hands are symmetrical opposites of each other, the left- and right-handed versions (or enantiomers) of the tartaric acid molecules are distinct and nonequivalent.
The significance of Pasteur’s observation went beyond the discovery of chirality — there was also the remarkable reason he was seeing it. The synthetic crystals were a mixture of the tartaric acid enantiomers because the boiling process allowed left- and right-handed versions to form in equal numbers. But in the natural crystals from wine barrels, all the tartaric acid molecules were right-handed — because the grapes used for the wine, picked from living vines, only made that enantiomer.
Chirality is a signature of life as we know it. Over and over, biochemists have found that when living cells use chiral molecules, they use one chirality exclusively. The sugars that make up DNA, for example, are all right-handed. The amino acids that make up proteins are all left-handed. If the wrong enantiomers slip into pharmaceuticals, the effects can sometimes be toxic or even lethal.
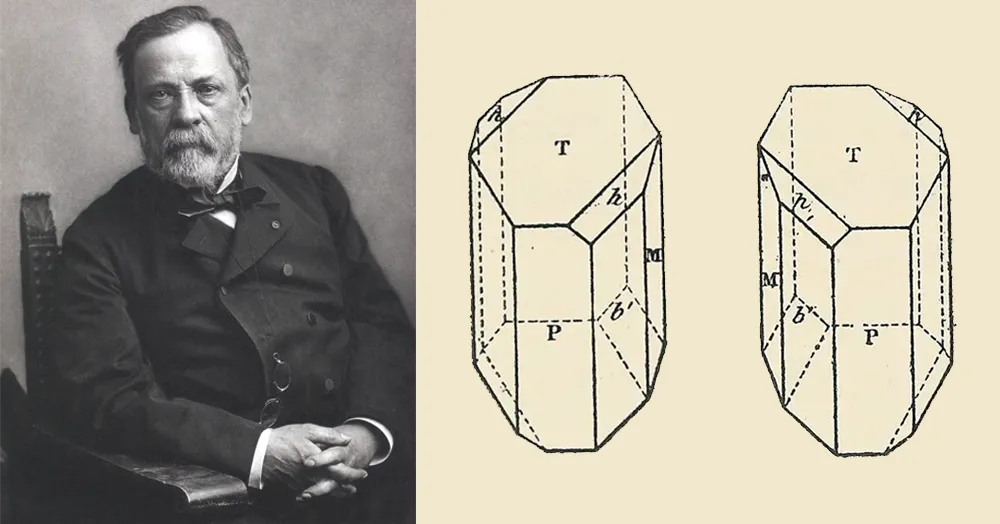
The French chemist Louis Pasteur (left) drew this illustration in 1848 to depict how the crystals of the mixture now called paratartaric acid are symmetrical but not superimposable. That property later became known as chirality.
Paul Nadar (left); Annales de Chimie et de Physique
Some event or series of events early in the history of life must have “broken the mirror,” as biochemists put it, throwing life into molecular asymmetry. Scientists have debated why life became homochiral, and whether it needed to happen or if it was purely a fluke. Were chiral preferences impressed on early life by biased samples of molecules arriving from space, or did they somehow evolve out of mixtures that started out as equal parts right- and left-handed?
“Scientists have been mystified by this observation,” said Soumitra Athavale, an assistant professor of organic chemistry at the University of California, Los Angeles. “They’ve come up with all sorts of proposals over the years, but it’s difficult to come up with proposals which are actually relevant geologically.” Moreover, while many theories could explain why one type of molecule might have become homochiral, none of them explained why whole networks of biomolecules did.
Recently, a group at Harvard University published a series of papers that present an intriguing solution for how life’s homochirality emerged. They suggest that magnetic surfaces on minerals in bodies of water on the primordial Earth, charged by the planet’s magnetic field, could have served as “chiral agents” that attracted some forms of molecules more than others, kicking off a process that amplified the chirality of biological molecules, from RNA precursors all the way to proteins and beyond. Their proposed mechanism would explain how a bias in the makeup of certain molecules could have cascaded outward to create a vast network of chiral chemistry supporting life.
It’s not the only plausible hypothesis, but “it’s one of the coolest because it ties geophysics to geochemistry, to prebiotic chemistry, [and] ultimately to biochemistry,” said Gerald Joyce, a biochemist and president of the Salk Institute who was not involved in the study. He is also impressed that the hypothesis is backed by “actual experiments” and that “they’re doing this under realistic conditions.”
The CISS Effect
The roots of the new theory about homochirality reach back almost a quarter century to when Ron Naaman, a professor of chemical physics at the Weizmann Institute of Science in Israel, and his team discovered a critical effect of chiral molecules. Their work focused on the fact that electrons have two key properties: They carry a negative charge, and they have “spin,” a quantum property analogous to intrinsic clockwise or counterclockwise rotation. When molecules interact with other molecules or surfaces, their electrons can redistribute themselves, polarizing the molecules by creating a negative charge at their destination and a positive charge at their starting point.
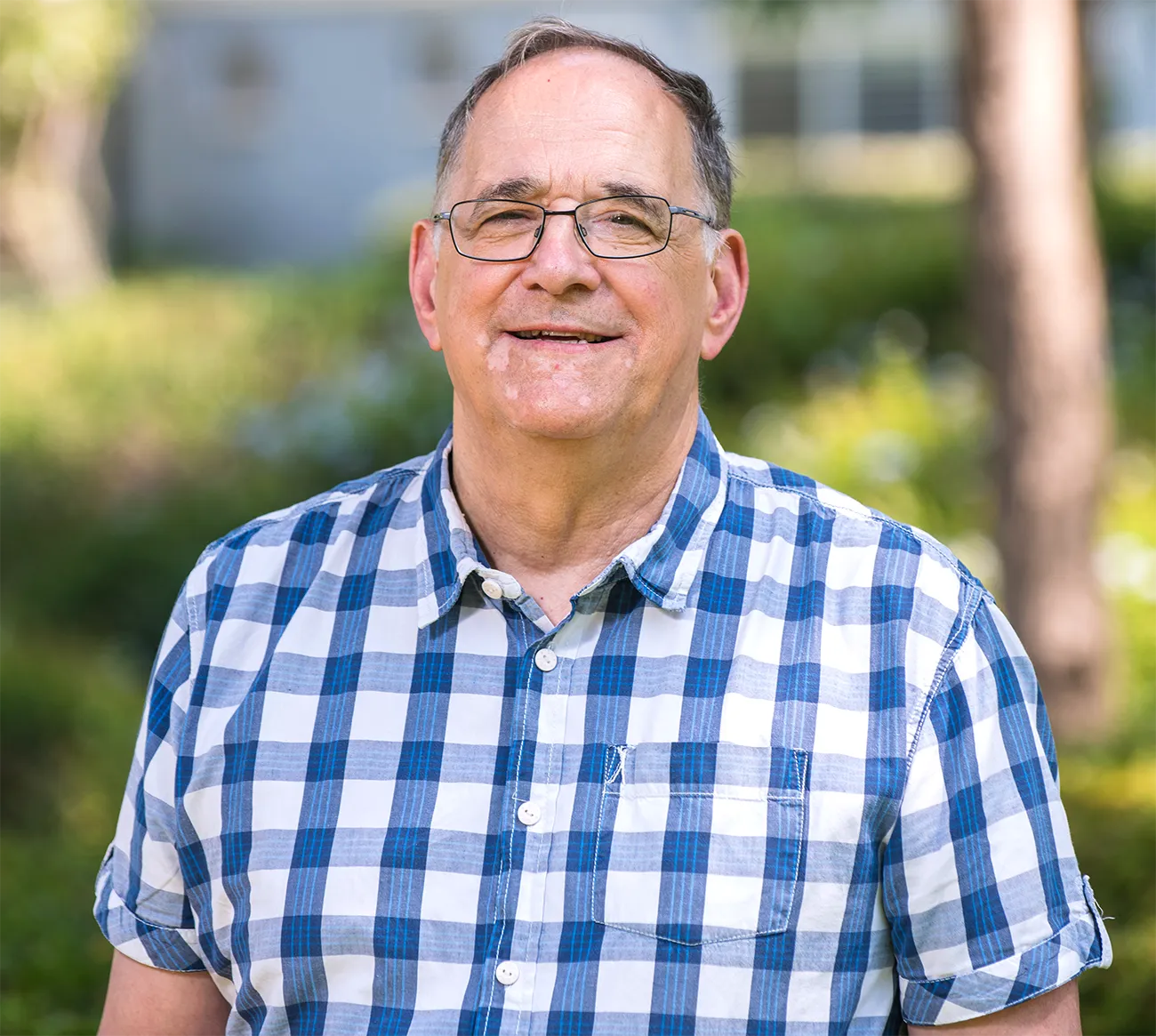
Ron Naaman of the Weizmann Institute of Science in Israel discovered the CISS effect in 1999. Its applications and biological significance have continued to emerge since then.
Courtesy of Ron Naaman
Naaman and his team discovered that chiral molecules filter electrons based on the direction of their spin. Electrons with one spin orientation will move more efficiently across a chiral molecule in one direction than the other. Electrons with the opposite spin move more freely the other way.
To understand why, imagine throwing a Frisbee that glances off the wall of a hallway. If the Frisbee hits the right-hand wall, it will bounce forward only if it’s rotating clockwise; otherwise, it will bounce backward. The opposite will happen if you hit the Frisbee off the left-hand wall. Similarly, chiral molecules “scatter the electrons according to their direction of rotation,” Naaman said. He and his team named this phenomenon the chiral-induced spin selectivity (CISS) effect.
Because of that scattering, electrons with a given spin end up aggregating at one pole of a chiral molecule (and the right-handed and left-handed versions of the molecule gather opposite spins at their respective poles). But that redistribution of spins affects how the chiral molecules interact with magnetic surfaces because electrons spinning in opposite directions attract one another, and those spinning in the same direction repel one another.
Consequently, when a chiral molecule approaches a magnetic surface, it will be drawn closer if the molecule and the surface have opposite spin biases. If their spins match, they will repel each other. (Because other chemical interactions are also going on, the molecule can’t simply flip to realign itself.) So a magnetic surface can act as a chiral agent, preferentially interacting with only one enantiomer of a compound.
In 2011, in collaboration with a team at the University of Münster in Germany, Naaman and his team measured the spin of electrons as they moved through double-stranded DNA, confirming that the CISS effect is both real and strong.
That’s when research into the effect and its possible applications “started to boom,” Naaman said. He and his team, for example, developed several ways to use the CISS effect to remove impurities from biomedicines, or to exclude the wrong enantiomers from drugs to prevent major side effects. They’ve also explored how the CISS effect might help to explain the mechanisms of anesthesia.
But they only began working seriously on the idea that the CISS effect plays a part in the rise of biological homochirality after they were invited to collaborate on a hypothesis by a team at Harvard led by the astronomer Dimitar Sasselov and his graduate student S. Furkan Ozturk.
A Physics Perspective
Ozturk, the young lead author on the recent papers, came across the homochirality problem in 2020 when he was a physics graduate student at Harvard. Unhappy with his research on quantum simulations using ultracold atoms, he flipped through a science magazine detailing 125 of the biggest mysteries in the world and learned about homochirality.
“It looked really like a physics question because it’s about symmetries,” he said. After reaching out to Sasselov, who is the director of Harvard’s Origins of Life Initiative and who was already interested in the question of homochirality, Ozturk switched over to become a student in his lab.
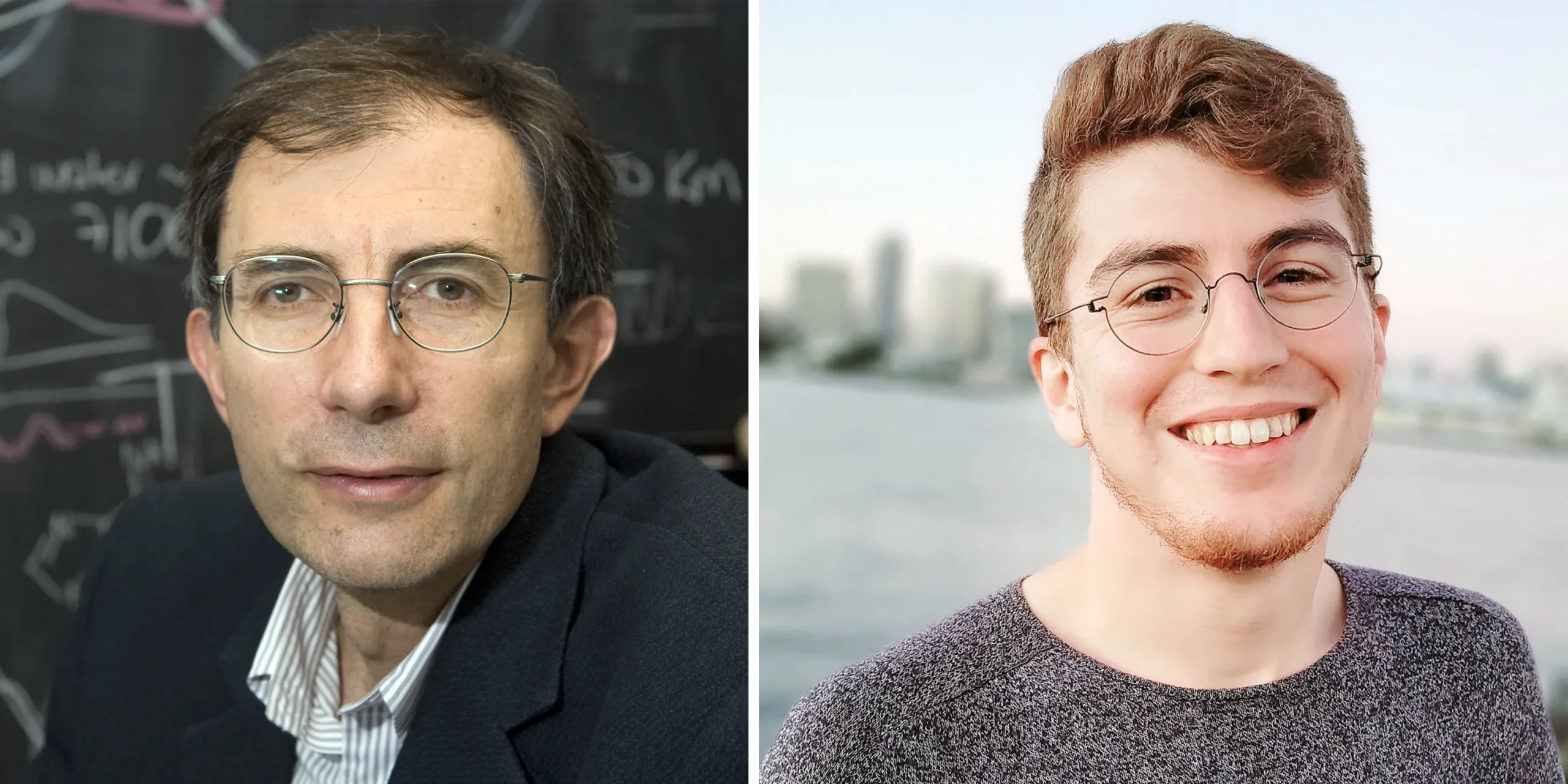
Dimitar Sasselov (left) and Furkan Ozturk at Harvard University led experiments that suggested magnetic surfaces in lakes could have imposed homochirality on vital biomolecules at the beginning of life’s history.
Jon Chase/Harvard (left); Courtesy of S. Furkan Ozturk
Ozturk and Sasselov soon hit on an idea based on the CISS effect. They imagined a primordial setting like a shallow lake where there were surfaces full of magnetic minerals and the water contained a mixture of chiral precursors to nucleotides. They theorized that ultraviolet light could have ejected many electrons from the magnetic surfaces, and many of those electrons would have had the same spin. The ejected electrons might then have interacted preferentially with specific enantiomers, and the resulting chemical reactions might then have preferentially assembled right-handed RNA precursors.
In April 2022, Ozturk traveled to Naaman’s lab in Israel, thrilled by the prospect of testing their hypothesis. His excitement was short-lived. Over the next month as he worked with Naaman, the idea fell apart. It “did not work,” Ozturk said, and so he returned home, dejected.
But then Ozturk had another idea. What if the CISS effect wasn’t manifesting as a chemical process but as a physical one?
Naaman’s group had shown that they could use magnetic surfaces to crystallize enantiomers preferentially. And crystallization would be the easiest way for purified collections of enantiomers to assemble. Ozturk mentioned that to John Sutherland, their collaborator at the MRC Laboratory of Molecular Biology in the U.K. “And I said, drop everything to do with electrons and just focus on the crystallization,” Sutherland said.
Sutherland was excited by the crystallization aspect because he and his team had already independently discovered that an RNA precursor called ribo-aminooxazoline (RAO) can synthesize two of the four building blocks of RNA. RAO also “crystallizes beautifully,” Sutherland said. Once a crystal seed forms from the enantiomer that is attracted to the surface, the crystal preferentially grows by incorporating more of the same enantiomer.
Ozturk remembers Sutherland telling him that it would be “game over” if the CISS effect idea worked. “Because it was so simple,” Ozturk said. “It was doing it on a molecule that was so central to the origin of life chemistry that if you can manage to make that molecule homochiral, you can make the entire system homochiral.”
Ozturk got to work in the Harvard lab. He put magnetite surfaces onto a petri dish and filled it with a solution containing equal amounts of left-handed and right-handed RAO molecules. He then put the dish on a magnet, put the experiment in the fridge and waited for the first crystals to appear. At first, the team found that 60% of the crystals were single-handed. When they repeated the process, their crystals were 100% of the same chirality.
Merrill Sherman/Quanta Magazine
As they reported in a study published in June in Science Advances, if they magnetized the surface one way, they created crystals that were purely right-handed; if they magnetized it the other way, the crystals were purely left-handed. “I was very surprised, because I’m super familiar with experiments that do not work,” Ozturk said. But this one “worked like a charm.”
Behind his desk, Ozturk keeps the empty bottle of champagne that Sasselov and the team shared at a celebratory dinner.
Multiply and Amplify
But they still had a major problem: The magnet they used in their experiment was about 6,500 times stronger than Earth’s magnetic field.
So Ozturk returned to the Weizmann Institute last November, and he and Naaman then worked on a follow-up experiment in which they didn’t use an external magnetic field at all. Instead, they found that when the chiral molecules were adsorbed onto the magnetic surfaces, they created a highly local magnetic field over the surface that was up to 50 times as strong as Earth’s magnetic field. Their findings have been accepted by a peer-reviewed journal but not yet published.
“You’re coercing the neighborhood to be magnetized, which makes it even easier for the crystals to keep forming,” Joyce said. That self-perpetuating effect makes the scenario plausible, he added.
Athavale agrees. The fact that you don’t need a highly magnetic field for the CISS effect to occur is “really nice, because now you have seen a possible geological setting,” he said.
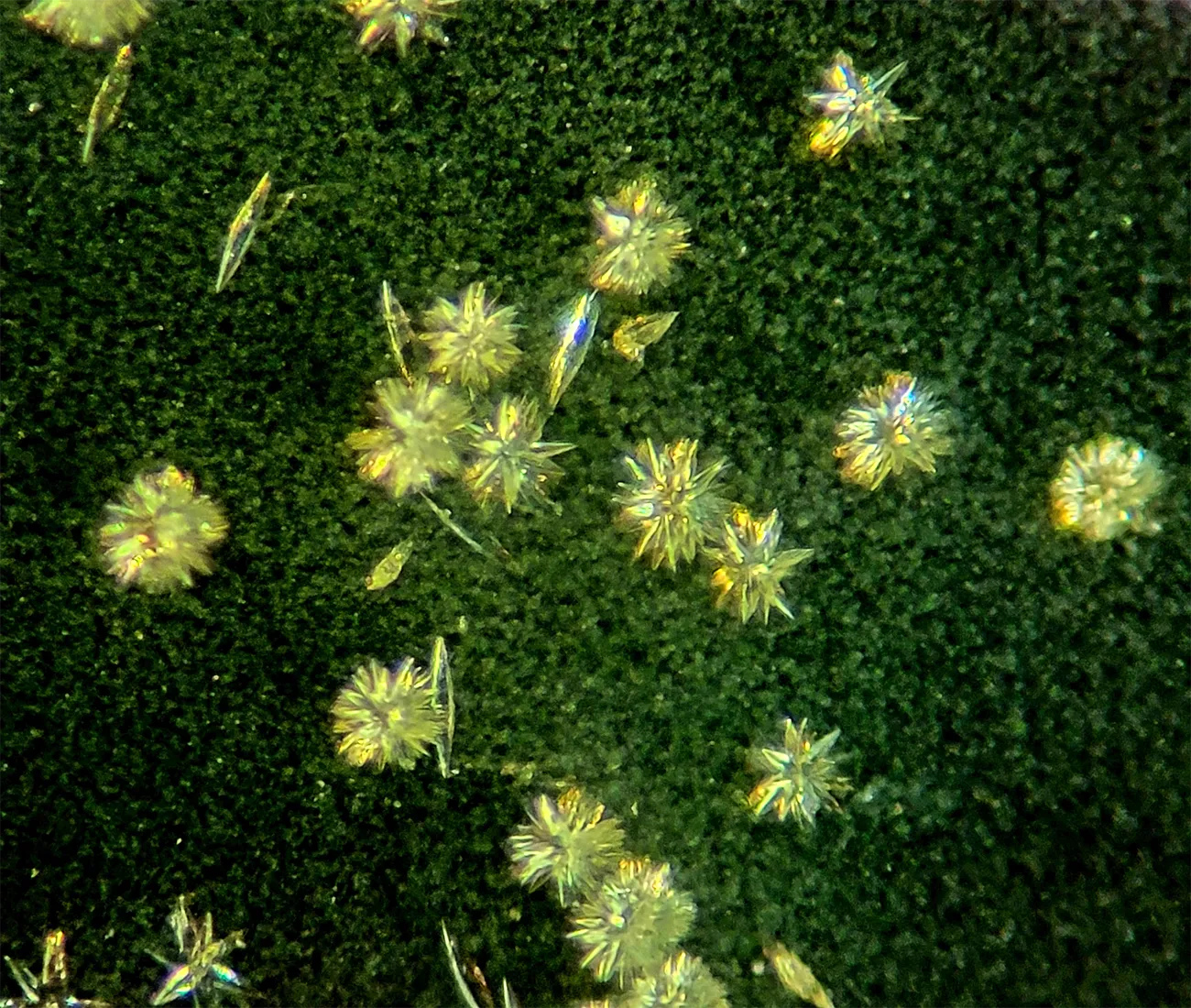
On a magnetic surface, crystals of an RNA precursor called RAO can form as either left- or right-handed structures.
S. Furkan Ozturk
But the real key to creating homochirality is to look at how the effect could have been amplified across a network of interacting molecules. “The most important aspect of all this is not that we managed to find yet another way to get a chiral product,” Sasselov said, but that his group had found a route to creating a homochiral network.
In a paper featured on the cover of The Journal of Chemical Physics in August, Ozturk, Sasselov and Sutherland proposed a model for how chiral information might propagate across a prebiotic network. Sutherland and his group had previously shown that analogs of right-handed transfer RNA molecules — which bind amino acids and bring them to the ribosome to make proteins — link to left-handed amino acids 10 times faster than to right-handed ones. The finding suggests that chiral RNA preferentially makes proteins of the opposite chirality, as is seen in nature. As the researchers wrote in the paper: “Therefore, the biological homochirality problem may be reduced to ensuring that a single common RNA precursor (e.g., RAO) can be made homochiral.”
The study didn’t directly explain why life’s preferred nucleotides are right-handed and its amino acids are left-handed, Ozturk said. But these new findings suggest that the determining factor was the magnetization induced by the Earth’s field. Athavale noted that even if the crystallization process happened in 100 primordial lakes, Earth’s magnetic field would ensure that they all produced precursors with the same handedness rather than a mixture.
Joyce noted that there’s a “cool little twist” if the magnetic field gave such a bias: If life started in the northern hemisphere and favored molecules with one handedness, then it would have shown the opposite handedness if it had arisen in the southern hemisphere.
The propagation of chirality between families of molecules is still highly hypothetical, Athavale noted, though it’s good to get people thinking. Sasselov agrees. “The idea of this paper is to motivate people to go and do these experiments,” he said.
Wentao Ma, an origins-of-life researcher at Wuhan University in China, said that the new papers mark “interesting progress.” But he would need to see the CISS effect lead to the polymerization of RNA to see it as a complete answer. “If they can achieve this result, I think we’re not far away from the … solution,” he said.
“I really like the CISS effect,” said Noémie Globus, an astrophysicist who is working on the homochirality problem. What would be more persuasive, she said, would be for the researchers to check whether meteorites containing an excess of amino acids with a particular handedness (which have been found before) also contain excess magnetic particles. She also noted that different theorized mechanisms could all have been creating homochirality in different molecules.
Jeffrey Bada, an emeritus professor at the Scripps Institution of Oceanography at the University of California, San Diego, is skeptical of the idea. He doesn’t believe that RNA could have been synthesized in primordial conditions as the first self-replicating molecule. “No one’s made RNA in a prebiotic context,” he said, because there are too many issues with the stability of the molecule.
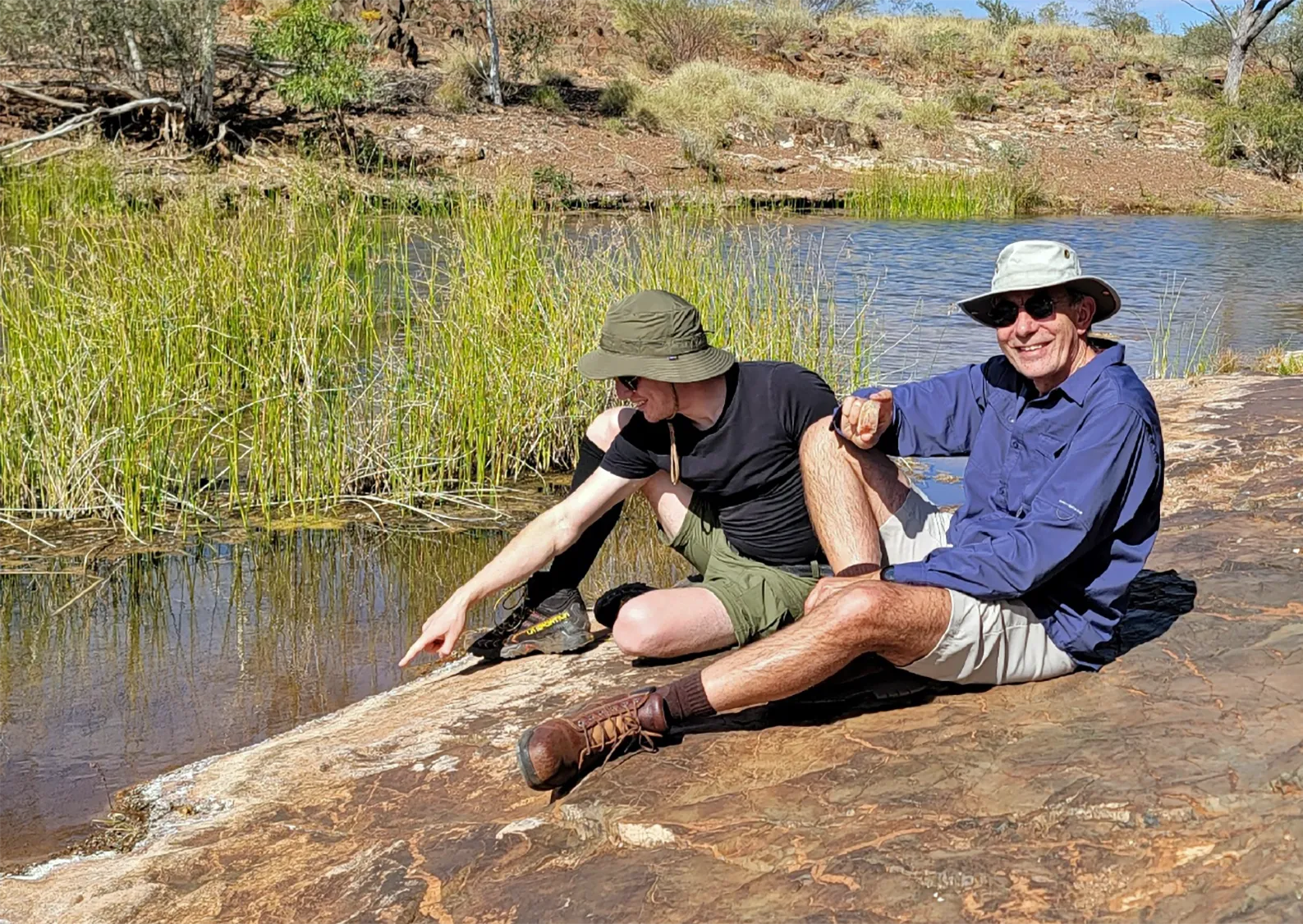
Ozturk and Sasselov survey a site in Pilbara, Australia, which they think might resemble the prebiotic lake in their hypothesis.
S.Furkan Ozturk
Sutherland’s team is still working to show that the other two types of nucleotides can be made from the RNA precursor molecule. “I think we’re pretty damn close,” Sutherland said. “But my group will tell you that I’ve been saying that for 22 years.”
Whether the CISS effect represents the solution, part of the solution or no solution at all, there are obvious next steps to testing it. “It’s got all the aspects of a nice hypothesis where you’re coming up with something creative, something which is feasible, and then something which can ultimately be tested,” Athavale said. The most convincing next step, he thinks, would be to show geological evidence that the process could have happened outside the lab.
Over a Zoom call, Ozturk held up a flat black rock that he had picked up on a trip to Australia, a place filled with magnetic iron rocks on which he’s hoping to replicate his experiments. He also wants to make future tests of the idea more dynamic: The primordial lakes where he thinks the early molecules formed would have had streams and flows of material, as well as natural “wet-dry” cycles driven by rains and high temperatures, that would allow crystals to form and dissolve, form and dissolve.
Though the mystery of homochirality is far from settled, Ozturk has received some enthusiastic encouragement from his mentors for his work on the CISS effect explanation. In April, he gave a talk at Harvard about the Sasselov group’s research, and one of his idols attended. Matthew Meselson, a geneticist and molecular biologist who experimentally confirmed how DNA is replicated, sat in the front row as Ozturk wrote out his findings on a chalkboard. The 93-year-old geneticist told Ozturk afterward that he was so glad he had lived long enough to see this problem being solved. He later gave Ozturk a signed copy of one of his books. “Already you have solved a deep problem,” he wrote in it. “I wish you the best fortune.”
Editor’s note: Sasselov and his group, as well as Joyce and Sutherland, have received funding from the Simons Foundation, which also funds this editorially independent magazine. Simons Foundation funding decisions have no influence on our coverage.