Quark Quartet Fuels Quantum Feud
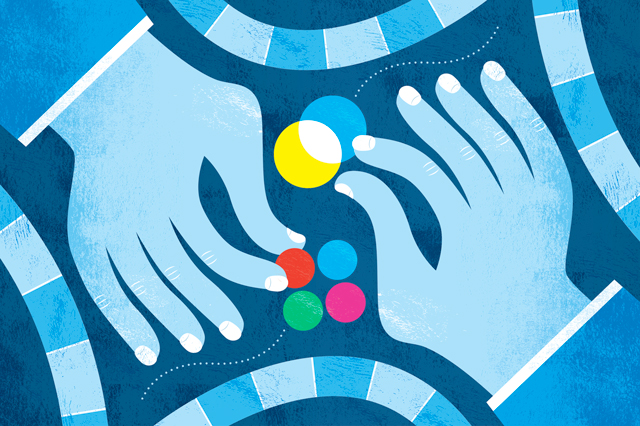
In August 2003, an experiment at the KEKB particle accelerator in Japan found hints of an unexpected particle: A composite of elementary building blocks called quarks, it contained not two quarks like mesons or three like the protons and neutrons that constitute all visible matter, but four — a number that theoretical physicists had come to think the laws of nature did not permit. This candidate “tetraquark” disintegrated so quickly that it seemed a stretch to call it a particle at all. But as similar formations appeared in experiments around the world, they incited a fierce debate among experts about the correct picture of matter at the quantum scale.
Most believed tetraquarks were a new kind of miniature molecule — essentially, two orbiting mesons, each made of one regular quark and one antimatter quark, or antiquark — while a smaller contingent saw them as stand-alone particles in which the two quarks and two antiquarks overlapped in the same small volume of space.
“We hate each other,” said Antonio Polosa, a theorist at Sapienza University of Rome who takes the latter stance, chuckling about the rival factions. “We really hate each other.”
Most critics find the molecular model hard to swallow. “It’s kind of a crystal glass in a nuclear explosion.”
All parties remained uncertain that tetraquarks were real — until one turned up in data from the Large Hadron Collider, the 17-mile, proton-smashing ring near Geneva. Detailed measurements reported in June in Physical Review Letters confirm that the particle, which was first detected in 2007 at the accelerator in Japan and designated Z(4430), is unambiguously a tetraquark. Now, the discovery is forcing physicists to extend their simple picture of quark interactions, or finally replace it with a more nuanced understanding.
And, to mixed reviews, the properties of Z(4430) clearly favor the underdog “diquark model” and the hypothesis that tetraquarks are genuine particles. The existence of such states would suggest a menagerie of exotic “hadrons,” or particles made of quarks, including groupings of more than four. It would also attest to subtle quantum interactions that may shape the cores of hypothetical “quark stars,” the piping hot quark soup thought to have saturated the infant universe, and, closer to home, the proton and neutron building blocks of ordinary matter.
“Other interpretations are really not very tenable, to be honest, with respect to this particle,” said Polosa, one of the originators of the diquark model.
But advocates of the rival molecular model disagree. To their minds, tetraquarks tell a novel but more conservative story of mesons engaging in chemistry below the ordinary atomic scale, without challenging the dogma that two- and three-quark particles are the only hadrons that exist. Z(4430), a tetraquark that looks unlike any combination of two types of mesons mingling as a molecule, certainly “makes it more difficult,” said Marek Karliner, a particle physicist at Tel Aviv University in Israel. But, he said, the diquark model has troubles of its own.
With each model able to account for some of the 20-or-so candidate tetraquarks that seem perplexing from the other perspective, a third view has recently gained ground: the belief that any simple model falls short. “These models might all have some aspect of the truth,” said Eric Braaten, a theoretical physicist at Ohio State University, but like the proverbial blind men encountering different parts of an elephant, “they can’t describe the elephant globally.”
The diquark and molecular models are both attempts to salvage a cartoonish picture of hadrons that worked perfectly, if inexplicably, for the half-century before tetraquarks came along. This “quark model” treats the proton as if it is made of three bloated quarks, each contributing a third to its total mass. However, experiments have shown that the quarks in a proton (and other hadrons) are actually much lighter than their sum, each one mere thousandths of the proton’s mass. The rest of its mass derives from the energy involved in gluing the three quarks together, a stickiness known as the strong force that is conveyed by particles called gluons. “The thing you call the ‘quark’ might have quark-antiquark pairs and glue and all the rest built into it,” explained Thomas Cohen, a physics professor at the University of Maryland.
The exact structure of hadrons is hidden in the folds of a 40-year-old theory of the strong force called quantum chromodynamics (QCD), an easy-to-write-down but infinitely self-referential and thus unsolvable set of equations. No one understands why QCD’s boundless complexity seems equivalent to the quark model, or in other words, why the dynamic confluence of quarks and gluons known as a proton “somehow behaves as if it’s a simple composite of three particles,” Braaten said. Up to now, all hadrons feigned such simplicity. Tetraquarks, which the renowned theorists Edward Witten and Sidney Coleman mistakenly argued in the 1970s were inconsistent with a simplified analogue of QCD, have turned out to be the first manifestations of the theory that aren’t also captured by the quark model.
“The embarrassing fact that tetraquarks have been discovered experimentally and weren’t predicted is an indication that we don’t understand QCD as well as we thought we did,” Braaten said.
Now, rather than abandon the quark model altogether, proponents of the molecular and diquark models hope to extend it to encompass the new discoveries.
In the spirit of the original model, the two proposed extensions pretend that tetraquarks are foursomes of plump quarks. But they present opposite visions for how these components are arranged. According to both QCD and the quark model, quarks have a property called “color,” and they must enter collective states that are color-neutral. The colors of three quarks cancel one another out inside a proton just as, for example, combining the primary colors red, green and blue makes white. And, as with complementary colors like blue and yellow, quarks pair with antiquarks to form colorless mesons. But how do four quarks achieve color neutrality inside tetraquarks?
In the molecular picture, each of the two quarks pairs with one of the two antiquarks, forming two color-neutral mesons that rendezvous momentarily as a meson molecule before flying apart. But in the diquark model, the quarks form a “diquark” pair, as do the antiquarks; both pairs have net color, so they must fuse as a compact, color-neutral particle for an instant before switching partners and dissociating.
The molecular model’s key supporting examples have masses very close to the sum of two separate mesons, implying that these mesons have briefly become bound by the smallest drop of glue (changing their combined molecular mass by a hair) before breaking up. The tetraquark signal found in Japan in 2003, designated X(3872) for its unknown identity and measured mass, exhibited just this mass coincidence; it and a smattering of subsequent examples elevated the molecular model to early prominence.
“The Occam’s razor approach says try the simplest thing,” Karliner said. “If it works, then that’s what it is.”
But with the recent discovery of Z(4430), the razor has turned against the molecular model. The tetraquark’s mass and other meticulously measured characteristics, such as a property called “spin-parity,” do not match up with those of any two mesons. To its critics, this kills the molecule idea. As for its holdouts, “the importance of the evidence against them hasn’t sunk in yet,” said diquark proponent Richard Lebed of Arizona State University. “There’s a certain resistance, if you’ve spent a long time working on a particular picture, to evidence that it’s not right.”
Although Lebed finds it mildly perplexing that so many tetraquark candidates have almost exactly the masses of known meson combinations, other diquark supporters call these coincidences unavoidable: With no less than 12 varieties of quarks and antiquarks, some of their four-way combined masses are bound to accidentally fall close to those of meson pairs, they say.
And, ultimately, most critics find the molecular model hard to swallow. How could a fragile construct of two mesons hold together with so little glue in the middle of a near-light-speed collision of protons at the Large Hadron Collider? “It’s kind of a crystal glass in a nuclear explosion,” Polosa said.
The diquark picture may be pulling ahead in the aftermath of the Z(4430) discovery, but it too remains incomplete. Recently, adherents like Polosa have been working to round out the model with new symmetry rules that would explain why certain quark combinations seem to be permitted and others not, a pattern that was much better captured by the molecular model. Meanwhile, in a new paper accepted for publication in Physical Review Letters, Lebed and colleagues attempt to explain why the colorful diquark and antidiquark pairs might form in the first place and why they quickly decay. If correct, these rules will influence other predictions about hadron physics, such as whether exotic, quark-filled stars exist. They would also deepen the understanding of familiar hadrons like protons and neutrons.
But not everyone feels satisfied with embellishing the diquark picture until it works.
“These models have lots of knobs in them,” Cohen said. “When it gets things right, you declare victory, and when it doesn’t quite get things right, you start turning knobs.” The dispute between the two models, he said, is about figuring out “which one is the better starting point for describing the data reasonably well” — not whether either one is true.
Physicists like Cohen and Braaten believe the full spectrum of tetraquarks can only be predicted by better approximating the unending equations of QCD, an effort that would also elucidate other unknown features of quarks and gluons, such as their behavior milliseconds after the Big Bang. In a recent paper in Physical Review D, Braaten and colleagues suggested an approximation scheme operable on a supercomputer that they think could work for predicting tetraquarks.
The advantages of such an approach might be marginal, however. In 40 years, researchers have failed to build abridgments of QCD that fit the data much better than the naive quark model. Many theorists prefer to extend this simple and effective understanding rather than enter a QCD quagmire.
So the question remains: In future diagrams of tetraquarks, will the quarks be shown hand-in-hand with quarks or with antiquarks?
“There’s going to be a picture that’s substantially more successful than others once all the data is known,” Lebed said. “But I would not at all be surprised if there were still a few nagging mysteries.”
This article was reprinted on Wired.com.