Ocean Bacteria Reveal an Unexpected Multicellular Form
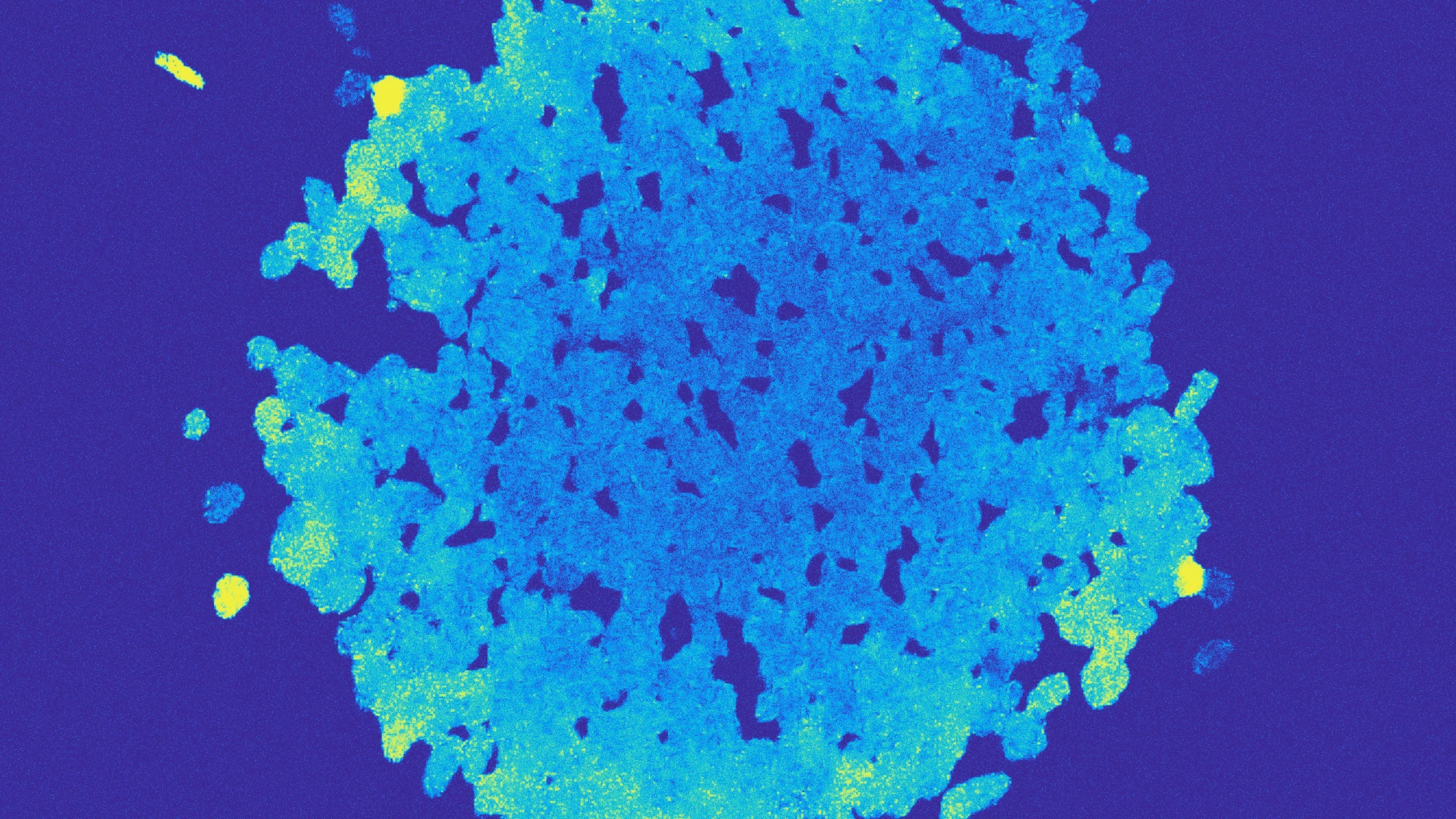
Hundreds of Vibrio bacteria join to form spherical structures like this one shown in cross section. The artificial color differences, which reflect the amount of nitrogen present, reveal that the orb is a hollow sphere with cells sealed together on the outside and mobile cells inside.
Julia Schwartzman, Ali Ebrahimi and Grayson Chadwick
Introduction
Close your eyes and imagine bacteria. Perhaps you’re picturing our intestinal Escherichia coli, or the shiny golden balls of staphylococcus, or the corkscrewing ringlets of Lyme disease spirochetes. Regardless of the species and its shape, chances are your mind’s eye conjured up a single cell, or maybe several free-living cells.
The problem with this image, says the microbiologist Julia Schwartzman, is that it doesn’t reflect how most bacteria are likely to live. Often, bacteria use sticky molecules to anchor themselves to a surface, growing in large, stable collectives called biofilms. The plaque on your teeth is a biofilm; so too are infections on catheters, the slimy green of pond scum and the gunk clogging your bathtub drain.
But Schwartzman’s recent work, which she conducted as a postdoctoral fellow in the lab of Otto Cordero at the Massachusetts Institute of Technology, shows that even bacteria floating in the open ocean, which lack an anchoring point for forming large conglomerates, exist in multicellular forms.
“We saw these structures that were just incredible,” she said.
As Schwartzman, Cordero and their colleagues showed in their recent paper in Current Biology, these multicellular forms arose because the bacteria developed a life cycle far more complex than is usually seen in unicellular organisms.
Company for Dinner
Schwartzman came to these discoveries about multicellularity in marine bacteria while trying to learn about something more fundamental: how they eat.
In the open ocean, often the only energy source for marine microbes is a gelatinous carbohydrate called alginate. Unlike glucose, fructose and other simple sugars that can readily cross a cell membrane, alginate is made up of long, coiled strands that are often larger than the bacteria dining on them. Schwartzman wanted to know more about how the bacteria feast efficiently, since the digestive enzymes they secrete to break down the alginate could easily be diluted and swept away in the open ocean waters.
That’s why she and Ali Ebrahimi, another postdoc in Cordero’s lab, started measuring the growth of the luminescent marine bacterium Vibrio splendidus in flasks of warm broth laden with alginate. In many microbiology experiments, scientists provide microbes with a smorgasbord of nutrients to encourage the cells to divide as quickly as possible, but Schwartzman and Ebrahimi’s flasks forced the Vibrio bacteria to subsist on relatively small amounts of oversize alginate polymers, just as they do in the sea.
Yet when Schwartzman started collecting data, she thought she had made a rookie mistake. As bacteria multiply, they transform the clear, amber-colored culture broth into a murky stew. By measuring the murkiness, Schwartzman could extrapolate the number of microbes in the flask and construct a growth curve to estimate how rapidly the cells were dividing. Bacteriologists have been estimating growth rates this way for decades. As a postdoc, Schwartzman had lost count of how many times she had done this over the years.
The growth curve for her Vibrio cultures, however, didn’t show the usual smoothly rising line but rather a bumpy squiggle like the track of a roller coaster. No matter how many times she repeated the process, the bacteria didn’t produce the expected cloudiness in the broth.
A Microscopic Snow Globe
To check what was going on, Schwartzman deposited a droplet of the culture solution on a glass microscope slide and peered through the lens at 40 times magnification. What she and Ebrahimi saw were not swarms of individual Vibrio but rather beautiful, layered orbs consisting of hundreds or thousands of bacteria living together.
“It wasn’t just a blob of bacteria,” Schwartzman said. “It’s a spherical thing, and you can see the cells mixing in the middle.”
Further work showed that the hollow spheres were Vibrio’s solution to the complicated challenge of eating at sea. An individual bacterium can produce only so much enzyme; breaking down alginate goes much more quickly when Vibrio can cluster together. It’s a winning strategy, Schwartzman says — up to a point. If there are too many Vibrio, the number of bacteria outstrips the available alginate.
The bacteria resolved the conundrum by developing a more complex life cycle. The bacteria live in three distinct phases. At first, an individual cell divides repeatedly and the daughter cells huddle in growing clumps. In the second phase, the clumped cells rearrange themselves into a hollow sphere. The outermost cells glue themselves together, forming something rather like a microscopic snow globe. The cells inside become more mobile, swimming about as they consume the trapped alginate. In the third phase, the brittle outer layer ruptures, releasing the well-fed inner cells to start the cycle anew.
In effect, Vibrio become a heterogeneous mixture of cells, with the bacteria using different genes to control their behavior in each phase. As the cells interact with their neighbors in the structure, what emerges is “a surprising amount of complexity,” said Schwartzman, who is launching her own lab at the University of Southern California in January. “The bacteria are constantly taking in information from their environment, and sometimes they respond in ways that change the environment.”
This complexity pays off for Vibrio in several ways. By altering their life cycle to include a multicellular stage, the bacteria can digest the alginate efficiently: Their numbers increase, and the hollow shell helps to concentrate the enzymes. Meanwhile, the structure of the community prevents too many cells from being born. The cells in the shell lose the opportunity to reproduce, but their DNA lives on in the next generation anyway, since all the cells in the orb are clones.
How Common Is Multicellularity?
The work is “a beautiful paper,” according to Jordi van Gestel, who studies the evolution of microbial development at the European Molecular Biology Laboratory and wasn’t involved with the research. Van Gestel says that the results bolster the idea that, far from being the exception, microbial group living is the norm.
“It beautifully illustrates the complexity of the life cycle in such simple bacteria,” he said.
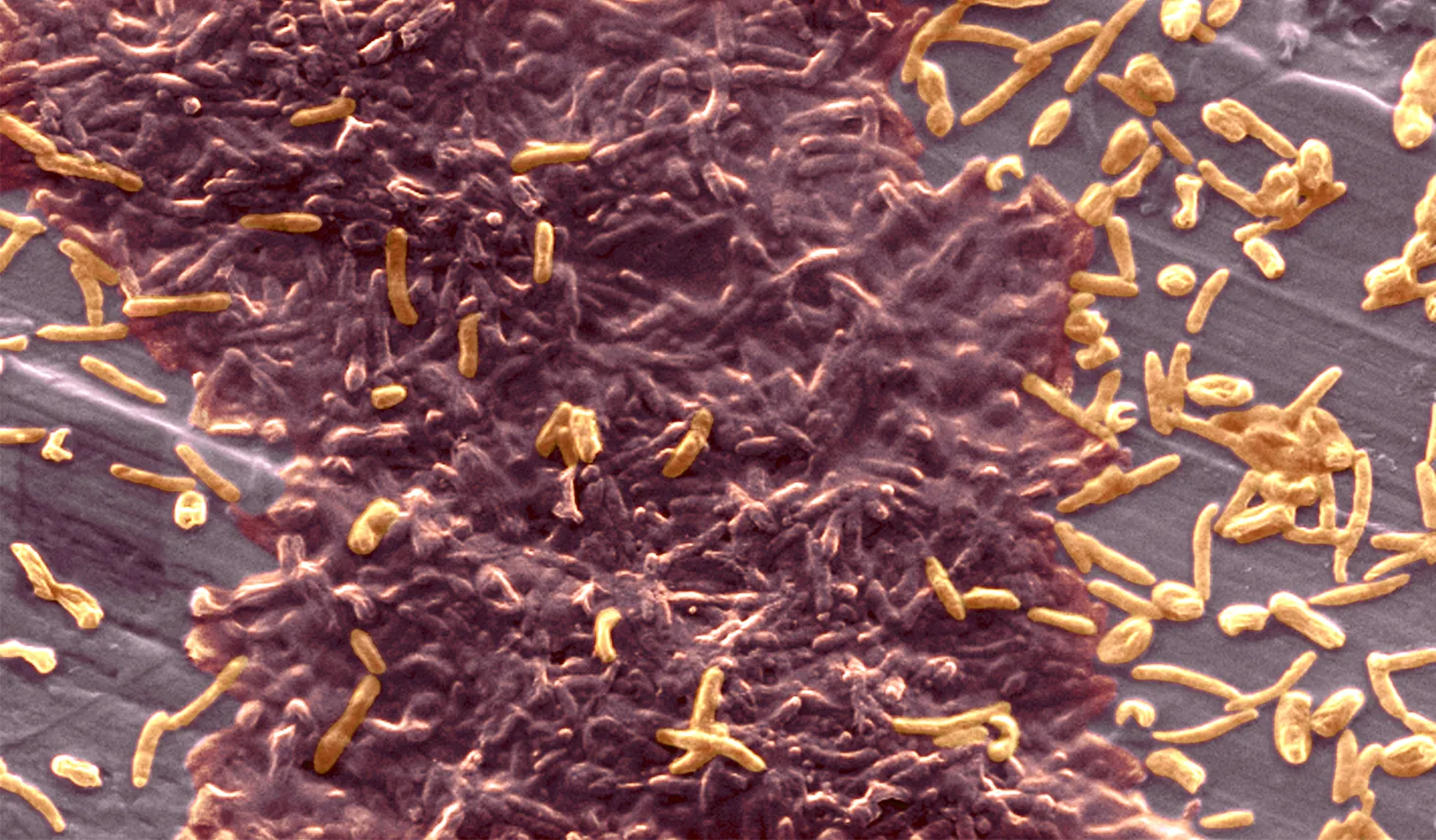
Bacteria growing on surfaces often congregate into complex structures called biofilms. Typically, the solid surfaces help the cells to organize themselves.
Science Source
Anahit Penesyan, a microbiologist at Macquarie University in Australia, says that Schwartzman and Cordero’s work offers a useful challenge to preconceptions about bacteria. “It’s engraved in our understanding that a microbe is just a single cell,” she said, and as a consequence, researchers often aren’t looking for complex behaviors that might dominate microbial life. “It’s like looking at a plant seed or spore and trying to infer what the whole plant is like.”
The new Vibrio finding adds to a growing list of microbes that can become multicellular for at least part of their life. Last year, researchers at the Georgia Institute of Technology reported that unicellular yeasts in their laboratory evolved a huge multicellular form in just two years. And in October, researchers in Japan announced their discovery of bacteria that grow into multicellular structures on the walls of caves; when the rocks are immersed by underground streams, the structures eject specialized cells like seeds to colonize other locations.
Schwartzman and van Gestel both believe that a capacity for multicellularity evolved early in life’s history and is shared with bacteria’s ancient cousins, the archaea, which also seem unicellular. They think it’s just a matter of time until researchers find other species with similar properties — and Schwartzman has already begun looking.
James Shapiro, a retired microbiologist from the University of Chicago, has little doubt that she’ll find it.
Beginning in the 1980s, Shapiro and other microbiology luminaries such as Bonnie Bassler at Princeton University showed that the single-celled lifestyle of well-studied bacteria was often an artifact of the artificial flask environments in which they were grown. In a 1998 article in the Annual Review of Microbiology, Shapiro argued that bacteria aren’t unicellular loners. “I came to the conclusion that basically all bacteria are multicellular organisms,” he said.
Over his four-decade career, Shapiro saw his hypothesis transform from nearly heretical to incontrovertible. “At first, I got just bemused attention, but now it’s become conventional wisdom,” he said. “Multicellularity is an inherent property of bacteria.”
Editor’s note: Cordero is co-director of the Simons Collaboration on Principles of Microbial Ecosystems. The research by Schwartzman, Cordero and their colleagues was supported through that collaboration by the Simons Foundation, which also sponsors this editorially independent magazine.