An Explorer of Abyssal Depths Looks to Oceans on Other Worlds
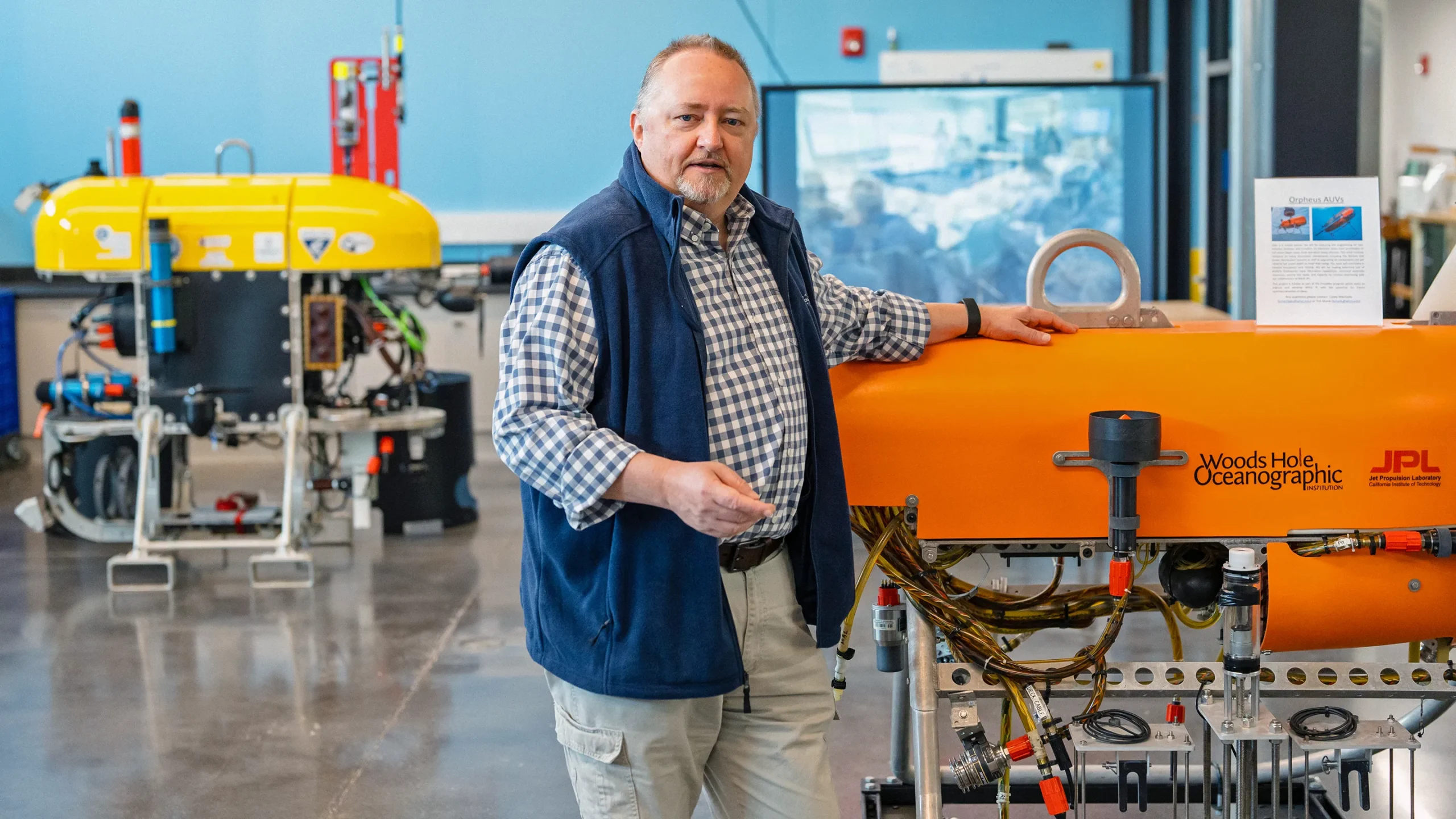
In a facility at the Woods Hole Oceanographic Institute (WHOI), researcher Chris German stands beside a remotely operated vehicle that will be able to descend 11,000 meters to the bottom of the deepest ocean trenches.
Ian MacLellan for Quanta Magazine
Introduction
As a boy growing up in Rochester, England, Chris German knew of his family’s strong maritime tradition, and he had no intention of continuing it. One of his grandfathers had served in the Royal Navy for much of his life, while the other had worked in the Chatham Naval Dockyard, as did German’s father and two uncles. Yet even though German attended a school founded in 1708 to train future ocean navigators, he vowed never to go to sea.
German also had strong opinions about other careers he would never consider. He disliked geology, which in his limited experience meant walking through malodorous mud flats in the Thames Estuary with his mother and brother, sifting through the muck for fossils. Biology was another subject for which he had little enthusiasm.
Young German might therefore have been deeply disappointed to learn that his adult self would become a marine geochemist. That choice has nonetheless been a boon for advancing our scientific understanding of the deep-sea realm. German, now a senior scientist at the Woods Hole Oceanographic Institution, has perhaps done more than anyone to explore hydrothermal vents — fissures in the ocean crust that discharge hot, mineral-rich fluids into the seas.
“He’s a genius in working at abyssal depths and finding hydrothermal vents and the biological communities they support,” said Adam Soule, an oceanographer at the University of Rhode Island.
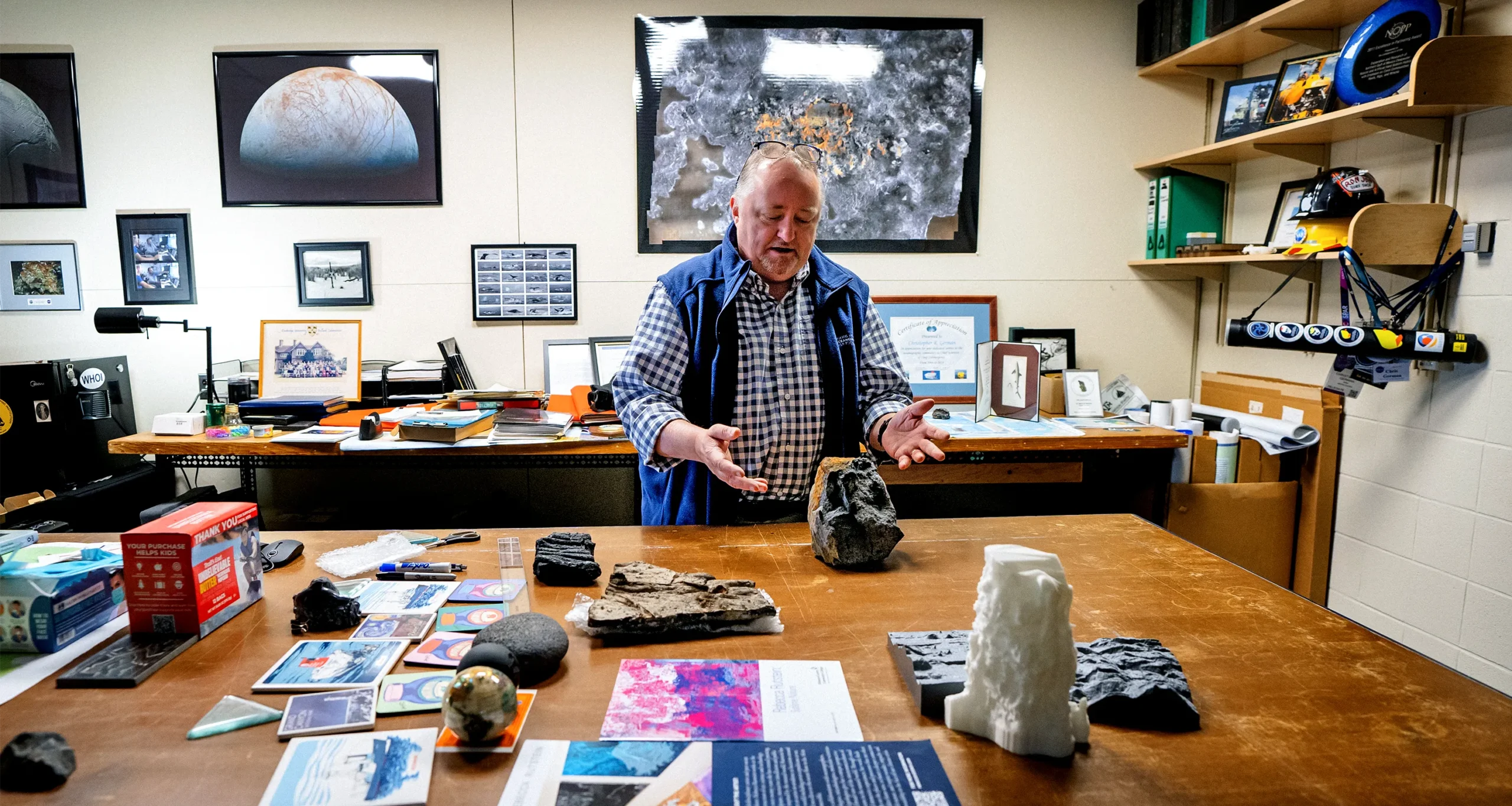
The workbench in German’s laboratory at WHOI is covered with samples and images collected during his past expeditions to hydrothermal vents around the world. The photos of frozen moons on the wall attest to the strange oceans he hopes to explore.
Ian MacLellan for Quanta Magazine
The first hydrothermal vents were spotted by scientists about 250 miles from the Galápagos Islands in 1977, when German was in high school. For years thereafter, the prevailing view was that hydrothermal vents existed only in the Pacific Ocean. German helped to change that: He was the first to discover vents off Antarctica and in the Arctic Ocean. He surveyed some of those sites and others firsthand in Alvin and other submersibles, but he has also developed sensors, robotic probes and other instruments to take pictures, measurements and samples from undersea environments more remotely.
Life and the potential for it have figured prominently in those seafloor discoveries. More than 600 new species have been found in thriving ecosystems near vent sites that are completely cut off from sunlight and the fruits of photosynthesis. In 2012, German led an expedition to the Mid-Cayman Rise — the spreading center of an underwater mountain range (or mid-ocean ridge) at the base of the Caribbean Sea, where tectonic plates are moving apart. There, for the first time, he and his colleagues witnessed underwater abiotic synthesis — the creation of organic molecules, the building blocks of life, through entirely non-biological processes.
“That was the starting point for my involvement in astrobiology,” German said. “Discoveries like that one helped NASA realize there are underwater environments they care about and [that] could become important in future exploration for life beyond Earth.”
In 2020, German was asked to lead a five-year, $7.6 million NASA-funded project called Exploring Ocean Worlds, charged with devising strategies to search for life in ice-covered oceans on Europa, Enceladus, Titan, Triton and other bodies in our solar system. The technical challenges are daunting, he acknowledged, “but NASA doesn’t have to reinvent the wheel from scratch because we have a lot of experience trying to solve those problems here in the Earth’s oceans.”
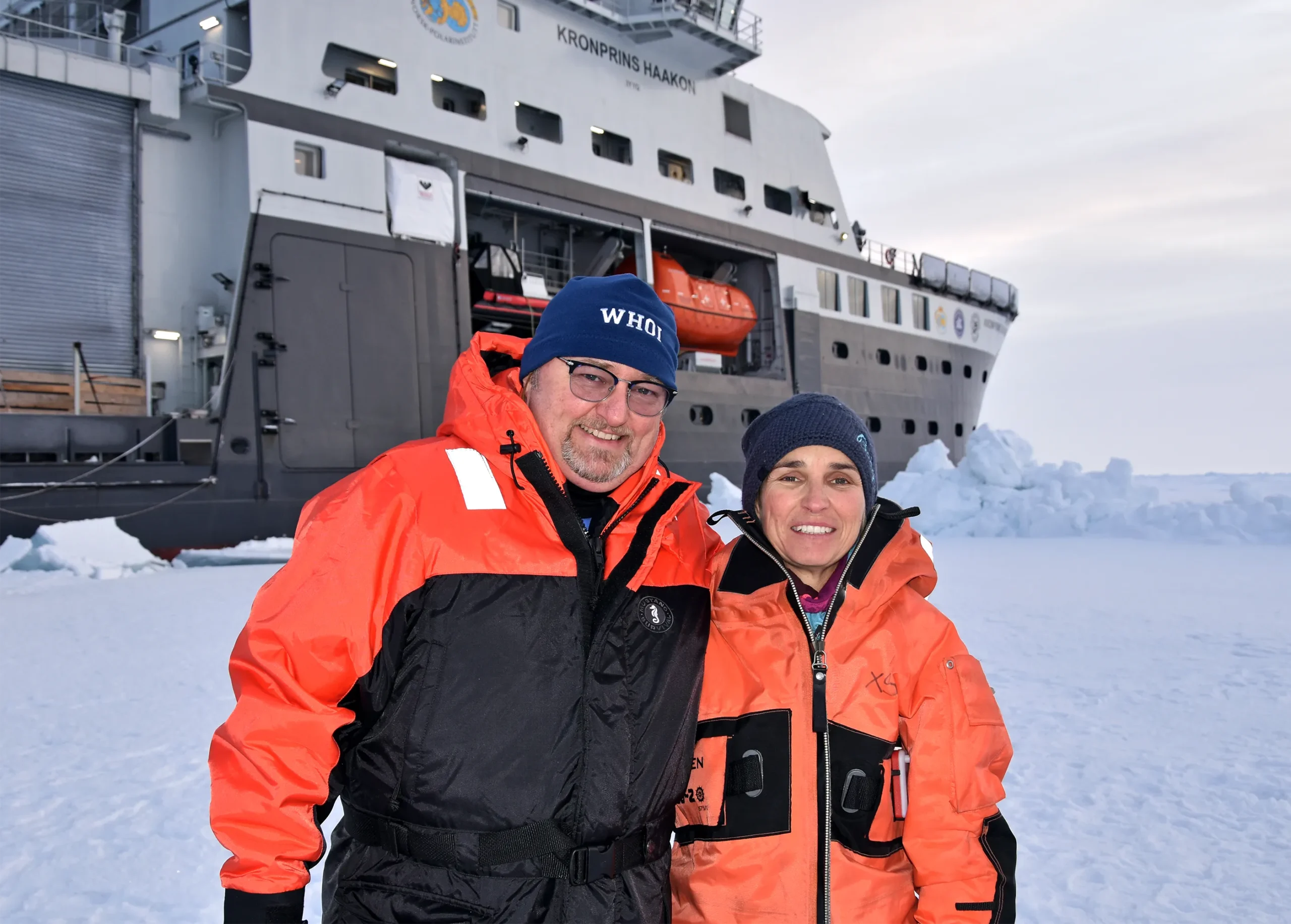
On the ice 4,000 meters above the Aurora hydrothermal vent field in the Arctic, German stands beside the Norwegian research vessel Kronprins Haakon with Eva Ramirez-Llodra of REV Ocean, the chief scientist on the expedition, in 2019.
Courtesy of Chris German
German spoke with Quanta from Woods Hole, Massachusetts, about his deep-sea adventures in a series of conversations between his excursions to the Arctic Ocean and an active underwater volcano near Hawai‘i. The interview has been condensed and edited for clarity.
How did you end up doing largely the opposite of what you expected for your career?
I entered the University of Cambridge as an undergraduate in 1981 intent on becoming a chemical engineer, but that changed pretty quickly. In my very first week, a lecturer who happened to be a giant in the field of plate tectonics talked to us about the evolution of the Alps. I had only been out of the U.K. once before, on a family trip to the Swiss Alps, where I was blown away by the beauty of nature. When I learned that the theory explaining all that was part of geology — a field that would allow me to go out and study volcanoes and earthquakes — I decided that would be a lot more exciting than working at an oil refinery or chemical plant.
During my second year, I was drawn further into the subject when Steve Sparks, a leading volcanologist, lectured about the Mount St. Helens eruption, which had recently made headlines. In my third year, I learned about ocean chemistry from Harry Elderfield. The idea of combining my interests in chemistry and volcanology with the Earth’s oceans sounded intriguing, so I made geology my major rather than minor. In 1984, I began graduate studies in marine geochemistry under Elderfield’s supervision.
When did you become interested in hydrothermal vents?
The first black smoker, the most spectacular kind of hydrothermal vent, was discovered in the Pacific in 1979 and reported on in a 1981 paper. Black smokers are spectacular features on the seafloor, spewing the hottest, darkest plumes out of “chimneys” formed from mineral deposits that can be nearly 200 feet tall. In 1985, Elderfield and others from Cambridge were part of a team that discovered the first black smoker in the Atlantic Ocean, on the Mid-Atlantic Ridge. They came back all excited, and some of that clearly rubbed off on me. I signed up for my first cruise a year later.
How did that first trip at sea go?
Before setting off, I told my colleagues that I hoped I liked it, because I was already pretty far down the path of my Ph.D. work.
We sailed from the Canary Islands to the mid-Atlantic, above the TAG [Trans-Atlantic Geotraverse] vent site, which is about as far from land as you can get in all directions. I was queasy, but by the third day, I got my sea legs. So maybe there was something in my genes after all.
Along the way, we passed a Woods Hole ship, the Atlantis II, which was carrying the Alvin sub. “I would never go down in that, would you?” a shipmate asked me.
“I hope I never get the chance,” I replied, “because if I’m asked, I couldn’t say no. Though I really do suffer from claustrophobia.”
A few years later, I was asked to do just that.
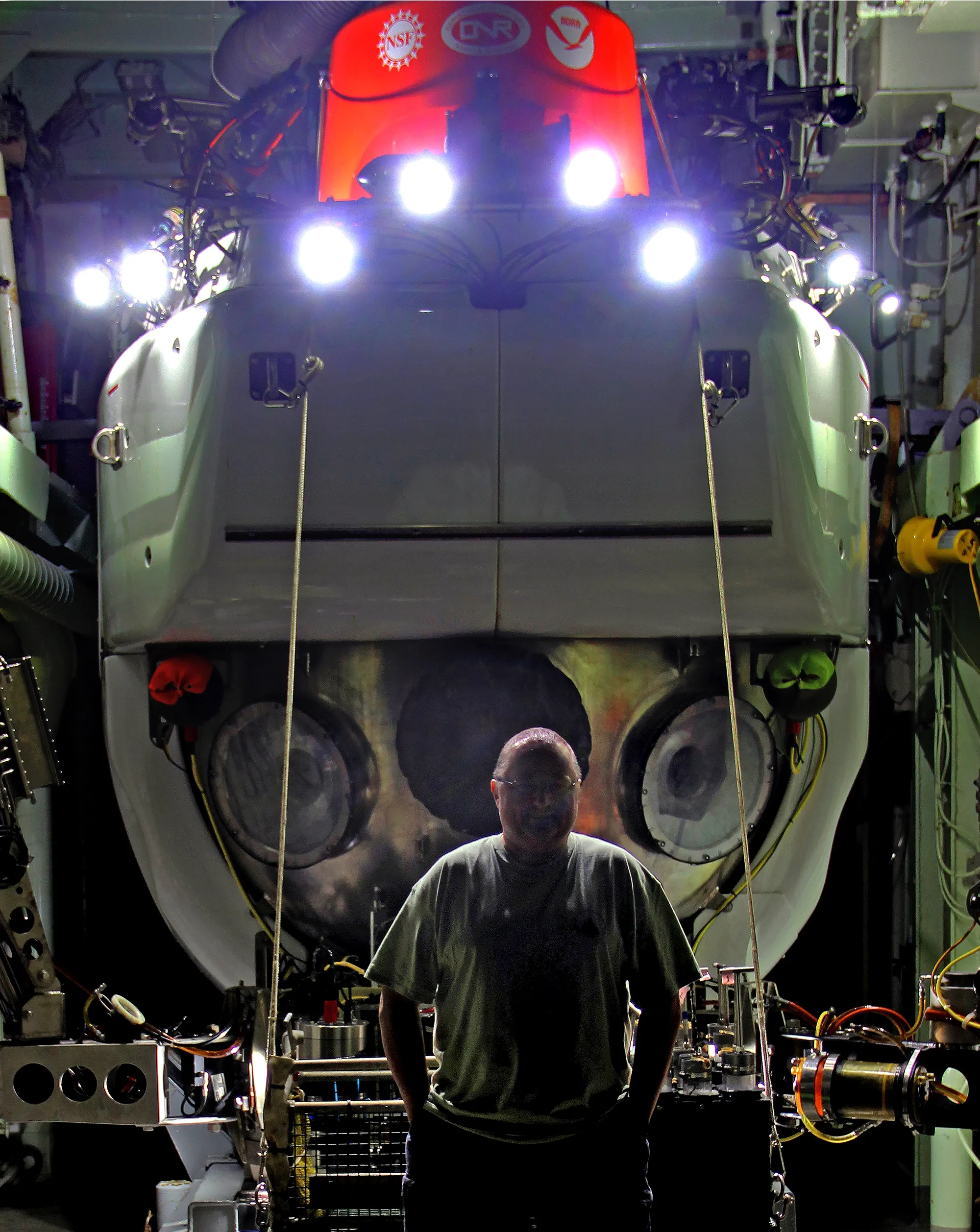
German in the lights of the Alvin submersible while aboard the research vessel Atlantis in 2014. He descended in the Alvin for his first glimpse of a black smoker in 1989.
Courtesy of Chris German
What was it like being in the Alvin?
This was in 1989, midway through my two-year postdoc at the Massachusetts Institute of Technology, where I worked with John Edmond — a co-discoverer of the first low-temperature hydrothermal vents on the 1977 Galápagos cruise. Edmond and I went back to TAG, and this time, thanks to Alvin, I got to see a black smoker up close. Alvin had been to this area before, but the pilot couldn’t figure out a safe way to get close enough to obtain good samples. The top of a black smoker is like a fire hydrant with the top knocked off. There’s a strong flow that you could get swept up in, and the water coming out can be up to 400 degrees Celsius.
The trick Edmond and I came up with was to get down deep, starting at the base of the chimney where things are relatively calm, and then cautiously move upward. That’s how we were able to get the first really good samples from that site — water laden with sulfur, iron, copper, zinc and lead, which is what makes it look black. That was the first successful sampling from a hydrothermal vent anywhere in the Atlantic.
Just being inside the Alvin was an experience. Only two scientists and a pilot can fit in it, and you’re down for just eight hours. Time passes quickly because it’s all so overwhelming. The chances are great that you’re seeing something no one else has seen before. And getting so close to a black smoker was incredibly exciting. You can’t drive your car right up to a hot spring at Yellowstone. And this spout at the bottom of the ocean, unlike Old Faithful, has been going off continuously for thousands of years. That speaks to the power and energy locked up inside our planet.
During my two years at MIT, I developed a passion for studying hydrothermal vents. The question I grappled with was: If I leave and return to the U.K., how can I play a role that is new and original?
How did you intend to contribute to this area?
Remember that when I had started graduate school just a few years before, it was still widely believed that there weren’t hydrothermal vents in the Atlantic. We knew that wasn’t true, but I wondered how many hydrothermal fields there were on this planet, and what were the most efficient ways of searching for them.
I realized that although a vent itself is typically only about the size of a football field, the plume coming out of it is like a mushroom cloud that rises into the water column and expands. Even after getting diluted by a factor of 10,000, the concentrations of iron, manganese and other metals are still 100 times greater than in ordinary seawater. We could find evidence of these plumes without doing any chemical measurements simply by using optical sensors to measure the cloudiness of the water. And because of the way the plumes spread, these features could sometimes be detected from hundreds of kilometers away.
After finishing my postdoc in 1990, I returned to the U.K., taking a job at the National Institute of Oceanography in the village of Wormley. I no longer had access to a submarine like Alvin, but a group at the institute had just developed a towable instrument that used sonar to map the seafloor. I said that if we put my optical sensors on that vehicle, I could figure out where all the hydrothermal activity is.
The first time we used this approach, we found six new vent sites in the Atlantic, where only two had previously been known. It was no longer a matter of stumbling upon things by accident; we could go about it systematically.
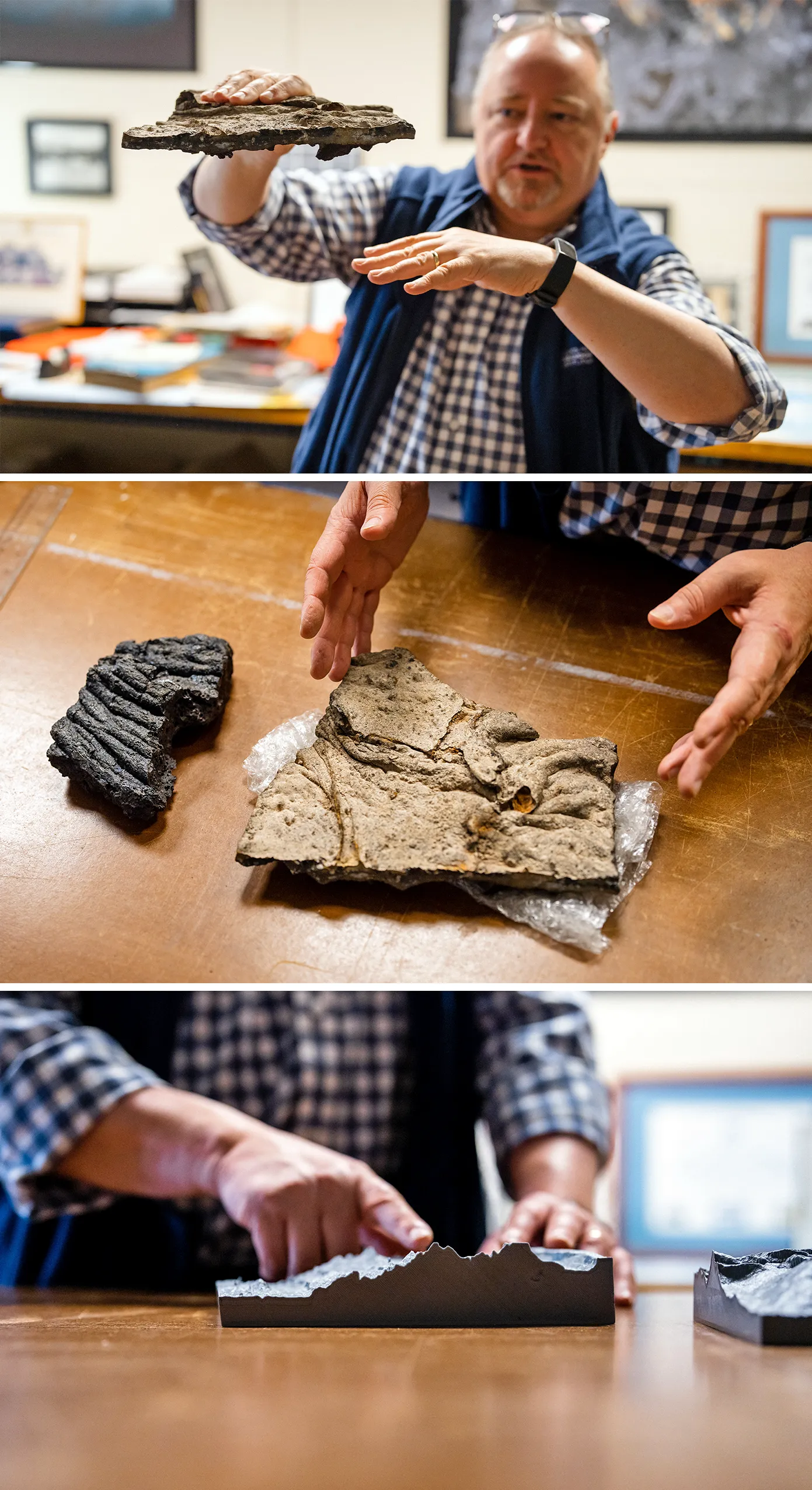
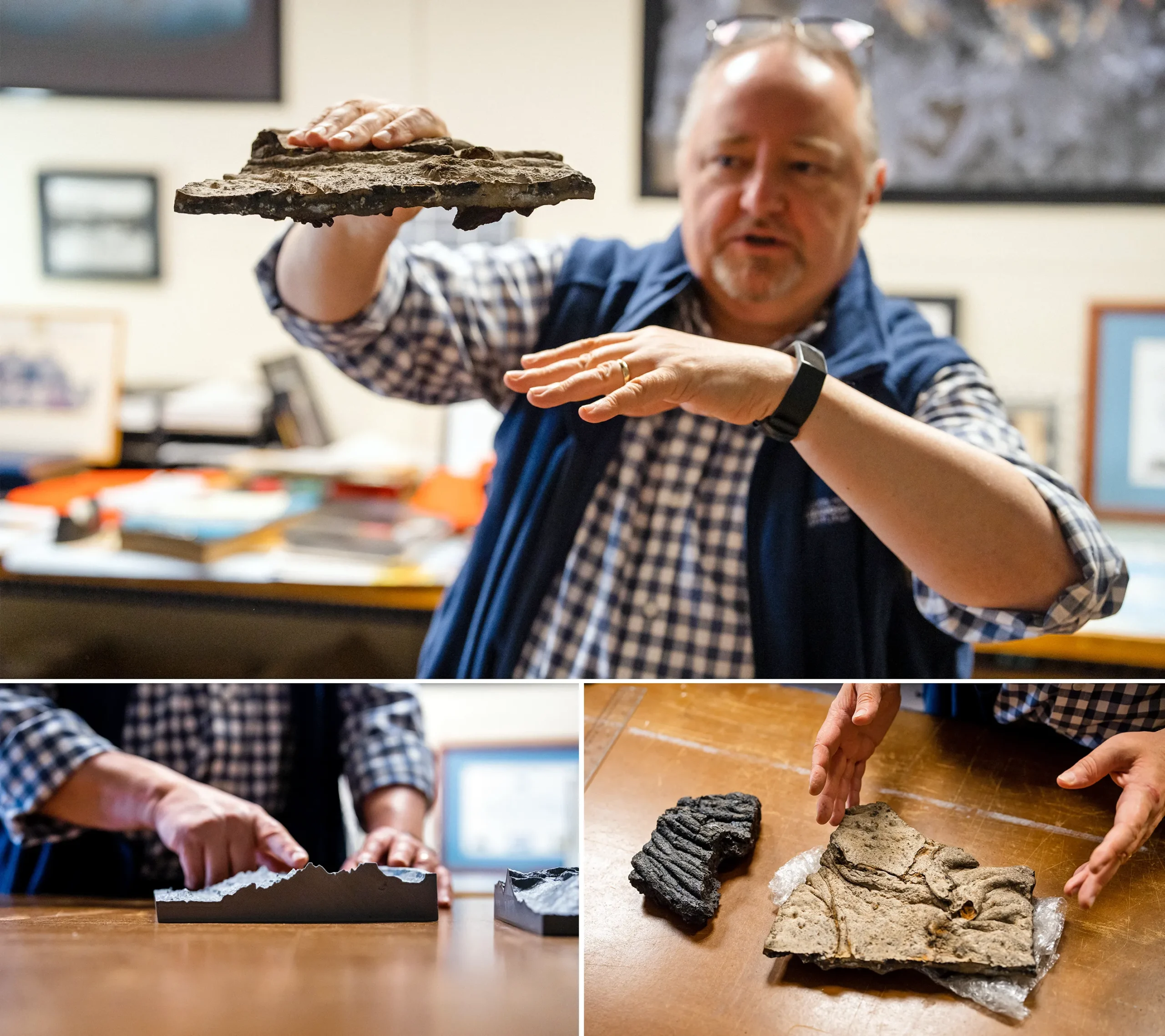
At top, German uses a recovered fragment to demonstrate the structure of the seafloor at the Mid-Cayman Rise. The slab contrasts with a dark chunk of lava that his team recovered from an underwater volcano near Hawai‘i (bottom left). On a miniature 3D model of the vent site (bottom right), the spindly stone chimneys that arise around the vents feel sharp to the touch.
Ian MacLellan for Quanta Magazine
What was the next big advance in the search for hydrothermal vents?
In the early 1990s, conventional wisdom held that there was no hydrothermal activity in oceanic ridges that were “slow-spreading” or “ultraslow-spreading,” where the tectonic plates move apart at only about 10 to 50 millimeters per year. (Plate movement in fast-spreading ridges is 10 times faster.) I didn’t see why that had to be true, and in 1997 we took our optical sensors to the ultraslow Southwest Indian Ridge in the Indian Ocean — one of the slowest-spreading ridges known. We found six hydrothermal vent sites there. Two years later, we found hydrothermal activity near Antarctica in an even more remote part of the unexplored ocean. By that point, I felt secure in believing that these vents can exist anywhere. Which meant I needed something new and different to do.
And you found inspiration in the international Census of Marine Life, a decade-long (2000-2010) effort to catalog all life at sea?
Exactly. Hundreds of new species had already been discovered at hydrothermal vents since 1977, and the rate of discovery was not — and still is not — slowing down. At every new vent site, we find new species. At the base of the food webs in these communities are chemosynthetic microbes that derive energy from chemical reactions rather than sunlight. That fact has piqued NASA’s interest in ice-covered oceans on other worlds, where there is no photosynthesis, yet there still are conditions, including the presence of hydrothermal activity, that may give rise to life.
Describe some of the research you’ve done with NASA’s backing.
My first funding from NASA supported four cruises, from 2009 to 2013, to the Mid-Cayman Rise in the Caribbean. We found that large amounts of hydrogen were released from these vents, which permitted the synthesis of organic compounds. These compounds, moreover, were newly created — “abiotically synthesized” — and not just recycled from animals. We suggested that this could be how one might go from a geologically active system to one that’s biologically active.
The next step was to work in an ice-covered ocean, like those believed to exist on Europa and Enceladus. I got my chance in 2014 in a trip to the Arctic Ocean on board a German icebreaker, equipped with a battery-powered sub I helped develop. Tethered robotic subs called ROVs [remotely operated vehicles] normally have heavy electric cables that hang vertically and only allow them to move 50 meters sideways, which doesn’t work well in an ocean where the ice is always moving. Our battery-powered sub has a thin fiber-optic cable for data and communications that enables it to go many kilometers sideways. We saw a black smoker — the first hydrothermal vent ever observed in the Arctic — just two hours before the mission ended.
I returned to that site, the Aurora vent field, on a Norwegian icebreaker in 2019 with a better camera that enabled us to see some vent animals. Our Norwegian colleagues returned in 2021, collecting the first biological samples from that area. I was away exploring the remote Southeast Pacific at the time, or I would have joined them.
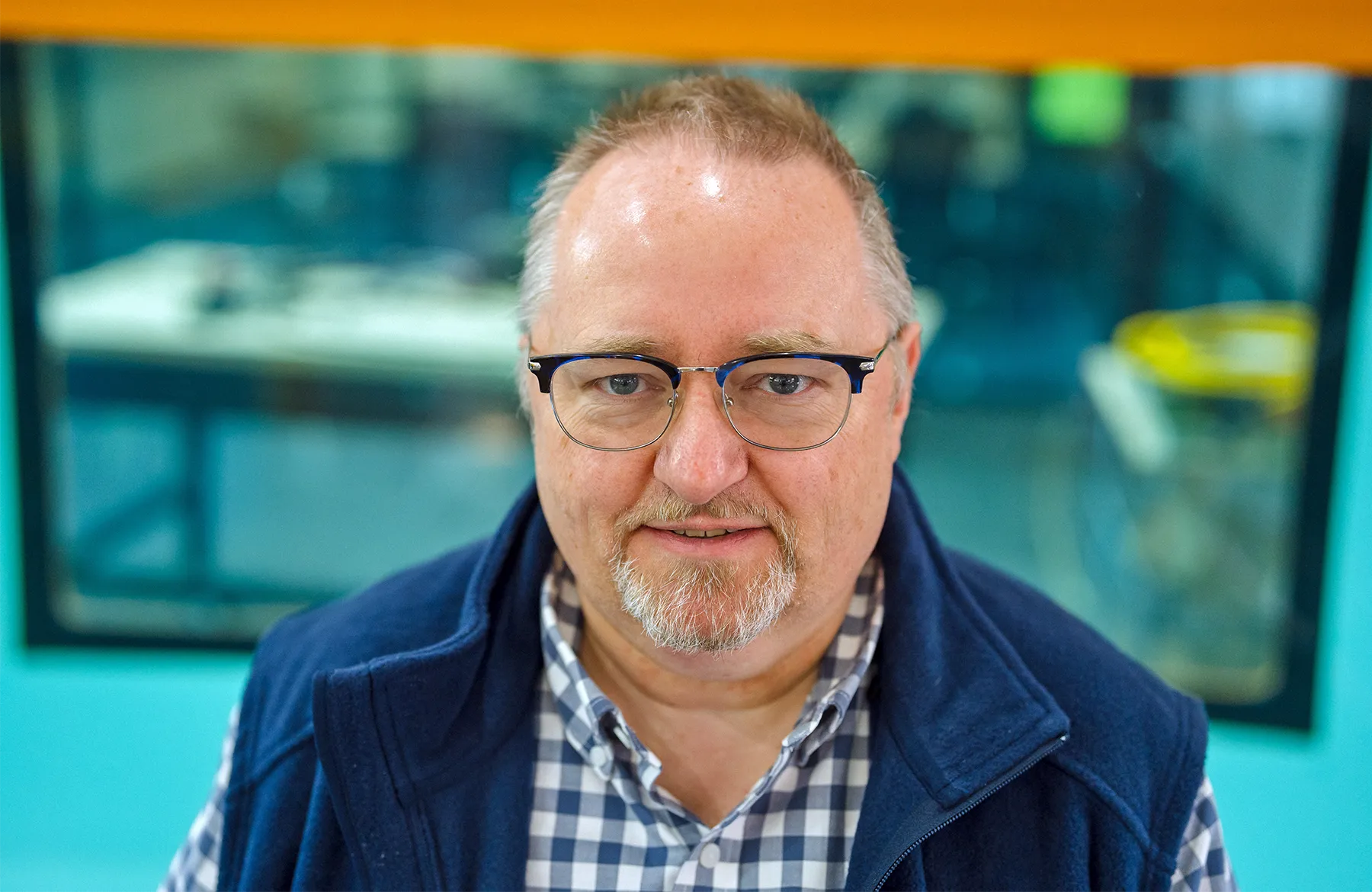
“The oceans are so big, and so little explored, that we’re always pushing the limits of knowledge, which is something you can’t say about every field,” German said.
Ian MacLellan for Quanta Magazine
Why do you consider Aurora a good model for ocean worlds?
For starters, the Arctic is the only ice-covered ocean we’ve got. Moreover, we’ve shown that the vents at Aurora are rich in hydrogen, so all indications are that we have the geological conditions in that it’s an ice-covered ocean that can give rise to the abiotic synthesis of organic compounds. We’re heading back to Aurora later this summer in the hopes of confirming that hypothesis. We also expect to show that these vents harbor some of the most primitive life forms on Earth.
I’ll be spending time this summer in the Arctic with engineers from the Jet Propulsion Lab, working on robots that can cut through ice, which is something we hope to be able to do on Europa someday. Once we get through the ice on another planet, what will we do? Well, we’ll need electronic devices that can work at high pressure, in wet and salty environments that are highly corrosive. That’s something NASA traditionally hasn’t had to worry about, but it’s something ocean engineers think about every day. What we’re working on now, through the Exploring Ocean Worlds project, is to blend those two lines of expertise.
Beyond that, my next priority will be to return to a Hawaiian seamount [underwater volcano] we’ve visited several times before, in part because it has the depth and water-pressure conditions that might be found on the seafloor of Enceladus.
With multiple excursions this year, and in previous years over the decades, what keeps you going back on these ocean voyages?
A big part of it is that it never gets boring. We never stop learning. The oceans are so big, and so little explored, that we’re always pushing the limits of knowledge, which is something you can’t say about every field. Eighty percent of the world’s mid-ocean ridges haven’t been explored for venting. I’m constantly humbled by the fact that there is more going on than I ever considered possible. Most of our planet’s surface is covered by deep ocean, so somebody ought to be paying attention to it.
With the benefit of hindsight, what do you think of the vow you once made about never going to sea?
I’ve learned that it is important to remain open-minded and follow life wherever it takes you. I think that’s pretty good advice in general.