On the Microbial Frontier, Cooperation Thrives
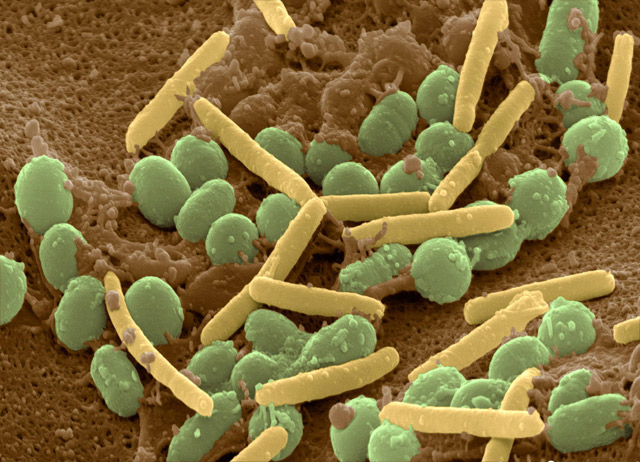
Despite their single-cell status, microbes are capable of striking feats of cooperation: They can secrete polymers that enable them to stick together and form biofilms in order to defend themselves against antibiotics and other poisons. They can manufacture large volumes of lubricants that allow a colony to swarm over soft surfaces, and they can even produce iron-scavenging molecules that allow them to live in iron-poor environments, such as a human host.
This diverse behavioral repertoire poses a major question in evolution: Given the selfish nature of natural selection, how can cooperators triumph? “The classic problem is that any sort of cooperative trait will impose some kind of cost on the individual expressing the trait,” said Michael Desai, a physicist turned evolutionary biologist at Harvard University who studies microbes. “The mystery is how can this evolve?”
Cooperation is by definition a behavior that benefits someone else — giving food or protection, for example — and it typically comes at a cost to the giver. Especially in rapidly mutating organisms like microbes, new modes of cheating regularly arise to outcompete cooperators.
According to the simplest model of natural selection, in a perfectly mixed population of cooperators and cheaters, the latter usually triumphs. But both theoretical work and experiments with microbes and other organisms have shown that cooperation can evolve under certain conditions. Groups of related individuals working together can outcompete the cheaters, hence explaining the myriad organisms — microbes, insects and even humans — that survive thanks to cooperation.
Two studies published in recent months have identified a new force that can help cooperators flourish: the expanding boundaries of a population. Both studies focused on yeast, but scientists say the findings might hold true for other species, including humans. “It’s not clear how generally common this mechanism is, but it seems quite plausible that it’s widespread,” said Desai.
Better understanding of the specific conditions that enable cooperation in microbes might have applications for human health. Many of the microbes that infect humans operate in a cooperative state known as biofilms, and new strategies to prevent biofilm formation may provide alternatives to resistance-prone antibiotics. The findings may also shed light on the evolution of multicellular organisms, which emerged from collections of cooperating cells, and on cancer, which can be viewed as cheater cells attacking the healthy cooperating cells of our bodies.
New Territory
Much of the theoretical work on the evolution of cooperation has focused on static populations that live in a fixed place or maintain a constant size.
Scientists have known for years that the spatial arrangement of a static population can encourage microbial altruism. Though cheaters win in a thoroughly mixed group of microbes, clumps of cooperating microbes can outgrow clumps of defectors. So too can groups encourage cooperation. Two popular and somewhat overlapping theories for the evolution of cooperation include kin selection, in which generosity toward family members aids in the survival of one’s genes, and group selection, in which a group that cooperates is more successful than one that does not. “Cooperative benefits don’t accrue indiscriminately across the population but to individuals who are spatially nearby or genetically related,” said Desai.
But most species do not live under static conditions; they undergo constant shifts in number and territory. Both global warming and geological cycles like ice ages trigger range shifts, for example.
A new wave of studies suggests that population expansion can have a significant impact on the dynamics of evolution. In a growing population, chance effects, known more formally in evolutionary theory as genetic drift, can become more powerful than natural selection. That can enable less fit groups, such as cooperators, to thrive.
A 2007 experiment demonstrated the power of expansion in graphic detail. Oskar Hallatschek, now a biophysicist at the University of California, Berkeley, and his collaborators started with a drop of two thoroughly mixed strains of microbes, fluorescently labeled two different colors, on a petri dish. Because the two strains grow at the same speed, the static-population model predicts that their concentration will remain stable over time; an initial 50:50 mix would stay 50:50. But the results were dramatically different. As the microbes divided, expanding out into the dish, they quickly segregated into a bacterial pinwheel with well-defined sections of color. “It’s a really strong effect and very hard to avoid,” said Hallatschek.
The findings were a striking illustration of a phenomenon called gene surfing, which had been predicted a few years earlier with theoretical simulations. (Many of the researchers are physicists, drawn in part by the potential to model and test theories of evolution.) In large static populations, new neutral mutations (ones that don’t affect evolutionary fitness) are unlikely to become fixed in the population. But according to the surfing model, mutations that occur on the frontier of a growing population are more likely to expand — they “surf” the expansion wave — and take root because only a few individuals are reproducing there. In their 2007 paper, Hallatschek and collaborators explained how genetic drift can drive both gene surfing and the pinwheel pattern: Green bacteria will divide and create more green colonies, creating a widening wedge of green. “In the case of an expanding colony, it’s all about location,” said Hallatschek. “Even if you are a highly fit mutant, you have to be at this frontier to really thrive or you have no chance.”
Hallatschek’s experiments provided the first direct evidence that “surfing can dramatically alter the neutral genetic variability of a large natural population,” said Laurent Excoffier, a population geneticist at the University of Bern in Switzerland, who was not involved in the study.
The findings highlight not just the stark contrast between static populations and those that are spreading but also the profound role that chance can play in evolution, given the right conditions. “This is about amplifying the importance of chance,” said Kevin Foster, an evolutionary biologist at the University of Oxford, who was not involved in the study. “It means that certain traits, even traits that are not favored by natural selection, can reach very high frequency just by chance.”
Hallstschek’s work “really picked up and sparked a lot of work in understanding the combination of natural selection and population expansion and the genetic signatures that leaves,” said Desai. “Our paper is an outgrowth of that literature. We had been thinking about genetics of expanding populations and realized it had implications for cooperation.”
Tiny Teamwork
Foster and his collaborators first suggested that expansion might provide an additional force driving cooperation using a detailed computational model of microbes in 2010. The model confirmed Hallatschek’s findings and took them one step further, suggesting that range expansion provided the optimal conditions for cooperative behaviors.
This spring, two groups demonstrated this phenomenon in real microbes, highlighting specific conditions that permit altruism to evolve. To study cooperation in yeast, the researchers used two strains — cooperators, which release an enzyme capable of breaking down sucrose into the microbes’ favorite food, glucose, and cheaters, which can’t. Nearly all of the food produced by the cooperators gets released into the environment, meaning that cheaters as well as cooperators can reap the rewards.
In Desai’s experiment, published in Current Biology in May, a drop of liquid housing both yeast strains was deposited on an empty petri dish. As the microbes began to divide and expand into the unoccupied space, the frontier of the population was randomly populated with cheaters and cooperators. This created a founder effect, with highly related groups living on the frontier. “Whatever individuals that managed to migrate early on will have lots of offspring who are related,” said Desai.
As a rule, a population of yeast cooperators will grow faster than a population made up only of cheaters, so the cooperators tend to expand more rapidly into new territory. “They take over at the frontier and eventually the whole frontier population will be cooperators,” says Desai. “Spatial population expansion can dramatically improve the chances that cooperation will successfully evolve.”
Desai’s microbes could expand across two dimensions, but some cases of expansion are one-dimensional, such as birds moving along a linear chain of islands. MIT physicist Jeff Gore and his collaborators analyzed a one-dimensional situation, growing a mix of cheater and cooperator microbes in plates of tiny liquid-filled wells. They manually migrated the microbes, transferring some of the liquid into a new well every day. Unlike Desai’s cheater microbes, which can survive without cooperators, Gore’s cheaters required cooperators for food and survival and invaded the cooperator population as it grew.
Researchers compared how fast the cooperators expanded along the front lines with the speed at which the defectors invaded from behind. For cooperators to succeed, they must expand faster than cheaters can invade. The findings, published in April in the Proceedings of the National Academy of Sciences, show that under harsh environmental conditions, cooperators can spread but cheaters or a mixed population die. However, when both cooperators and defectors are invading an empty space, cooperators can outrun defectors in benign but not harsh environments. (Researchers calculate migration speed by measuring each well’s population density, which grows over time.) “It’s striking the manner in which spatial expansion favors cooperation — they can go to new territory faster than cheaters can invade,” said Gore.
Cooperators have preferential access to the fruits of their labor, because some of the enzyme they secrete gets stuck in their cell walls. That preferential access is particularly important at low cell density because in these conditions there is not very much sugar for any of the cells to eat,” said Gore. “So cooperators can eat some of the public good before it diffuses away.”
Range Expansion in Humans
A growing number of experiments have shown that in microbes, range expansion can have a profound effect on the dynamics of evolution. Human populations have undergone a number of significant expansions, including the migration out of Africa many thousands of years ago. Are the same forces at play?
Expanding populations carry a characteristic genetic signature, and scientists have found this signature in humans. But the challenge is that this signature resembles one left by natural selection. Humans may have migrated out of Africa “for no specific reason, maybe simply because it was possible, but not necessarily because of some selective pressure,” said Laurent Excoffier, a population geneticist at the University of Bern in Switzerland. The findings imply that just because a mutation increases in frequency, it isn’t necessarily under the influence of natural selection. “One set of studies is trying to develop methods to disentangle these purely neutral effects of chance from a real selective sweep,” said Oskar Hallatschek, now a biophysicist at the University of California, Berkeley.
Excoffier and his collaborators tried to examine the effects of range expansion in humans by analyzing the migration patterns of a population of French-Canadians in the 19th and 20th centuries. Because extensive genealogies were kept, the researchers could determine who moved into different territories and when. According to the findings, published in Science in 2011, women on the frontier had 15 percent more children than other women. “People on the wave front left more genes to the current population than those in the core,” said Excoffier. “So there is a similar phenomenon for humans and bacteria — individuals on the wave front have a higher genetic impact on future generations.”
The records showed that the frontier women tended to marry a year earlier, leaving them more time to have children. Though it’s impossible to know exactly why, Excoffier theorizes that earlier marriage arose because there was less competition on the frontier. “They were farmers; they had more resources on the wave front versus the core where the good places were already occupied,” he said, so it would have been easier for young men to provide for wives.
Foster said that spatial expansion is likely to be fundamental to the development of cooperation in microbes. “It’s extremely simple, arguably universal, and explains one of the major findings on microbes,” he said.
Beyond Microbes
Microbes, of course, aren’t the only organisms whose populations expand, or the only cooperative species. In principle, the same factors at play in yeast could also apply to higher organisms, though scientists are careful to say there isn’t clear evidence either way.
“It remains to be seen how widespread this sort of effect is in nature,” said Desai. Many species expand their territories, either seasonally or over longer periods. Did the human migration out of Africa tens of thousands of years ago, for example, favor the development of cooperation?
“I am not aware of any evidence in human populations that range expansion changed the cooperative state in human populations, but both of these studies suggests it can in principle favor cooperative behavior,” said Gore.
Foster is more skeptical about how broadly spatial expansion contributes to cooperation. “It could happen at much greater scale arguably, but I am not yet sure that population expansion promotes cooperation in non-microbe organisms,”he said. Social insects, another group of organisms that display an array of cooperative behaviors, “have a different way of doing things,” he said. “They don’t undergo spatial expansion or genetic segregation as the insect colony grows.”
But understanding microbial cooperation may be important for other reasons, said Joao Xavier, a computational biologist at the Memorial Sloan-Kettering Cancer Center in New York. For example, the dynamics of spatial expansion might have implications for understanding how solid tumors acquire the ability to spread, or metastasize.
In some ways, cancer cells act as cheaters in our otherwise cooperating body. But the most successful cancers also work together. A cell that recruits blood vessels to a tumor “will benefit itself and neighbors,” said Xavier, who began his career as a chemical engineer studying how bacterial colonies can be used to process wastewater. “That’s a potentially cooperative trait.” Xavier, Foster and collaborators have already shown in modeling simulations that the same dynamics that apply to microbes also apply to cancer cells.
Foster said his team is now starting to study more complex communities of microbes. Most laboratory studies focus on one or two strains, but our skin or gut, for example, can harbor hundreds, perhaps thousands, of species, which scientists are discovering play an essential role in human health. “Microbes are not only meeting cheater mutants of their species, they are meeting a whole range of other bugs,” said Foster. “If we want to manipulate or change the microbial community in the gut or in an infection or whatever, we need to understand how they interact in order to understand how they will respond.”
The growing body of work on spatial expansion prompts a darker question as well: What happens when there is nowhere to go? The answer depends on the circumstances. If resources run out, the entire population dies. If resources remain abundant and there is no more room to expand then the defector strain starts to triumph. “When populations stop expanding, those cooperative phenotypes can die out, because the mechanism totally depends on the expansion,” said Desai.
On the other hand, populations rarely stabilize completely. “In natural populations, the idea is that cooperation is maintained because range expansions happen frequently,” said Kirill Korolev, now a physicist at Boston University and Gore’s collaborator. “Maybe there is big disturbance, like a forest fire, and then the population slowly repopulates, over and over.”
This article was reprinted on Wired.com.