Primitive Asgard Cells Show Life on the Brink of Complexity
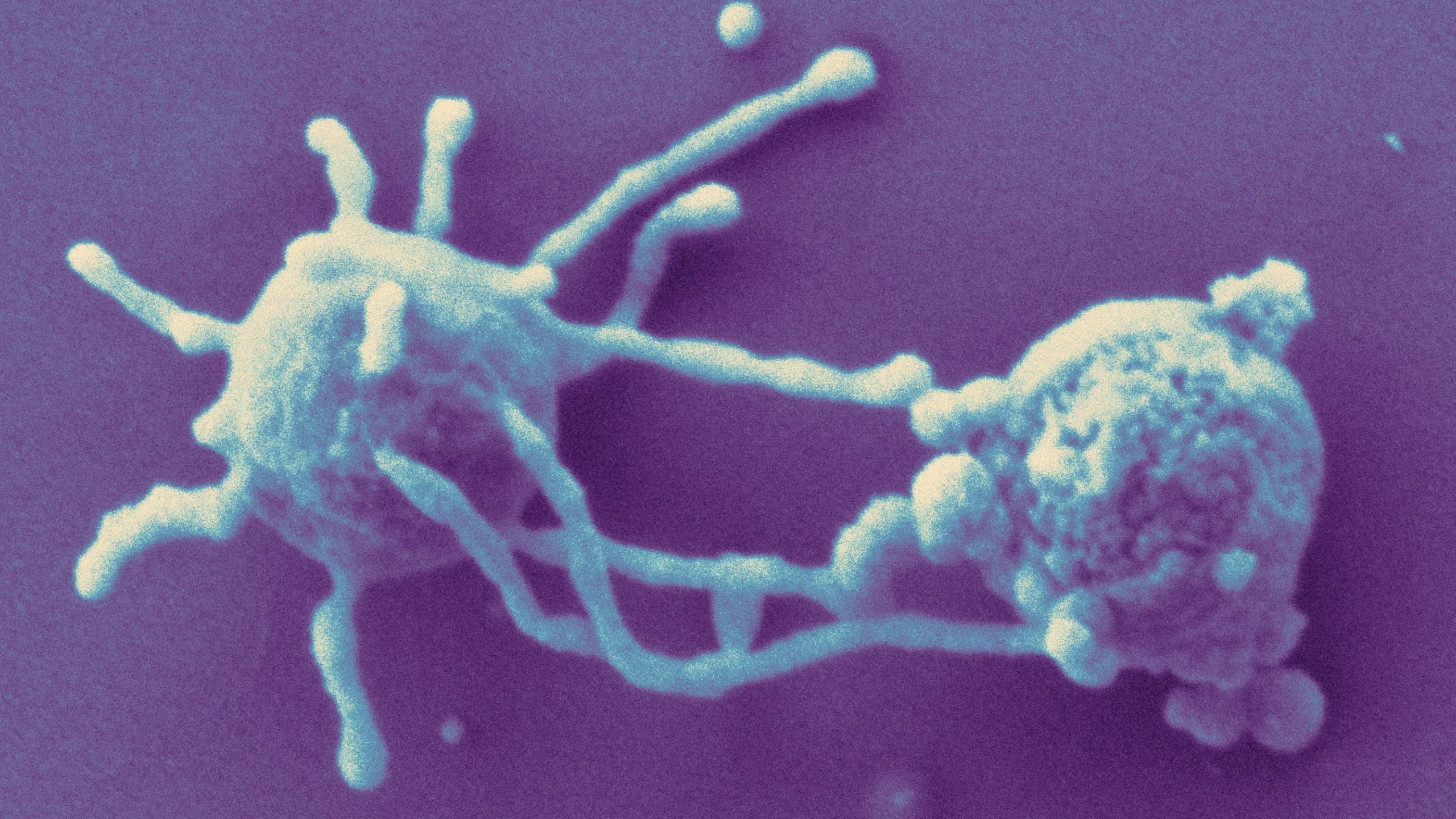
This cell studded with Medusa-like tendrils is tentatively named Lokiarchaeum ossiferum. It’s a newly discovered example of the elusive Asgard archaea, a group of microorganisms that researchers think holds clues to the evolution of complex, eukaryotic life.
Andreas Klingl, Ludwig Maximilian University; modified by Quanta
Introduction
An oak tree. The symbiotic fungus intertwined with its roots. A cardinal chirping from one of its branches. Our best clue yet to their shared ancestor might have arrived in electron microscope images that were unveiled in December.
“Look!” said the microbiologist Christa Schleper, beaming as she held a printed, high-resolution image in front of her webcam at the University of Vienna. “Isn’t it beautiful?” The cells in the micrograph were 500 nanometer-wide orbs surrounded by a Medusa-like halo of tendrils. Her team had not only isolated and cultivated the organism for the first time but shown that its flailing filaments were made of actin, the protein that forms a skeletal scaffold in almost all complex cells, or eukaryotes.
But this was no complex cell. It looked more ancestral, primordial. The organism, first published in Nature, is only the second representative of a group of microbes called Asgard archaea to be grown and studied in detail. Coaxing it to grow out of a tiny spoonful of seafloor sludge, which took six years, was like preparing a dressing room for a temperamental celebrity. The organism couldn’t be centrifuged, stirred, exposed to oxygen, separated from a few other microbes it pals around with, or rushed into growing any faster than a glacial pace.
For months, it didn’t even grow at all. “I worried also for my own future in science,” said Thiago Rodrigues-Oliveira, who led the effort to cultivate the new species as a postdoc in Schleper’s lab, betting his own career on the whims of a single, recalcitrant organism.
As excruciatingly difficult as they are to deal with, the Asgard archaea are now among the most coveted organisms in science, and for good reason. To many evolutionary biologists, their discovery and subsequent studies justify revising the textbook pictures of the tree of life to situate us — and every other creature built from eukaryotic cells — as mere offshoots of the Asgard group.
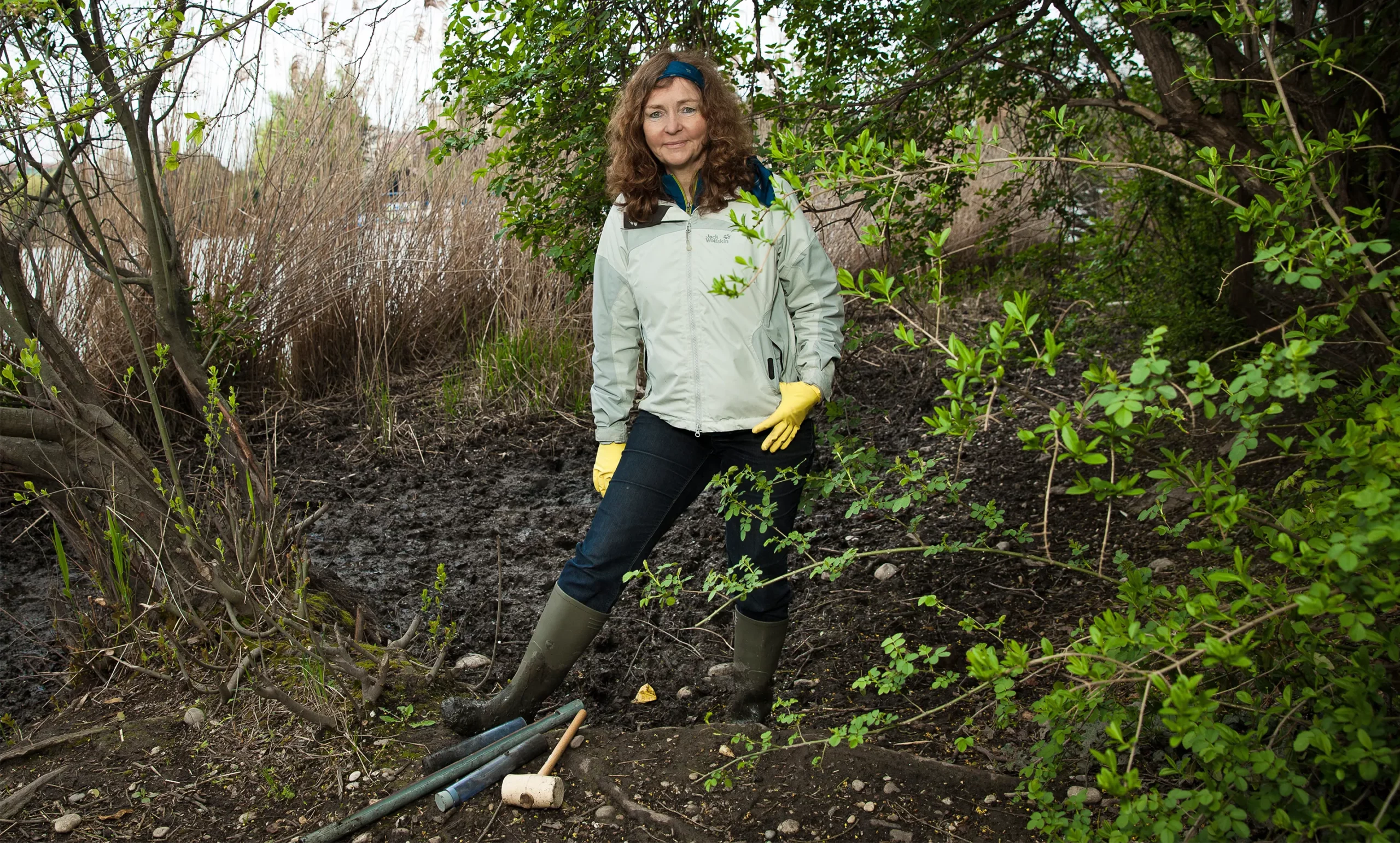
The microbiologist Christa Schleper leads the archaea ecology and evolution group at the University of Vienna. Recently, her laboratory isolated and cultivated a new Asgard archaeon, only the second organism from that group to be studied in detail.
Courtesy of Schleper Lab
Studies of Asgard genomes, meanwhile, have brought badly needed data to the question of how eukaryotes evolved, an epochal event in Earth’s history that inspires contentious debates. Most of the studies to date have had to rely on indirect genetic probes of the Asgard group, which don’t offer the same opportunities as prodding living microbes in a lab, the gold standard in microbiology since the days of Louis Pasteur.
Now a high-stakes, slow-motion race is on as labs around the world attempt to grow their own Asgard cultures. Samples aren’t shared; growth strategies are tightly guarded secrets. “We were honestly shocked” when the Schleper team’s results came out, wrote Hiroyuki Imachi, the microbiologist at the Japan Agency for Marine-Earth Science and Technology who, after a grueling 12-year effort, isolated the first and currently only other Asgard archaea sample.
They aren’t the only ones. Thijs Ettema, an evolutionary microbiologist at Wageningen University in the Netherlands, hinted that his lab had made progress toward enriching Asgard cultures too, and he guessed that at least 10 other labs had similar projects underway. “They wouldn’t be telling me,” he said.
Piecing Together an Organism
The trail that led to Asgard archaea first warmed up a decade ago. That’s when a team including Ettema, Schleper and Anja Spang, who is now an evolutionary microbiologist at the University of Amsterdam, set out to find what they hoped would be an evolutionary missing link.
Biologists had long used genetic data to sort all known organisms into three taxonomic bins: bacteria, archaea and eukaryotes. But they disagreed vociferously on how to draw the family tree that should tie these groups together.
Carl Woese, the influential American microbiologist who discovered archaea in the late 1970s, held that the three groups stood on their own, each alike in dignity, representing distinct “domains” of life. In the view of Woese and his allies, the archaea and the eukaryotes were sister groups descended from an older progenitor. Their opponents argued for a “two-domain” tree of just bacteria and archaea, claiming that eukaryotes had evolved directly from archaea.
Camps formed; positions grew entrenched. “Anything that has to do with our origin, independent of how far you go back in time, is something humans care deeply about,” Spang said.
Years before the new organisms were isolated, microbial surveys picked up hints of an unknown group of archaea with genomes suspiciously close to those of eukaryotes in marine sediments around the world. One study, led by Steffen Jørgensen, Schleper’s doctoral student, showed that these mysterious microbes were thriving in seafloor muck scooped up near a hydrothermal vent in the Atlantic Ocean in 2008. Working with 7.5 grams of mud from these same samples, the team began fishing out longer sequences of stray DNA.
Their intermediate goal was to use a 20-year-old technique called metagenomics to obtain genetic sequences from each organism present. Imagine you have a mixed-up pile of pieces from thousands of puzzles, Spang explained. First you figure out which pieces belong to each puzzle. Then you put each puzzle together. Metagenomics can assemble genomes this way, working only from the DNA of microbes lurking in the mud.
That analysis, published in 2015, unearthed one particularly provocative genome. The organism it belonged to seemed to be the most eukaryote-like archaeon ever discovered, with genes for at least 175 proteins that strongly resembled eukaryotic proteins. The researchers argued that all eukaryotes might have sprung from a close relative of that very archaeon, a view strongly supporting the two-domain version of the tree of life.
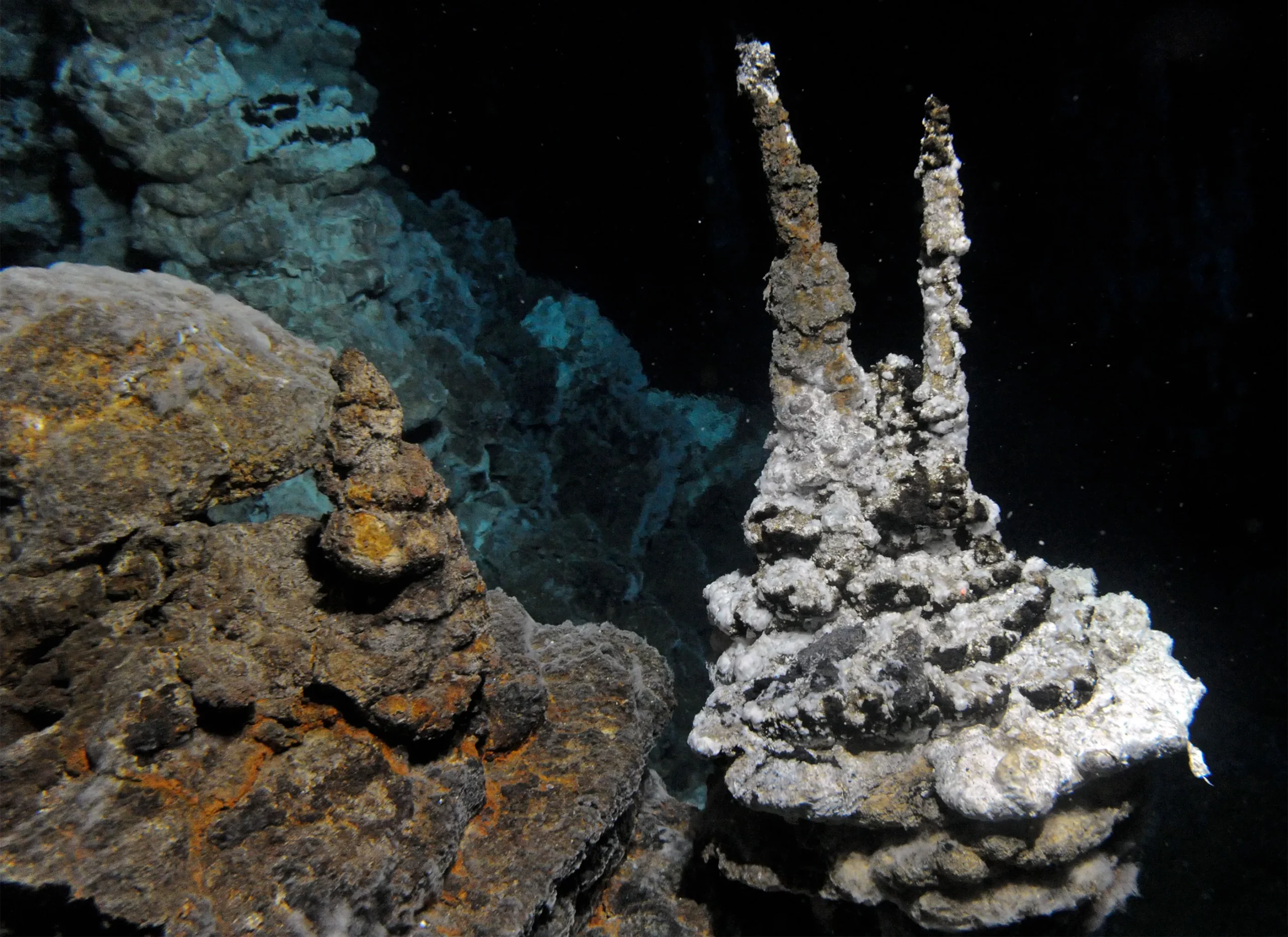
The hydrothermal vent formation called Loki’s Castle, located on the seafloor off the coast of Norway, is near the site where samples yielded the first specimens of what are now called Asgard archaea.
University of Bergen Norway
Ettema named the organism Lokiarcheota. The name was a nod to Loki’s Castle, the hydrothermal vent formation near where the samples had been collected. But the 2015 paper gave an additional reason. “Loki has been described as ‘a staggeringly complex, confusing, and ambivalent figure who has been the catalyst of countless unresolved scholarly controversies,’” they wrote, quoting a scholar of Scandinavian literature. The allusion seemed to fit the contentiousness surrounding eukaryogenesis, the origin of complex cells.
Their discovery soon came under fire from proponents of the three-domain model. Did the Loki organisms really exist? Or had Spang done the metagenomic puzzle-solving wrong and mixed up the genomes of several different microbes into one chimerical, imaginary creature?
But soon Ettema, Spang and many other collaborators uncovered genetic sequences similar to that of the Loki organism in hot springs, aquifers, and both saltwater and freshwater sediments around the world. The organisms weren’t rare at all. They had just been overlooked.
Scientists gave the emerging groups new names that kept to the Norse mythology theme — Odin, Thor, Hel, Heimdall — and referred to the entire realm as the Asgard archaea, after the home of the Norse gods. The additional genomes also seemed to include many eukaryote-like proteins, which further supported the two-domain version of the tree of life in which our eukaryotic branch sprouted from an Asgard ancestor.
Even so, resolving where eukaryogenesis happened in life’s family tree did little to resolve debates around how that process unfolded. Biologists suspected that studying living examples of Asgard archaea might yield more insights than they could glean from looking at fragments of DNA. In 2015, soon after the Asgard group was discovered, Schleper began trying to grow a Loki in Austria.
Unbeknownst to them all, though, one was already multiplying, ever so slowly, in cultivation in Japan.
A Microbe That Plays Hard to Get
“My first name, Hiro, means ‘tolerant,’” Imachi told Quanta in a 2020 interview. “I think [being] tolerant and patient is — how to say it — important in my life.”
In 2006, off the coast of Japan, a crewed submersible called the Shinkai 6500 drilled a core of black, sulfurous sediment out of the floor of a trench under 2.5 kilometers of ocean. Later that year, Imachi put some of this sediment into bioreactors that could simulate a deep-sea environment; he had adapted the equipment from sewage treatment systems for developing countries. Then he settled in to see what this strange garden might grow.
Metagenomics had already revealed that the entirety of known culturable organisms represented just a fraction of nature’s true microbial diversity. Imachi, then a few years out of graduate school, had dedicated his career to the quixotic goal of bringing all microbes into cultivation. To grow something like a Loki for laboratory study, though, would require clearing several daunting hurdles at once.
Merrill Sherman/Quanta Magazine; image courtesy of Florian Wollweber, ETH Zürich (Pilhofer lab)
First, any small piece of seafloor mud hosts hundreds of microbial species. To strip away unwanted bacteria, you can add antibiotics, which are lethal to bacteria but tolerated by archaea. But the antibiotics might also kill symbiotic bacterial species that your target archaeon can’t live without. So it’s necessary to experiment with various antibiotics at different concentrations to find a treatment that’s only appropriately lethal.
Second, you have to find the right mix of nutrients, medium and sediments for your target organism to thrive in. Finally, you have to wait and wait for the target to grow to concentrations high enough to find under an electron microscope or to experiment on. When it’s happy, the organism that Imachi was nurturing divides about once every two or three weeks. By comparison, Escherichia coli, the bacterial workhorse in many microbiology labs, obligingly doubles itself in just 20 minutes.
Five and a half years after their samples went into Imachi’s bioreactor, the Japanese team inoculated whatever was growing inside into little glass tubes. After about a year, they noticed faint signs of life within one tube dosed with antibiotics. Then they started trying to push their target — which they saw had sequences matching those of the Lokiarcheota group Spang had published in 2015 — to higher concentrations.
In the summer of 2019, shortly before uploading their manuscript to a preprint server, Imachi sent Ettema a draft paper announcing their success. Ettema recalled his first glimpse of the creature he had been studying through genetic sequences for years. “It looked like an organism from a different planet,” he said. “I’ve never seen something like that.”
The Japanese group’s electron microscope images ended the debate over whether the Loki organism was real or an artifact of metagenomics. But their work also established two crucial new discoveries about the Loki archaea: that the organism surrounded itself with tiny arms, and that it seemed to thrive in codependent clumps with a sulfate-reducing bacterium and another species of archaeon that produced methane.
Meanwhile, in Schleper’s lab in Austria, the initial six-year grant was dwindling and no new funding was in sight. One postdoc assigned to the task of growing the organism had ended up leaving science. Another team member, a technician, had pipetted so much they needed surgery for carpal tunnel syndrome.
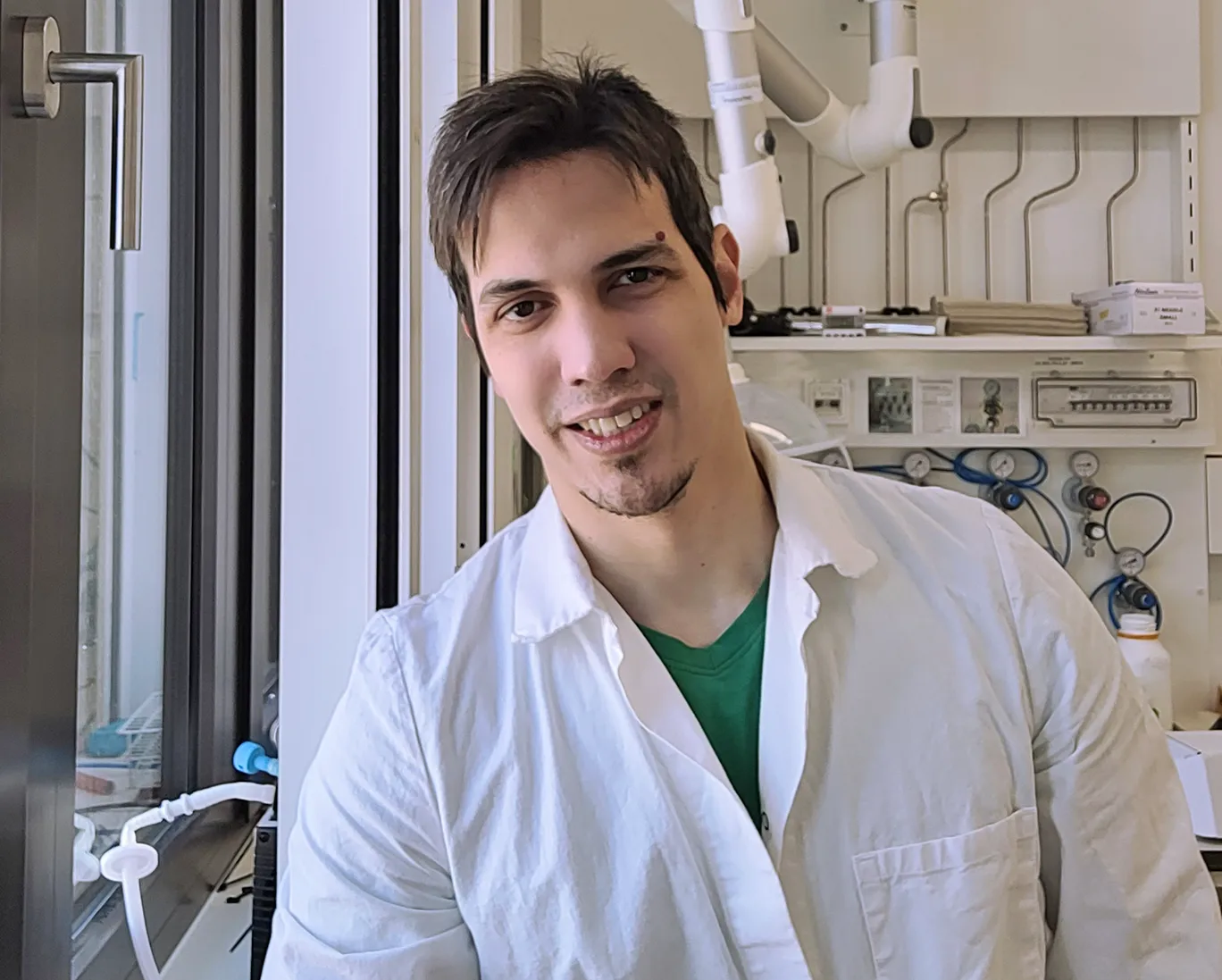
Thiago Rodrigues-Oliveira, a postdoc in Schleper’s lab in Vienna, led the effort to cultivate the new species of Asgard archaea, the second from the Loki group.
Karin Hager
In the fall of 2019, however, a culture of a Loki organism started by Rodrigues-Oliveira began to inch along. It divided in about half the time as the Japanese strain, and it reached densities 50 to 100 times higher. Even so, working with it could still be like leafing through a Where’s Waldo? book: In 36 hours of scanning samples through an electron microscope, Schleper said, the team spotted just 17 individual specimens.
Last December, they debuted their results in Nature. This Loki, too, had tentacle-like filaments that Schleper’s group speculate might entangle other organisms and interact with them. Scooping the Japanese team, they showed that the tentacles were made of a protein, Lokiactin, that closely resembles the actin with which eukaryotic cells build supportive cytoskeletons. So not only is the Lokiactin gene like a eukaryotic gene, but it performs a eukaryote-like function.
The Lokiactin gene also pops up in every one of the 172 or so Asgard genomes that scientists have encountered. That implies that the ancestor of the entire group — and maybe the ancestor of all eukaryotes — might have had a similar proto-skeleton.
So what is Schleper’s lab trying to do with the organism now? “Everything!” she said, laughing.
Reaching Out to Form Complex Cells
Within the now-dominant two-domain picture to which the Asgard archaea are contributing, the big story of life on this planet goes something like this. Some 4 billion years ago, life forked into two single-celled branches, the archaea and the bacteria.
Genetic evidence implies that the two branches crossed again 2 billion years later when an archaeon — likely from the Asgard group — somehow ingested a bacterium. The process domesticated what was once a distinct, free-living cell and turned it into the organelles called mitochondria that persist inside eukaryotic cells. The descendants of that fateful union branched into other single-celled organisms like dinoflagellates, and then later into multicellular creatures that grew to macroscopic sizes, left fossils behind, and colonized both sea and land.
But even theorists who stand behind this narrative belong to divided camps. Some argue that gaining mitochondria was the defining event in eukaryogenesis. Others insist that mitochondria arrived late in an ongoing transition.
“You might have had Asgard archaea that were already quite complex and quite eukaryote-like,” said Tom Williams, a computational microbiologist at the University of Bristol, describing the latter position. “Then they acquired mitochondria, in an extreme form of this view, as a sort of icing on the cake.” Williams, however, thinks that mitochondria were acquired earlier than that: The complexity of the Asgards has just tipped the discussion toward an intermediate view, he said.
But the data from research on Asgards has also constrained the eukaryogenesis debate in other ways. For one thing, both of the Asgards cultivated so far have proved hard to separate from an entourage of other microbes. Like the Japanese Loki, the Austrian organisms seems to prefer — even depend on — having an extra species of archaeon and another sulfate-reducing bacterium in culture with them. Scholars working on eukaryogenesis, such as Purificación López-García at the French National Center for Scientific Research, have long promoted the idea that mitochondria were first captured from within just this kind of “syntropic” partnership, where multiple species live interdependently.
The finding that Lokis have actin tentacles adds plausibility to a eukaryogenesis scenario called the inside-out model, Spang and Schleper said. In 2014, the cell biologist Buzz Baum at University College London and his cousin, the evolutionary biologist David Baum of the University of Wisconsin, Madison, proposed an idea they had kicked around at family events: that the first eukaryotes were born after a simple ancestral cell extended protrusions past its cell walls. First these arms reached toward a symbiotic bacterium. Eventually they closed around that partner, turning it into a proto-mitochondrion. Both the original archaeal cell and the captured symbiote were enveloped within a skeleton provided by the arms.
Back when Asgard archaea were still known only from scraps of environmental DNA, Baum had asked attendees at a conference to draw what they thought the organisms would look like. His own drawing based on the inside-out ideas, which predicted that they would sport protruding arms, surprised the other assembled scientists. At the time, Schleper said, it seemed “so odd that he makes this funny suggestion.”
A Competitive Atmosphere
The events of eukaryogenesis have been so obscured by intervening time and gene-swapping that we may never know them with certainty.
The two Loki species currently in culture, for example, are modern-day organisms that differ from ancient archaea in the same way that a living, singing cardinal differs from the ancestral dinosaur from which it evolved. The Loki group isn’t even the subset of Asgard archaea that genetic analyses suggest is most closely related to eukaryotes. (Based on known Asgard genomes, a preprint posted by Ettema and his colleagues in March argued that the ancestor of eukaryotes was a Heimdall archaeon.)
Still, labs around the world are gambling that bringing more diverse representatives of the Asgard group into cultivation will yield a bonanza of new clues about their — and our — common ancestor. Schleper is trying. So is Ettema. So is Baum, who said his lab is soon welcoming a new colleague who will bring vials of archaea from groups like Heimdall and Odin. So is Imachi, who declined to speak to Quanta for this story.
“If I were to be interviewed by you now, I would most likely talk about new data that has not yet been published,” he explained in an email, adding that his group applauded the Schleper team’s efforts. “It is very competitive now (although I do not like this kind of competition),” he added.
Other sources also bemoaned the overly pressurized atmosphere. “It would be nice if the field would be more open to sharing,” Spang said. The pressure weighs heaviest on the young scientists who tend to take on the high-risk, high-reward cultivation projects. Success can add a glowing Nature paper to their resume. But wasting years on a failed effort can stunt their chances of ever getting a job in science. “It’s really an unfair situation,” Schleper said.
For now, though, the race continues. When the Baum cousins published their ideas about eukaryogenesis in 2014, Buzz Baum said, they assumed we’d probably never know the truth. Then suddenly the Asgards showed up, offering new glimpses of the liminal, transitional stages that boosted life from single-celled simplicity into overdrive.
“Before we destroy this beautiful planet, we should do a bit of looking, because there’s cool things on planet Earth we know nothing about. Maybe there are things that are sort of living fossils — states in between,” he said. “Maybe it’s on my shower curtain.”
Correction added April 11, 2023:
In an earlier version of this story, when Tom Williams described a “mitochondria late” view of eukaryogenesis, it was not clear that he was describing a view with which he disagrees.