Room-Temperature Superconductivity Claim Falls Apart [Update]
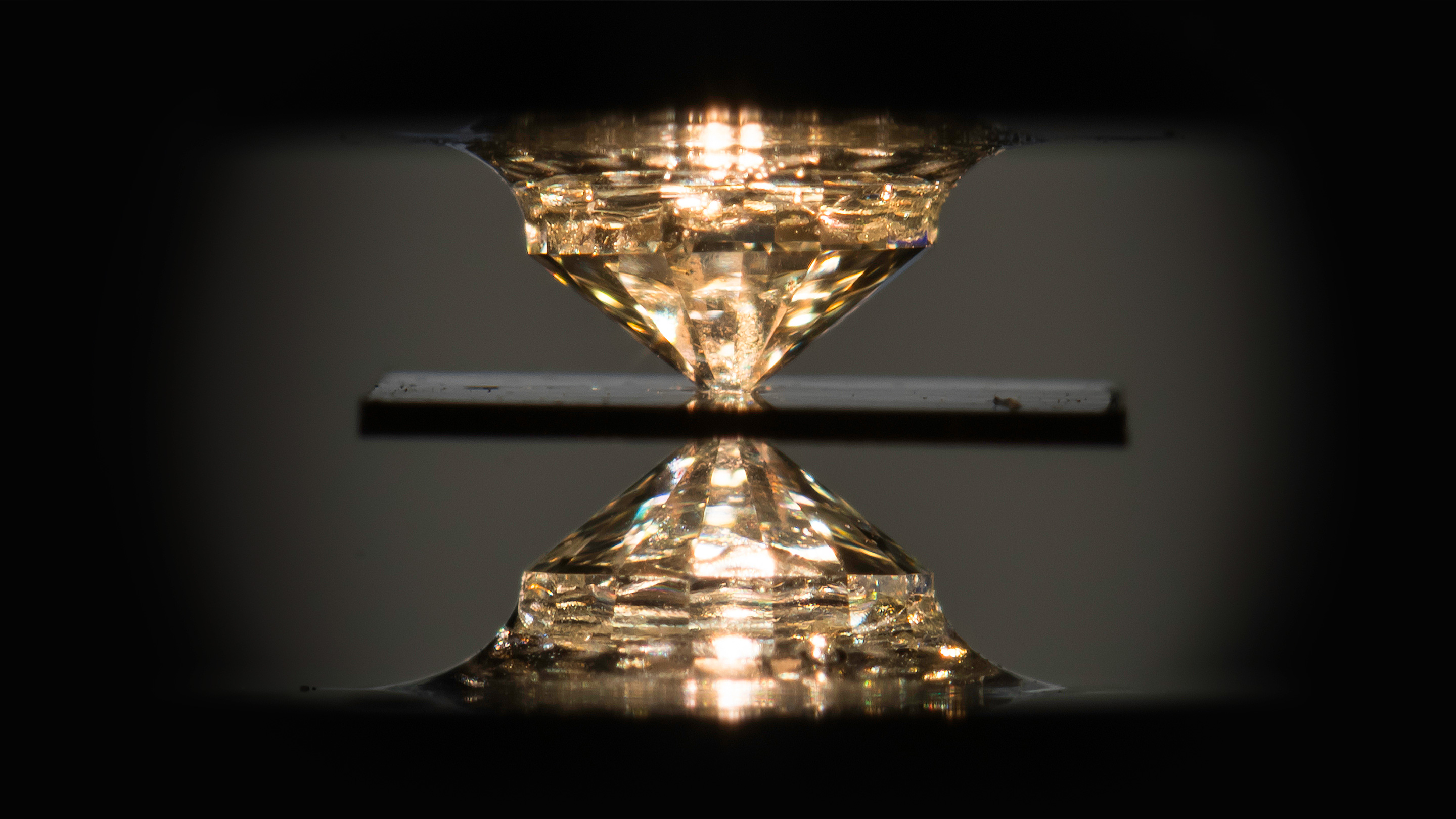
A novel metallic compound of hydrogen, carbon and sulfur was claimed to exhibit superconductivity at a balmy 59 degrees Fahrenheit when pressurized between a pair of diamond anvils.
J. Adam Fenster / University of Rochester
Introduction
Editor’s Note: On September 26, 2022, the journal Nature retracted the paper by Ranga Dias and coauthors claiming room-temperature superconductivity in a pressurized carbonaceous sulfur hydride (CSH) material.
Doubts began to swirl soon after the publication of the paper. Outside researchers became puzzled by aspects of the data handling — in particular, the non-standard procedure Dias and colleagues used to subtract a background signal from their raw data in order to infer their CSH sample’s “magnetic susceptibility,” a property that indicates whether it is superconducting.
Dias’ group reportedly plans to resubmit their paper to Nature without the questionable subtraction method, claiming that the magnetic susceptibility signal is apparent even in their raw data. But independent researchers have told Quanta that they doubt the result will hold up, both because of unusual features in the raw data itself that Dias and coworkers have not explained, and in light of failed attempts by other groups to replicate the results.
Mikhail Eremets, a physicist at the Max Planck Institute for Chemistry who reported the first observation of superconductivity in a hydride material in 2015, told Quanta that he spent half a year trying to replicate Dias’ results. “We failed,” he said. When his group synthesized CSH compounds and subjected them to high pressure, they did observe superconductivity in some samples, but the effect only survived at temperatures up to –73 degrees Celsius, far colder than room temperature.
Alexander Goncharov at the Carnegie Institution for Science and coworkers also attempted to synthesize the CSH compound in question; they could not create any CSH compound that undergoes a structural change (as revealed by spectroscopy measurements) at the pressure where Dias says superconductivity sets in.
“We were not able to synthesize the material with the reported [spectroscopic signature] according to their recipe,” Goncharov told Quanta.
Eremets emphasized that the most direct evidence of room-temperature superconductivity in Dias and colleagues’ CHS compound — raw measurements of the sample’s electrical conductivity — has never been released. Like the processed magnetic susceptibility signal in their paper, the processed conductivity signal is “also controversial,” Eremets said, with strange and unexplained features.
Dias and coauthors stand behind their work and do not agree with the retraction.
This article’s original headline, “Room-Temperature Superconductivity Achieved for the First Time,” has been updated. Our original article from October 2020 appears below.
A team of physicists in New York has discovered a material that conducts electricity with perfect efficiency at room temperature — a long-sought scientific milestone. The hydrogen, carbon and sulfur compound operates as a superconductor at up to 59 degrees Fahrenheit, the team reported today in Nature. That’s more than 50 degrees hotter than the previous high-temperature superconductivity record set last year.
“This is the first time we can really claim that room-temperature superconductivity has been found,” said Ion Errea, a condensed matter theorist at the University of the Basque Country in Spain who was not involved in the work.
“It’s clearly a landmark,” said Chris Pickard, a materials scientist at the University of Cambridge. “That’s a chilly room, maybe a British Victorian cottage,” he said of the 59-degree temperature.
Yet while researchers celebrate the achievement, they stress that the newfound compound — created by a team led by Ranga Dias of the University of Rochester — will never find its way into lossless power lines, frictionless high-speed trains, or any of the revolutionary technologies that could become ubiquitous if the fragile quantum effect underlying superconductivity could be maintained in truly ambient conditions. That’s because the substance superconducts at room temperature only while being crushed between a pair of diamonds to pressures roughly 75% as extreme as those found in the Earth’s core.
“People have talked about room-temperature superconductivity forever,” Pickard said. “They may not have quite appreciated that when we did it, we were going to do it at such high pressures.”
Materials scientists now face the challenge of discovering a superconductor that operates not only at normal temperatures but under everyday pressures, too. Certain features of the new compound raise hopes that the right blend of atoms could someday be found.
Electrical resistance occurs in normal wires when freely flowing electrons bump into the atoms that make up the metal. But researchers discovered in 1911 that at low temperatures, electrons can induce vibrations in a metal’s atomic lattice, and those vibrations in turn draw electrons together into couples known as Cooper pairs. Different quantum rules govern these couples, which stream together in a coherent swarm that passes through the metal’s lattice unimpeded, experiencing no resistance whatsoever. The superconducting fluid also expels magnetic fields — an effect that could allow magnetically levitating vehicles to float frictionlessly above superconducting rails.
As the temperature of a superconductor rises, however, particles jiggle around randomly, breaking up the electrons’ delicate dance.
Researchers have spent decades searching for a superconductor whose Cooper pairs tango tightly enough to withstand the heat of everyday environments. In 1968, Neil Ashcroft, a solid-state physicist at Cornell University, proposed that a lattice of hydrogen atoms would do the trick. Hydrogen’s diminutive size lets electrons get closer to the nodes of the lattice, augmenting their interactions with the vibrations. Hydrogen’s lightness also allows those guiding ripples to vibrate faster, further strengthening the glue that binds the Cooper pairs.
Impractically high pressures are needed to squash hydrogen into a metallic lattice. Still, Ashcroft’s work raised hopes that some “hydride”— a mixture of hydrogen and a second element — might deliver metallic hydrogen’s superconductivity at more accessible pressures.
Progress took off in the 2000s, when supercomputer simulations let theorists predict the properties of various hydrides, and the widespread use of compact diamond anvils let experimentalists squeeze the most promising candidates to test their mettle.
Suddenly, hydrides started setting records. A team in Germany showed in 2015 that a metallic form of hydrogen sulfide — a pungent compound found in rotten eggs — superconducts at −94 degrees Fahrenheit under 1.5 million times the pressure of the atmosphere. Four years later, the same lab used lanthanum hydride to hit −10 degrees under 1.8 million atmospheres, even as another group found evidence for superconductivity in the same compound at 8 degrees.
Dias’ lab in Rochester has now shattered those records. Guided by intuition and rough calculations, the team tested a range of hydrogen compounds searching for the goldilocks ratio of hydrogen. Add too little hydrogen, and a compound won’t superconduct as robustly as metallic hydrogen does. Add too much, and the sample will act too much like metallic hydrogen, metalizing only at pressures that will crack your diamond anvil. Over the course of their research, the team busted many dozens of $3,000 diamond pairs. “That’s the biggest problem with our research, the diamond budget,” Dias said.
The winning recipe proved to be a riff on the 2015 formula. The researchers started with hydrogen sulfide, added methane (a compound of carbon and hydrogen), and baked the concoction with a laser.
“We were able to enrich the system and introduce just the right critical amount of hydrogen necessary to maintain these Cooper pairs at very high temperatures,” said Ashkan Salamat, Dias’ collaborator and a condensed matter physicist at the University of Nevada, Las Vegas.
But the fine details of the hydrogen-carbon-sulfur potion they’ve cooked up elude them. Hydrogen is too small to show up in traditional probes of lattice structure, so the group doesn’t know how the atoms are arranged, or even the substance’s exact chemical formula.
Eva Zurek, a computational chemist at the University at Buffalo, belongs to a group of theorists loosely affiliated with Dias’ lab. Earlier this year they predicted the conditions under which one metal that might have formed between the diamond anvils should superconduct, and they found different behavior. She suspects that high pressures instead transformed Dias’ substance into an unknown form whose superconductivity is especially robust.
Once Dias’ group can figure out exactly what they’ve got on their hands (details he and Salamat say are coming soon), theorists will build models exploring the features that give this hydrogen-carbon-sulfur mixture its superconducting power, in hopes of further modifying the recipe.
Physicists have proved most two-element hydrogen hybrids to be dead ends, but the new three-element blend marks a potentially significant advance into the world of complex chimera materials. One of the elements involved seems particularly promising to some.
“What I like about this work: They bring carbon into the system,” said Mikhail Eremets, an experimentalist at the Max Planck Institute for Chemistry in Germany whose lab set the hydride records of 2015 and 2019.
He explained that the lightness of hydrogen is not the only way to beef up the vibrations that steer electrons into Cooper pairs. Stronger links between neighboring atoms in the lattice also help, and, he said, “carbon has very strong covalent bonds.” Materials with carbon frames could bring the added benefit of preventing the whole assembly from tumbling down at the low pressures humans find comfortable.
Zurek agrees. “Room pressure I thought would be very challenging,” she said. “But if we can bring carbon compounds into the mix, I think that presents a way forward.”
This article was reprinted on Wired.com.
Correction October 15, 2020: Ashkan Salamat is an assistant professor at the University of Nevada, Las Vegas, rather than simply the University of Nevada, as originally stated.