Researchers Revise Recipe for Building a Rocky Planet Like Earth
Introduction
Bob O’Dell wasn’t quite sure what he was looking at. It was 1992, and he had just got his hands on new images from the Hubble Space Telescope that zoomed in on young stars in the Orion Nebula. O’Dell had been hoping to study the nebula itself, an interesting region of star formation relatively close to Earth. Yet something else caught his attention. Several of the stars didn’t look like stars at all, but were instead enveloped by a dim shroud. They seemed to form a “silhouette against the nebula,” said O’Dell.
At first O’Dell and his colleagues thought they might be seeing an image artifact resulting from Hubble’s warped primary mirror, which had been molded ever so slightly into the wrong shape and would be fixed by a space shuttle mission in 1993. “We really wondered if this was a residual effect of the flawed primary mirror,” said O’Dell, who had been Hubble’s project scientist. Soon, however, they saw more and more of the phenomena in the images, even after the mirror was fixed, and realized it wasn’t a flaw at all. They were actually seeing infant disks of dust and gas surrounding young stars. They were, for the first time, witnessing the birth of planets.
O’Dell’s discovery of protoplanetary disks sparked a transformation in our understanding of planet formation. In the following decades, astronomers would realize that our classical idea of how planets form — small rocks clump into bigger rocks, which then clump further — might not be correct. For the gas giants, such as Jupiter and Saturn, a model called pebble accretion, where a dominant object gobbles up smaller rocks — would come to replace the old views of how such monstrous worlds come to be.
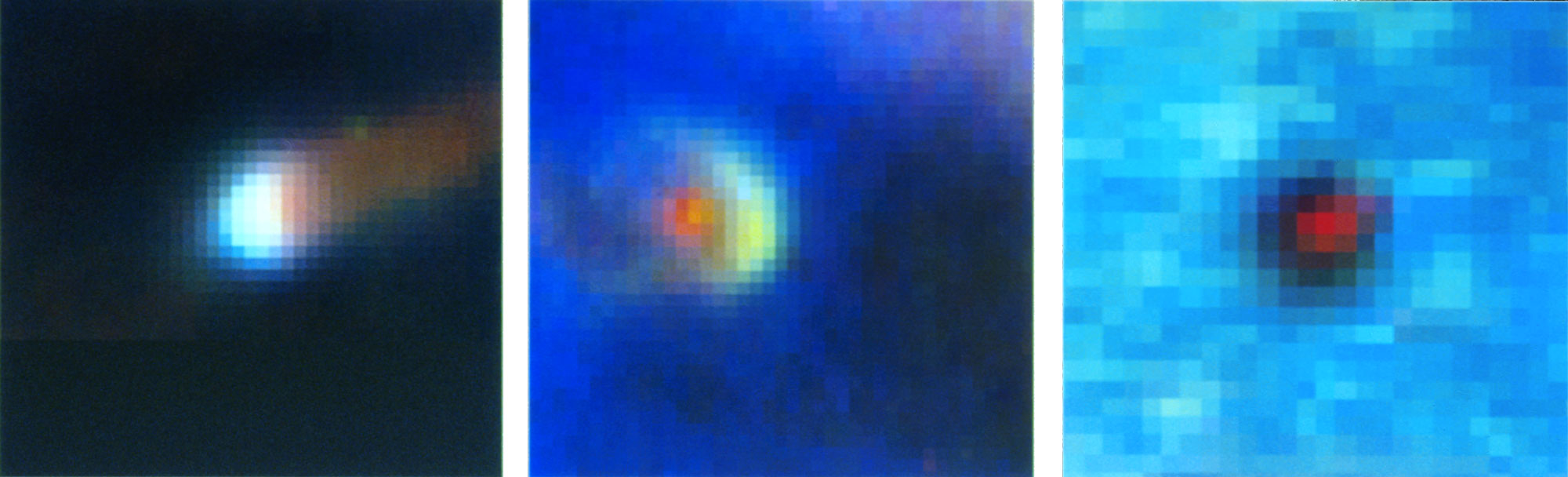
These Hubble Space Telescope images provided the first direct evidence of protoplanetary disks around distant stars.
C.R. O’Dell (Rice University), and NASA
The rocky worlds of the inner solar system are trickier. Planetary scientists have intensely debated whether pebble accretion can explain how Earth and its neighbors arose, or whether the older view is still most likely. The clash has played out over the past few years in journal articles and even, more recently, in a castle in the Bavarian Alps.
The debate doesn’t only affect great mysteries such as the origin of Earth — and its water. The answer will also help reveal just how prevalent Earth-like worlds are across the universe. Are such worlds a cosmic fluke, merely a combination of chance events that make the prospects of life elsewhere in the universe slim? Or are habitable planets a certainty in solar systems with just the right ingredients, making us but one of many?
“It’s part of the human experience to ask how the world around us formed,” said Konstantin Batygin, a planetary scientist at the California Institute of Technology. If it formed from pebbles, it will have huge consequences for how many more worlds like ours are out there.
Invasion of the Pebbles
In 2012, Anders Johansen and Michiel Lambrechts, astronomers at Lund University in Sweden, made a bold prediction. For much of the preceding few decades, astronomers had believed that planets such as Earth and Jupiter grew from the gradual accumulation of asteroid-like objects, planetesimals, that collided with each other in young solar systems. This process, known as planetesimal accretion, would be slow — perhaps taking up to 100 million years to form a planet. But it made sense. We could see lots of asteroids in our solar system, and it seemed reasonable to assume there were many more when it formed 4.5 billion years ago, enough to form all the worlds we see today.
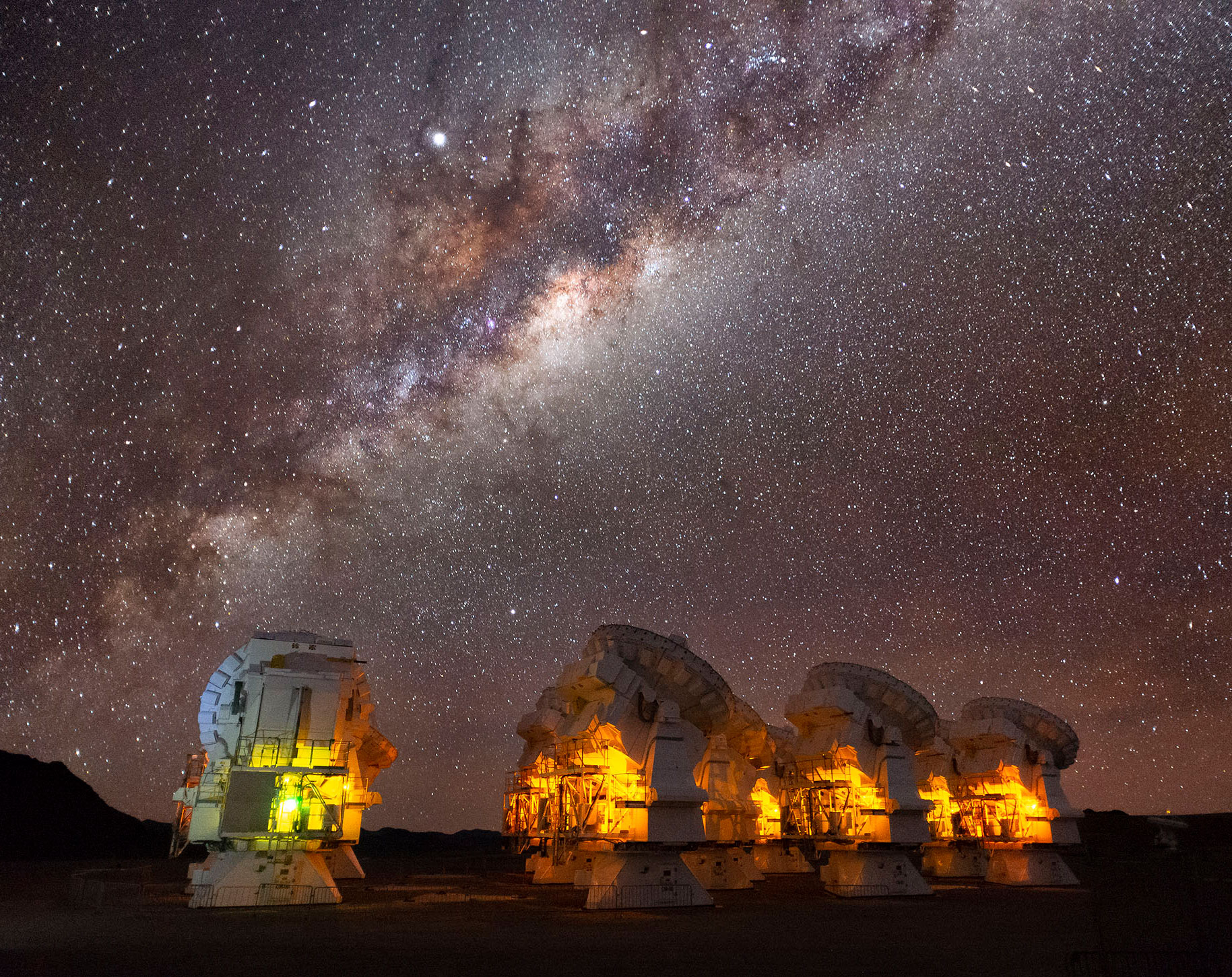
The ALMA telescope array can create exceptionally high resolution images, allowing researchers to examine planet-forming disks around other stars.
Sergio Otarola (ESO/NAOJ/NRAO)
But there were problems. No one was quite sure how planetesimals themselves formed — how they made the jump from tiny dust grains to city-size rocks, a problem known as the meter-size barrier. The presence of liquid water on Earth was confusing, as it relied on the chance arrival of water-bearing asteroids. And most troubling, planetesimal accretion would take far too long to build Saturn, Uranus and Neptune. By the time their solid cores formed — after tens of millions of years — it would be too late for them to accumulate enough gas from the protoplanetary disk to become gas giants, as “most disks go away in a few million years,” said André Izidoro, a planetary scientist at Rice University.
Johansen and Lambrechts proposed a new model. Instead of multiple planetesimals colliding together, they instead suggested that a single dominant planetesimal could grow to a huge size in a short amount of time — just a few million years — by sweeping up material inside a protoplanetary disk “like a vacuum cleaner,” said Johansen. This material would consist of tiny seedlike rocks that surrounded young stars. They called the idea pebble accretion.
Pebbles are extremely small, just a few millimeters to centimeters in size, whereas planetesimals are much larger, up to hundreds of kilometers wide, like many of the asteroids we see in the solar system today. Both would be found in a star’s protoplanetary disk, with the latter occasionally smashing into one another.
In 2014, just two years after Johansen and Lambrechts published their pebble model, observations revealed that disks were indeed full of pebbles. A network of 66 telescopes called ALMA (the Atacama Large Millimeter/submillimeter Array) revealed up to 100 Earth masses’ worth of pebbles inside a protoplanetary disk surrounding a young star, including wide gaps created by growing planets carving out their orbits. Inside these disks, pebbles were everywhere. ALMA “showed that protoplanetary disks are born with enormous mass reservoirs of small pebbles, not planetesimals,” said Lambrechts.
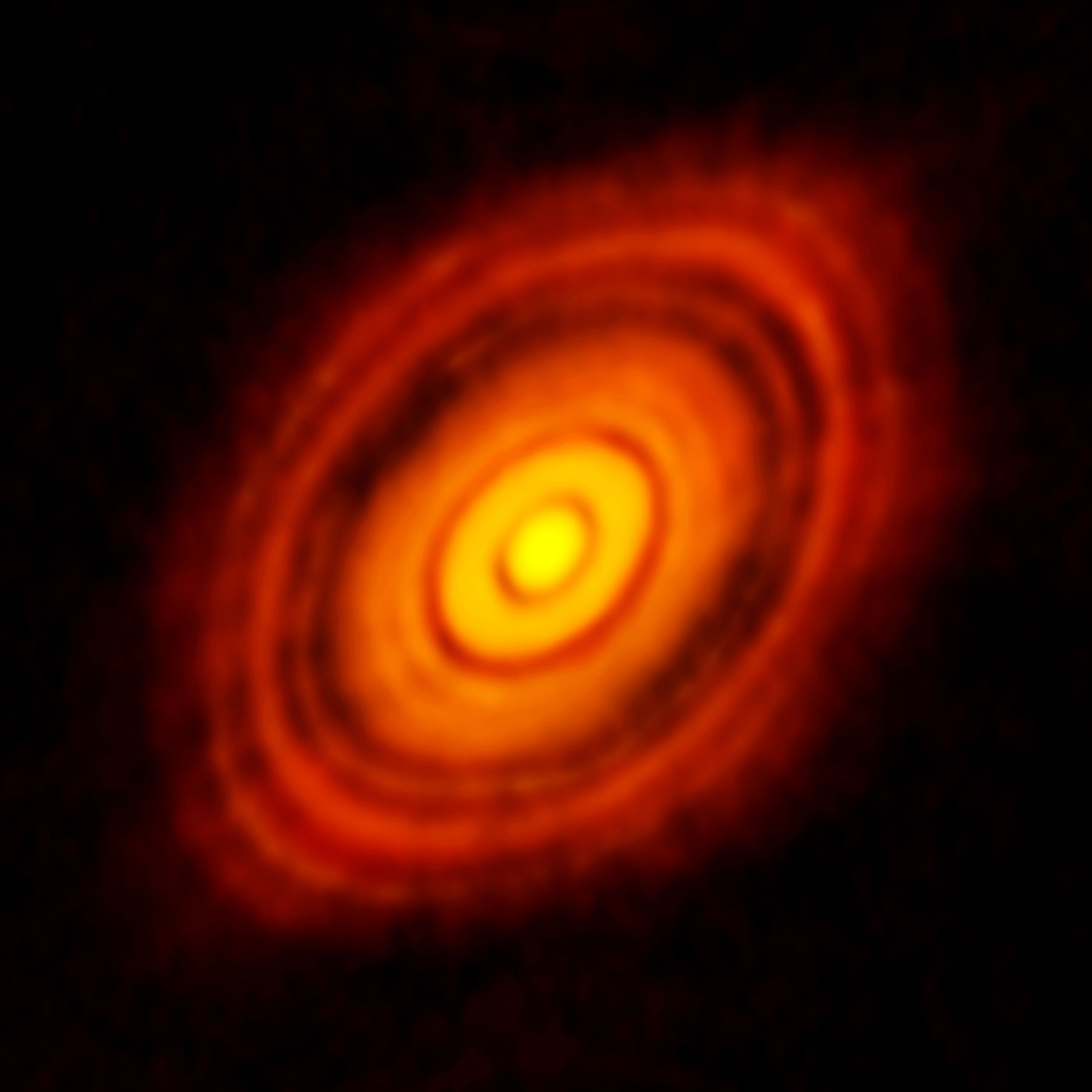
ALMA observations of protoplanetary disk around HL Tauri in 2014 revealed hidden structures, including the presence of pebbles in the disk.
ALMA (ESO/NAOJ/NRAO)
Before long, most scientists came to agree that pebble accretion formed the giant planets. It just seemed to be the only way for them to grow fast enough. “For the cores of the giant planets there is no doubt pebble accretion is the solution,” said Alessandro Morbidelli, a planetary scientist at the Côte d’Azur Observatory in France.
Yet, while it seemingly explained the formation of Jupiter, Saturn, Uranus and Neptune, pebble accretion raised considerable questions about the formation of the terrestrial planets: Mercury, Venus, Earth and Mars. “In principle one could form the terrestrial planets with planetesimal accretion,” said Lambrechts. “But now there’s this invasion of the pebbles.”
In the pebble accretion model, you begin with a protoplanetary disk around a young star, just like in the planetesimal accretion model. Both models then require planetesimals to form via a phenomenon called streaming instability. Essentially, dust and pebbles experience drag as they encounter the gas surrounding the star. This causes the pebbles to clump together, until some clumps “are so massive that they become gravitationally bound, and they collapse into planetesimals” up to hundreds of kilometers wide, said Joanna Drążkowska, an astrophysicist at the Ludwig Maximilian University of Munich. The clumps may then rotate as they form, which gives them two lobes. “This is exactly what we see” in outer solar system objects such as Arrokoth, said Drążkowska. The process is expected to be incredibly quick, perhaps taking only 100 years.
From here, the two models diverge. Under planetesimal accretion, these planetesimals form everywhere in the disk, leaving few pebbles left. Over tens of millions of years, the large planetesimals collide and merge, eventually giving rise to the terrestrial planets we see today.
In pebble accretion, just a few planetesimals become dominant. These planetesimals begin to sweep up pebbles in the protoplanetary disk, which stream down onto the surface of the planetesimal in long riverlike filaments. It is an extremely energetic process, with hot magma oceans glowing on the surface as pebbles rain down. “These planets would shine,” said Lambrechts. The process is very efficient; Earth would grow to its full size in just a few million years, compared to perhaps 100 million years in planetesimal accretion.
One of the most interesting outcomes of pebble accretion is that it gives a direct prediction of how habitable planets form. Rather than relying on water-rich asteroids to haphazardly collide with protoplanets, the model suggests that incoming icy pebbles from the outer solar system could provide a steady supply of water to a planet like Earth, an idea known as pebble snow. “The nice thing about pebble snow is that it becomes predictable,” said Johansen. “The amount of water and carbon and nitrogen that comes down to Earth is something that can be calculated.”
Thus, if the pebble accretion model for terrestrial planet formation is correct, it may bode well for the prospects of other life in the universe. Whereas under planetesimal accretion the existence of water on Earth was a chance event, in pebble accretion it might be expected in a planetary system like our own. Take a proto-Earth and put it around a similar star in a similar position, and the amount of water it collects could be the same. Habitable worlds would not be chance events; their existence would be a calculable outcome if a planetary system has the right ingredients. “One can use this as a starting point for understanding prebiotic chemistry and the origin of life,” says Johansen.
The Great Architect
Pebble accretion seems like an attractive idea. It solves the problem of rapid planet growth, it explains the presence of water on Earth, and we can even observe pebbles in developing exoplanetary systems. “With ALMA we know now pebbles are concentrated in particular regions that lead to planetesimal formation and potentially planets,” said Paola Pinilla, a planetary formation scientist at University College London.
Yet, while it provides a good explanation of giant planet growth, pebble accretion has some notable issues when it comes to terrestrial planets.
First, where did the pebbles in the inner solar system come from? In recent years, planetary scientists have come to believe that Jupiter, the largest planet in our solar system, was the primary force shaping the destiny of the planets. “The emergent picture is that Jupiter was the great architect of the solar system,” said Batygin.
Soon after Jupiter’s rapid formation, it created a barrier between the inner and outer solar system, preventing material from the “mass-rich” outer regions from flowing to the “mass-starved” inner terrestrial planets, said Batygin. “The giant planets blocked the flux of dust and pebbles,” said Morbidelli. Pebbles in the inner disk may have dissipated before the terrestrial planets could form, and without more material coming in from the outer solar system, there simply would not have been enough material to make Earth.
Even if there was enough material, pebble accretion runs into another problem: It is extremely efficient, but perhaps too much so. If Earth and the other terrestrial planets did form by pebble accretion, it is not clear why they did not grow larger and larger, eventually becoming super-Earths — worlds somewhere between Earth and Neptune in size, which seem to be relatively common in other planetary systems. “The difficulty with pebble accretion is it’s either not very efficient or it’s very efficient,” said Sean Raymond, an astronomer at the Astrophysics Laboratory of Bordeaux in France. “It’s rarely in between. And to work for the terrestrial planets, you need to have just the right amount of stuff.” Too little material and planets simply never grow. Too much and the planets grow too large too quickly “and the solar system would have super-Earths rather than terrestrial planets,” said Raymond.
These issues have caused considerable debate among planetary scientists in the last few years, with much ongoing research from both sides of the argument. In September, Morbidelli and his colleagues published an article in Nature Astronomy based on studies of the protoplanet Vesta that suggested how planetesimals would explain the current configuration of the solar system. The study suggests that a ring of planetesimals once orbited the sun at the current location of Earth. In time, this ring formed two large planets — Earth and Venus — toward the middle of the ring, with two smaller worlds — Mars and Mercury — on the flanks.
Others, however, continue to investigate ways in which pebbles may birth terrestrial planets. In February, Johansen and colleagues described how our own solar system could have formed in this way. Then last month, Drążkowska and colleagues used pebble accretion to explain why super-Earths are relatively uncommon around other sunlike stars.
At a workshop at Ringberg Castle in Germany last month, the debate flowed freely. Some, like Johansen and Lambrechts, remain very much in favor of a pebble accretion model for terrestrial planets. “There’s very strong evidence that this is the dominant process,” said Johansen. Others are less convinced. “I think pebble accretion is a very important process to understand planet formation, but I don’t think it’s the process that built the terrestrial planets in our solar system,” said Thorsten Kleine, a planetary scientist at the University of Münster in Germany. The two processes could also have worked in concert, with pebble accretion creating planetesimals that then merged after Jupiter cut off the flow of incoming pebbles.
Some hope that turning to cosmochemistry, the study of the compositions of cosmic objects, might reveal the answer. Johansen pointed out that if the planetesimal accretion model were correct, we would expect to find asteroids of a similar composition to Earth, given that they were likely the building blocks of our world. Yet this is not the case. “I think that’s a limitation of the classical model, because they haven’t been able to find one,” he said. “There are really no meteorites that look like Earth at all.”
If Earth formed via pebble accretion, however, we might have expected to see a “much higher abundance” of volatile elements like nitrogen and carbon on Earth, delivered by pebbles coming from the outer solar system, said Conel Alexander, a cosmochemist at the Carnegie Institution for Science in Washington, D.C. “We just don’t see that,” he said. Scientists like Alexander hope that combining new ideas of cosmochemistry with modeling of the early solar system could provide some useful answers. “Both the modelers and the cosmochemists have a bit of work to do,” he said.
Elsewhere, continued studies of exoplanetary systems could reveal more information. Already more than 5,000 protoplanetary disks have been observed by ALMA, said Pinilla, from very young disks of less than 1 million years to disks up to 30 million years in age. On one occasion, we have even seen a giant planet, a world called PDS 70b, being born inside such a disk, with more sightings hoped for in the future. Some disks show the glow of dust, indicating the possible presence of colliding planetesimals — although how many isn’t clear. Upcoming observations from the James Webb Space Telescope (JWST), set to launch in December, alongside work from ALMA, could provide invaluable additional clues. “If we bring chemical traces from JWST, and the pebble distribution from ALMA, we can have some hints of what types of planets can form in the inner parts of the disks,” said Pinilla.
Much remains uncertain. The major question now is: Was our planet the result of repeated collisions between huge asteroid-like bodies, or are we standing on top of a world made of trillions upon trillions of tiny, perhaps ice-rich cosmic pebbles? Solving that fundamental question will provide a window not only into our own past, but into Earth-like worlds everywhere.