Scientists Re-Create the Microbial Dance That Sparked Complex Life
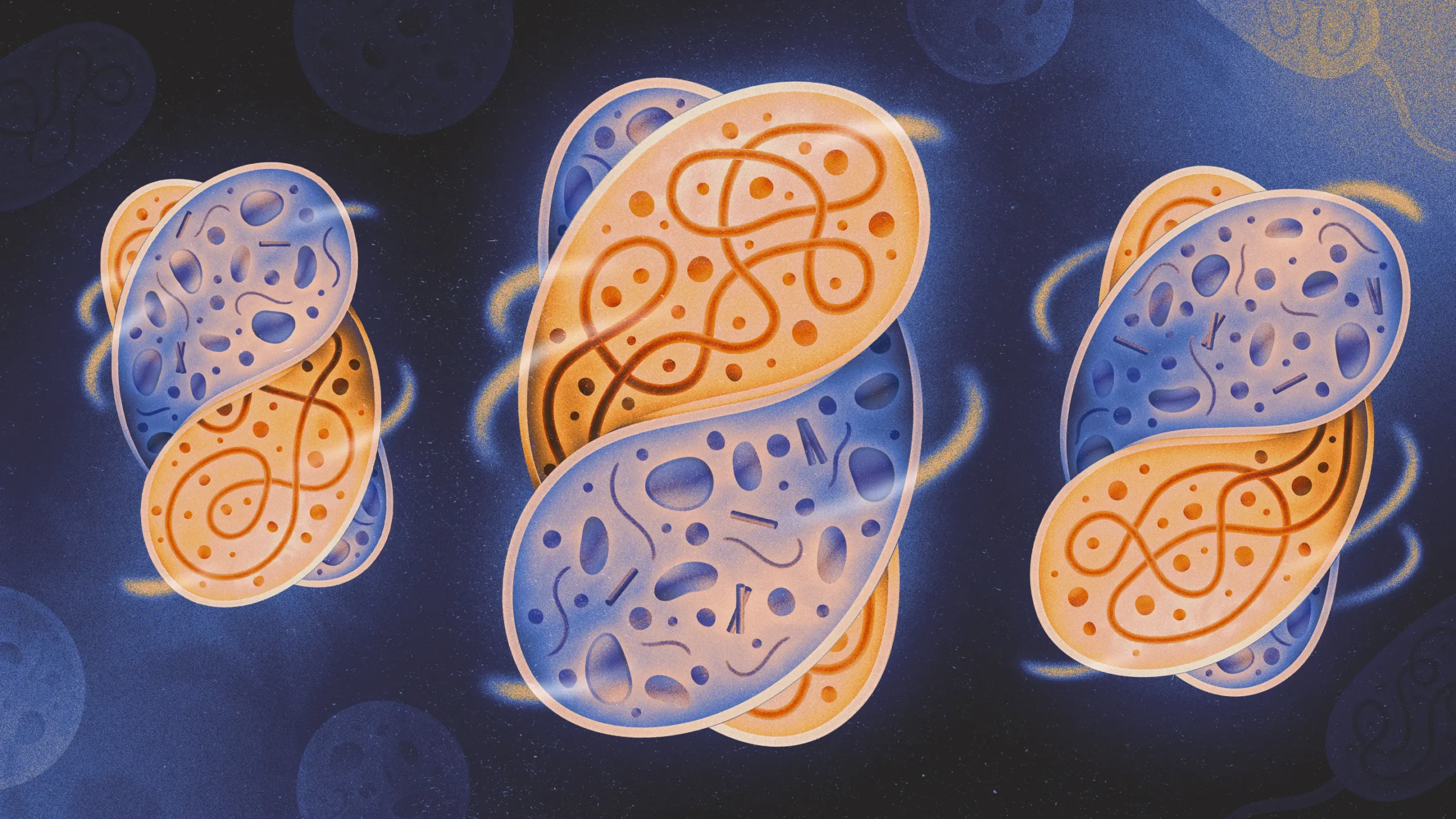
Endosymbiotic relationships between two microbes, with one living inside the other, underlie cellular complexity across the tree of life. Until now, no one has observed the opening choreography of such a partnership’s formation.
Kristina Armitage/Quanta Magazine
Introduction
Far from being solo operators, most single-celled microbes are in complex relationships. In the ocean, the soil and your gut, they might battle and eat each other, exchange DNA, compete for nutrients, or feed on one another’s by-products. Sometimes they get even more intimate: One cell might slip inside another and make itself comfortable. If the conditions are just right, it might stay and be welcomed, sparking a relationship that could last for generations — or billions of years. This phenomenon of one cell living inside another, called endosymbiosis, has fueled the evolution of complex life.
Examples of endosymbiosis are everywhere. Mitochondria, the energy factories in your cells, were once free-living bacteria. Photosynthetic plants owe their sun-spun sugars to the chloroplast, which was also originally an independent organism. Many insects get essential nutrients from bacteria that live inside them. And last year researchers discovered the “nitroplast,” an endosymbiont that helps some algae process nitrogen.
So much of life relies on endosymbiotic relationships, but scientists have struggled to understand how they happen. How does an internalized cell evade digestion? How does it learn to reproduce inside its host? What makes a random merger of two independent organisms into a stable, lasting partnership?
Now, for the first time, researchers have watched the opening choreography of this microscopic dance by inducing endosymbiosis in the lab. After injecting bacteria into a fungus — a process that required creative problem-solving (and a bicycle pump) — the researchers managed to spark cooperation without killing the bacteria or the host. Their observations offer a glimpse into the conditions that make it possible for the same thing to happen in the microbial wild.
The cells even adjusted to each other faster than anticipated. “To me, this means that organisms want to actually live together, and symbiosis is the norm,” said Vasilis Kokkoris, a mycologist who studies the cell biology of symbiosis at VU University in Amsterdam and wasn’t involved in the new study. “So that’s big, big news for me and for this world.”
Early attempts that fell short reveal that most cellular love affairs are unsuccessful. But by understanding how, why and when organisms accept endosymbionts, researchers can better understand key moments in evolution, and also potentially develop synthetic cells engineered with superpowered endosymbionts.
The Cell Wall Breakthrough
Julia Vorholt, a microbiologist at the Swiss Federal Institute of Technology Zurich in Switzerland, has long puzzled over the circumstances of endosymbiosis. Researchers in the field theorized that once a bacterium sneaks into a host cell, the relationship teeters between infection and harmony. If the bacterium reproduces too quickly, it risks depleting the host’s resources and triggering an immune response, resulting in the death of the guest, the host or both. If it reproduces too slowly, it won’t establish itself in the cell. Only in rare cases, they thought, does the bacterium achieve a Goldilocks reproductive rate. Then, to become a true endosymbiont, it must infiltrate its host’s reproductive cycle to hitch a ride to the next generation. Finally, the host’s genome must eventually mutate to accommodate the bacterium — allowing the two to evolve as a unit.
“They become addicted to each other,” Vorholt said.
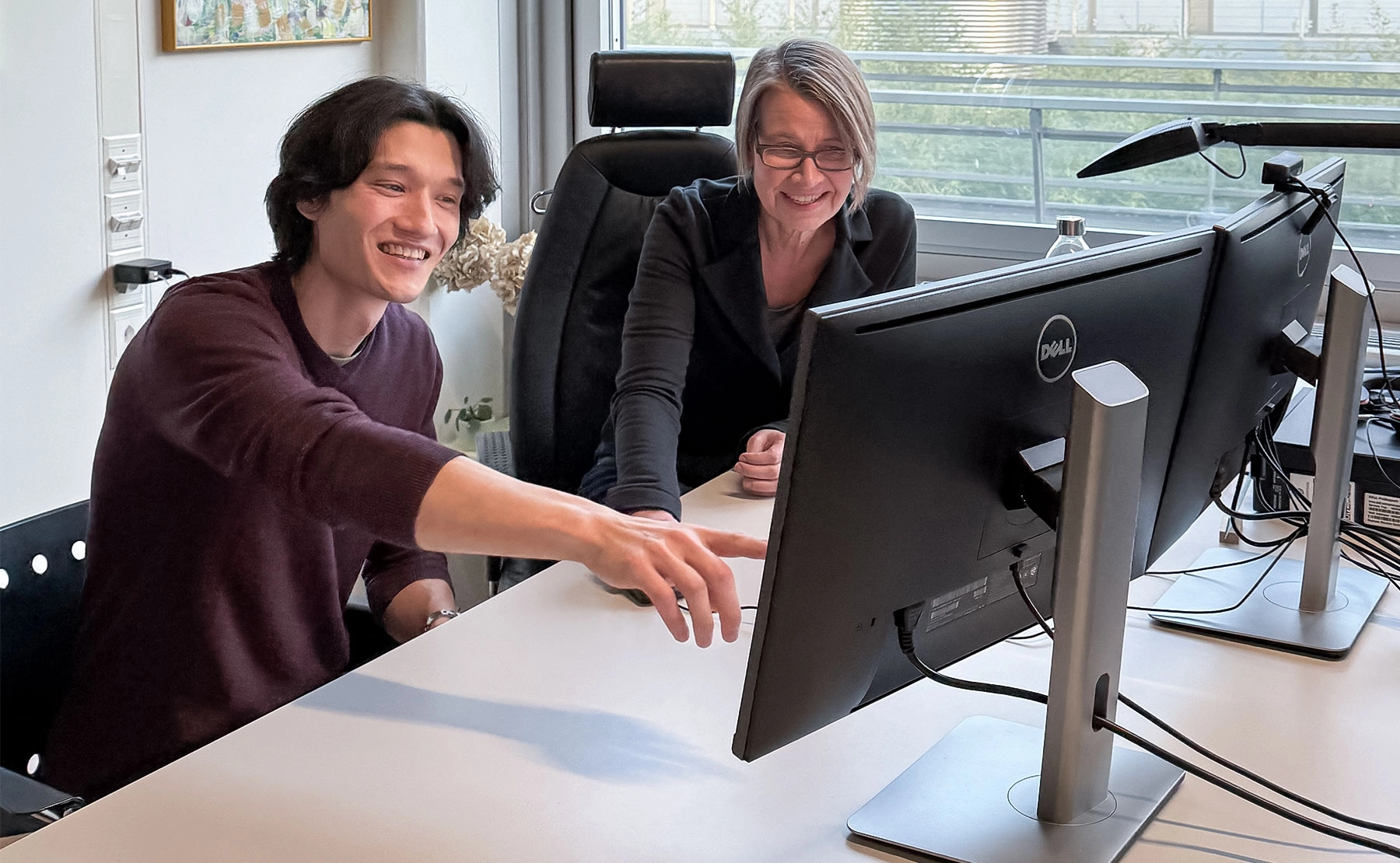
To observe the initial steps taken by two microbes as they adjusted to endosymbiotic life, biologists Gabriel Giger and Julia Vorholt re-created a wild endosymbiotic relationship in their lab at the Swiss Federal Institute of Technology Zurich.
Thomas Gassler
These ideas made logical sense, but no one had ever witnessed the early steps of microbial endosymbiosis. So Vorholt decided to try to make it happen in the lab. Rather than reinventing the endosymbiotic wheel, she thought her team would have its best shot if it re-created a partnership that had already occurred in nature.
Rice seedling blight is a disease caused by the toxic by-product of a wild, endosymbiotic affair. At some point in its evolutionary history, the fungus Rhizopus microsporus adopted the bacterium Mycetohabitans rhizoxinica. The bacterial resident produces poison, which the fungus uses to infect rice plants; both partners benefit by absorbing nutrients from the dead and dying plant cells. Over generations, the pair have become so intertwined that now the fungus can’t reproduce without its endosymbiont.
However, there is a strain of the fungus that lives without the endosymbiont. Vorholt thought she could use it to re-create the poisonous partnership. Before she got to the harder steps of cellular matchmaking, though, her team had to overcome a basic physical constraint: How do you physically squeeze a bacterium through a fungus’s rigid cell wall?
Gabriel Giger, lead author on the paper and Vorholt’s graduate student, started by cooking up a cocktail of enzymes to soften the wall. Then he used an atomic force microscope equipped with a technology known as FluidFM, repurposed to serve as a tiny syringe. When Giger punctured the fungal cell with the microneedle, cytoplasm came rushing out like water from a burst dam.
“We had so much backflush,” Giger said. “[The cell fluid] just comes shooting right at you.”
He needed something with more oomph to resist the intracellular pressure and push the bacteria in. Giger jury-rigged a connection between his bike pump and the microscope. It worked: The bike pump boosted the pressure and forced the bacteria through the cell wall and into the cytoplasm.
After tinkering with different amounts of pressure, they refined the system. “The way they adapted technology to inject the bacteria into fungus is really, really cool,” said Thomas Richards, an evolutionary biologist who studies endosymbiosis at the University of Oxford and wasn’t involved in the study. “They had to use special sharpened needles and then three times the tire pressure of car tires to push that bacteria inside. That represents a big technological step forward.”
Giger and Vorholt first injected the fungus with Escherichia coli, a standard bacterial lab organism. Once inside, E. coli reproduced quickly as it fed on nutrients within the cell. The bacteria grew so fast that the fungal immune system noticed them — and handily locked them away for disposal.
Then the researchers moved on to M. rhizoxinica, the bacteria already established within other R. microsporus strains. Once inside, it divided at an agreeable rate and evaded the immune response. Most importantly, neither partner died. “It was already super exciting to see that both the fungus and the bacteria grew after injection,” Giger said.
The pair had initially accepted each other, but that was only the first step. Giger patiently waited, and then saw what he was looking for under the microscope: The bacteria had wiggled their way into the fungal spores to hitchhike to the next generation.
“I had to make sure the signal was the real deal, and you don’t sleep soundly until you know,” he said. “The excitement lasted for quite a while.”
Giger and the team hand-selected spores and germinated 10 successive generations of fungi. More bacteria survived in each reproductive round, and the spores got healthier and more efficient. For the first time, researchers watched endosymbiotic and host microbes adapt to each other. “Neither of these organisms is poisoning each other, and their growth rates roughly match this spectrum of viability for both,” Giger recalled. The bacteria survived, protected and fed by the fungus — and the fungus scored a poisonous partner.
To confirm the microbial partnership, the lab isolated both parties to analyze their genomes. Already, the fungus genome had gained mutations to accommodate the bacteria. Clearly, these relationships can stabilize quickly, the researchers saw. Soon the two species couldn’t live without each other.
Striking the Right Balance
By re-creating a natural relationship, Vorholt and Giger have “rerun that tape of evolution,” Richards said, to learn lessons about how endosymbiosis happens. They concluded that the process can’t happen if there is a mismatch between host and endosymbiont at any point in the adaptation process. “That’s probably what happens in nature a lot,” Vorholt said. “Maybe their starting points are successful, but somehow the selection is not there, or there is a cost rather than a benefit. And then you just lose the system, and it doesn’t get stabilized.”
They also learned that in pairings that work, both partners adapt to each other — a phenomenon that has been largely overlooked. It wasn’t just the bacteria adapting to a new environment; the host changed too, even in the early stages. “That is a fundamentally important question that people have ignored,” Richards said. “This opens the doors for real advances.”
While illuminating, this bacterium-fungus pairing is only one example of a process that may have a number of mechanisms or conditions. “I can imagine that in protists and other groups that have not been well studied, we will find many new patterns of how symbiosis is supported,” said Laila Partida Martínez, who discovered the rice seedling–blight endosymbiosis and is now director of Cinvestav Irapuato, a plant science research institute in Mexico.
More research in a variety of endosymbiotic systems will reveal which conditions apply generally and which are specific to certain pairs. Further down the line, those findings could lead to a new kind of synthetic biology, featuring lab-grown endosymbiotic relationships, which could be a “fascinating avenue to explore biological innovation,” Vorholt said.
Instead of editing organisms’ genes to create new traits, labs could engineer bacteria to perform specific functions and then slip them into hosts. “Many new features could be brought together in a symbiotic system by doing this and making them evolve together,” Partida Martínez said. By inducing endosymbiosis, researchers could potentially engineer plants to metabolize pollutants or manufacture medicines. “It will take time to design and to really tune the systems,” she added. “I think our imagination would be actually the limit.”
Does that mean we could one day gain chloroplasts and become photosynthetic? Giger thinks it would be difficult for a chloroplast to stabilize inside a mammalian cell. Even if it did work, photosynthesis alone wouldn’t fuel us — our energy demands are too high. “You might get fancy green skin and run a little bit on your own photovoltaics, but the energy gain that you could get from the sun would be minimal,” he said. “You’d go hungry a lot and need to supplement with other staples, such as pizza.”