Scientists Unravel How the Tonga Volcano Caused Worldwide Tsunamis
Introduction
In August 1883, a mountainous island in Indonesia named Krakatau, or Krakatoa, self-destructed. Episodic volcanic eruptions crescendoed in an explosion that sent debris 80 kilometers high and smothered 800,000 square kilometers of Earth’s surface in corrosive ash. As much of the island blasted apart and splashed into the sea, a tsunami rose up and slammed into the nearby islands of Java and Sumatra, causing most of the eruption’s 36,000 fatalities.
While Indonesia bore the brunt of the damage, the Krakatau eruption had perplexing effects across the world. Somehow, small tsunamis lapped at the shorelines of countries in both the Pacific and Atlantic oceans, even though there didn’t appear to be any way Krakatau’s tsunami could have leapfrogged from the Indian Ocean over continents and into other ocean basins. Lacking any other explanation, scientists at the time blamed these far-flung tsunamis on coincidentally timed earthquakes.
But geophysicists in subsequent decades kept puzzling over the data. A study from 1955, for example, found that the distant tsunamis correlated with the arrival of the pressure wave that propagated outward through the air from the eruption. The study’s authors speculated that some sort of coupling had taken place between this atmospheric disturbance and the water. Computer simulations in 2003 further supported this, showing that Krakatau’s main tsunami, even where it did make its way through gaps in the continents to reach both the Pacific and Atlantic oceans, lagged behind tiny tsunamis in places like Hawai‘i, California and Alaska, which instead synced up with the faster pressure wave from the blast. (A pressure wave whose frequency is in hearing range is known as sound.)
To corroborate the speculative idea that volcanoes’ sound or pressure waves could cause tsunamis, scientists needed to see another version of Krakatau play out in real time in the modern era — an awkward wish, to be sure.
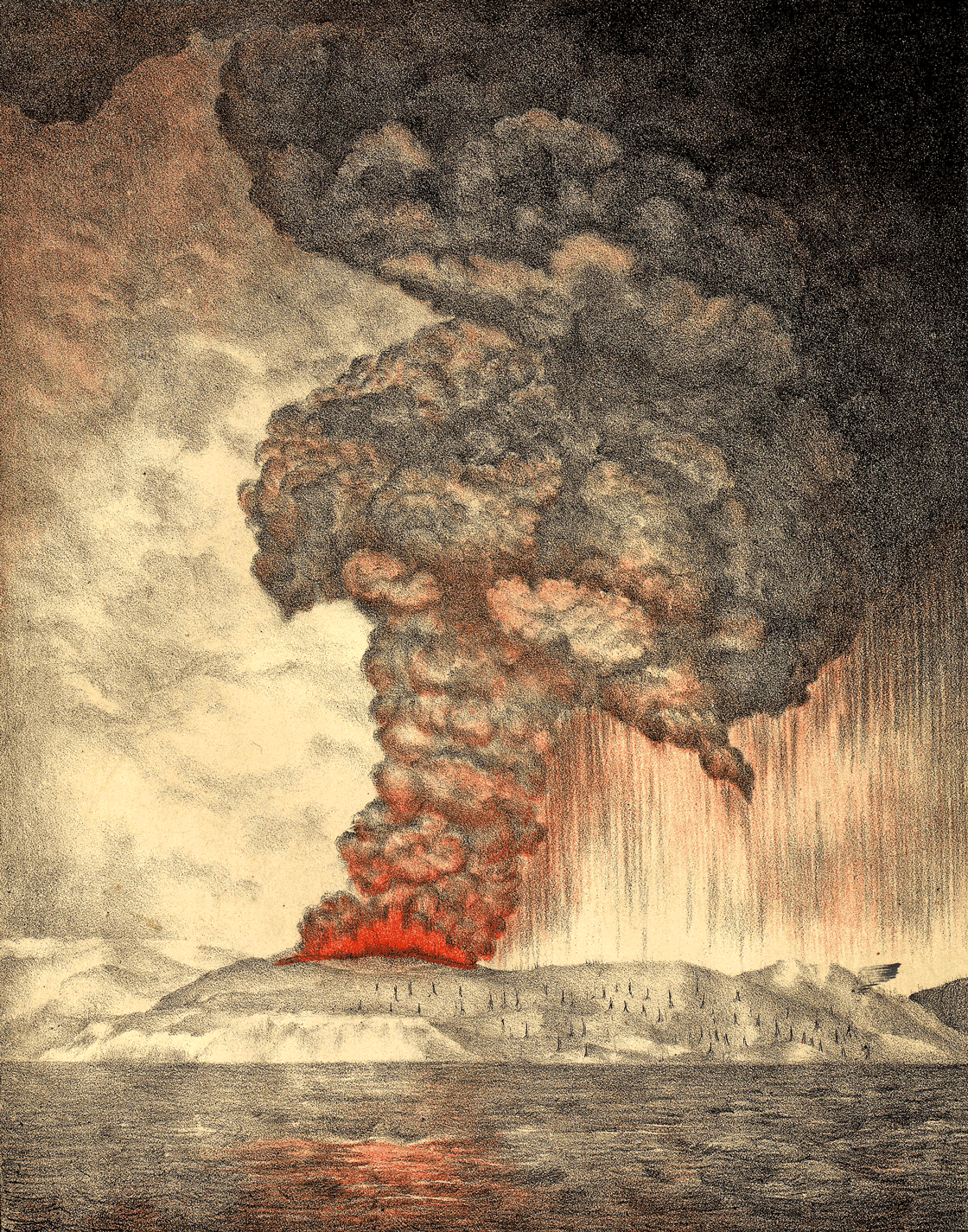
An 1888 lithograph of the 1883 eruption of Krakatoa.
Parker and Coward
Then, on January 15, 2022, a mostly submerged volcanic cauldron in the South Pacific named Hunga Tonga-Hunga Ha‘apai unleashed a nightmarish roar. Its mushroom cloud of ash and its local tsunami devastated the archipelagic Kingdom of Tonga. Although it caused mercifully few fatalities, this volcano broke all sorts of records: It blasted debris two-thirds of the way to space; its ash cloud generated up to 200,000 lightning discharges per hour; and the explosion itself was one of the most powerful ever recorded.
In terms of the scale and energy of the explosion, the Tongan event was “basically like Krakatoa 2,” said Matthew Haney, a geophysicist at the U.S. Geological Survey’s Alaska Volcano Observatory. A gunshot-like crack could be heard in Anchorage, where Haney is based. “We’re 6,000 miles away. You could hear a volcanic eruption? Wow. That just blows my mind.”
It wasn’t just Hunga Tonga-Hunga Ha‘apai’s sound that made its way around the world. A spate of tsunamis, tens of centimeters tall, splashed onto distant shores in disparate ocean basins. “We weren’t expecting that tsunami signal in the Caribbean,” said Paul Fanelli, an oceanographer at the National Oceanic and Atmospheric Administration (NOAA).
This time, scientists think they’ve found the solution. When wave height gauges across the planet were matched with corresponding air pressure sensors, it became clear that the pressure wave from the explosion must have connected with the surfaces of multiple oceans and seas, transferred energy to the water, and created myriad tsunamis.
This explanation simultaneously solved a 139-year-old mystery about Krakatau. “Given what was observed in 1883 … it makes sense that that would have happened then too,” said Greg Dusek, a physical oceanographer at NOAA. “Both sets of observations seem to agree well.”
But as with all sweeping scientific revelations, new questions have arisen. When and why do volcanoes’ pressure waves tango with ocean waves? Why did Tonga’s far-flung tsunamis only appear along certain coastlines? And just how potent, and potentially destructive, could these tsunamis get?
Tsunami School
Normally, to make a tsunami, you need to push a lot of mass into a body of water to displace it. Earthquakes do this in a straightforward way. “Earthquake tsunamis are really, really simple,” said Emily Lane, a hydrodynamicist at New Zealand’s National Institute of Water and Atmospheric Research. “There’s an earthquake that happens under the water, that actually causes the seafloor to be deformed, and that deformation travels to the surface of the water, and then it radiates out as a tsunami.”
Volcanic tsunamis are more complicated. Debris ejected into or splashing down into the water, the partial or complete collapse of the volcano itself, and underwater explosions can all displace water. Investigative work on the seafloor around Hunga Tonga-Hunga Ha‘apai will find out in the coming months which process, or combination of processes, generated its regional, classic-style tsunami.
But nearly three hours before that major tsunami crossed the Pacific Ocean and reached Japan, small wave peaks arrived in the Ogasawara Islands, about 1,000 kilometers south of Tokyo. That same day, similar peaks showed up in the Caribbean Sea from Puerto Rico to Mexico, and even in the Mediterranean Sea, 18,000 kilometers away from the eruption.
These small, fast waves reminded some scientists of a less conventional way in which Earth can make tsunamis: by using the atmosphere.
Storms can sometimes produce sustained and significant atmospheric disturbances. In 1929, the British mathematician and oceanographer Joseph Proudman hypothesized that if a disturbance moves at a certain speed above a body of water, it can initiate something now called a Proudman resonance. His equations showed that the atmospheric pressure wave can transfer energy to the waves in the water, making them bigger. And when these amped-up waves hit the coast, they’re known as meteotsunamis.
Proudman’s math ultimately showed that the transfer of energy from sky to sea is most efficient when an atmospheric disturbance travels at the same speed as the water waves. And the speed of water waves depends on the depth of the water.
Merrill Sherman/Quanta Magazine
Storms tend to produce atmospheric pressure waves that travel tens of centimeters per second, said Alexander Rabinovich, an expert on meteotsunamis at the Institute of Ocean Sciences in Sidney, British Columbia. At that slow speed, the pressure waves resonate with similarly slow water waves found on shallow bodies of water to trigger major meteotsunamis.
This is why they show up dozens of times per year in the relatively shallow waters of the U.S. East Coast, the Gulf of Mexico and the Great Lakes, sometimes to lethal effect: A 3-meter-high meteotsunami on Lake Michigan in 1954 killed seven people. On rare occasions, these meteotsunamis can rival the threat of quake-made tsunamis: In 1978, meteotsunami waves measuring 6 meters high terrorized Croatia’s harbor town of Vela Luka, crashing into it repeatedly for several hours.
The tsunamis caused by Tonga’s January eruption were very similar to meteotsunamis — as numerous oceanographers, physicists and volcanologists realized in near-unison just hours after the volcano’s explosive zenith. “All of the mechanics and physics are quite similar,” said Eric Anderson, a researcher at the Colorado School of Mines who studies interactions between the hydrosphere, cryosphere and atmosphere. Except here, there is one key difference. “The entire globe is affected,” said Rabinovich.
Roar Heard Around the World
Researchers don’t yet agree about the specific type of pressure wave, or combination of types, that’s responsible in this case. The Tonga eruption produced multiple atmospheric disturbances, including a short-lived shock wave and globe-trotting acoustic waves. By pushing lots of air upward out of its way, the explosion also made what are called atmospheric gravity waves. These are created when a cold air mass rapidly rises, then obeys gravity and sinks back down to sea level, essentially striking the stratosphere like a gong and generating pressure oscillations that propagate horizontally in all directions.
Many researchers consider Tonga’s atmospheric gravity waves the most likely source of the far-flung tsunamis, given the specific locations where the tsunamis appeared. The gravity waves zipped across the planet at speeds reaching or exceeding 300 meters per second. To get a Proudman resonance, water waves would have to be moving comparably fast, and that requires unusually deep water. “To have an ocean wave going that fast, you’re going to need about 9,000 meters of water,” said Dusek, “and there’s not many places that you get down to that water depth.” It’s telling, then, that strong tsunamis cropped up in the Caribbean Sea and south of Japan, where ocean trenches surpass 8,000 meters in depth.
In these locations, sea waves really do move at speeds close to the speed of sound. That may be difficult to envisage, but then, waves are largely invisible in the open ocean. “Until you hit that shallow water, the wave’s not as evident on the sea surface,” Dusek said.
According to Eric Geist, a geophysicist at the U.S. Geological Survey’s Pacific Coastal and Marine Science Center, although the Proudman resonance may have been most efficient atop those two trenches, even elsewhere, as the atmospheric gravity waves moved across the open ocean, some resonance and sea wave amplification would have happened. The underwater topography of the seafloor close to affected shorelines may have also amplified incoming tsunami waves.
Along with the deep-trench theory, these ideas require further investigation before firm conclusions can be made. And other types of pressure waves may have further amplified wave heights. But even at this early stage, no one doubts that Tonga’s dramatic eruption made seas and oceans on the other side of the world cower, crest and convulse — a revelation in the present that all but confirms a remarkable notion from the past.
Quirks of Physics
Given the “strong similarity,” in Rabinovich’s words, between the Tonga and Krakatau events, the cold case of Krakatau’s worldwide tsunamis can be closed. But as in Krakatau, the pandemonium in Tonga has raised new queries.
The distant tsunamis may not have been dangerous, but the local tsunami caused by the Tonga volcano itself certainly was. Tonga has a deep trench of its own, so it seems likely that a Proudman resonance was generated close to the eruption too. “Did it add to the peak wave height a little more than what would be expected from just the seismic signal?” asked Dusek.
That the wave heights never came close to those of some storm-made meteotsunamis came as a relief. But will that always be the case? Researchers wonder how powerful a blast you would need to create a much larger meteotsunami event. Volcanoes on land can also create big booms, Lane noted — so are they capable of causing distant tsunamis too?
Volcanic explosions as fearsome as Krakatau’s and Hunga Tonga-Hunga Ha‘apai’s are extraordinarily infrequent on human timescales. And so far, it seems as if such monstrous bellowing is incapable of causing severe wave peaks worldwide. When it comes to tsunamis caused by the atmosphere, “weather-driven meteotsunamis are still really what we’re concerned with from a public safety standpoint,” said Dusek.
Tonga’s marine manifestation wasn’t a horror show. But it did remind scientists that nature’s deployment of even the smallest quirks of physics can lead to global consequences.