Spreading the Word on a Possible Alzheimer’s Treatment
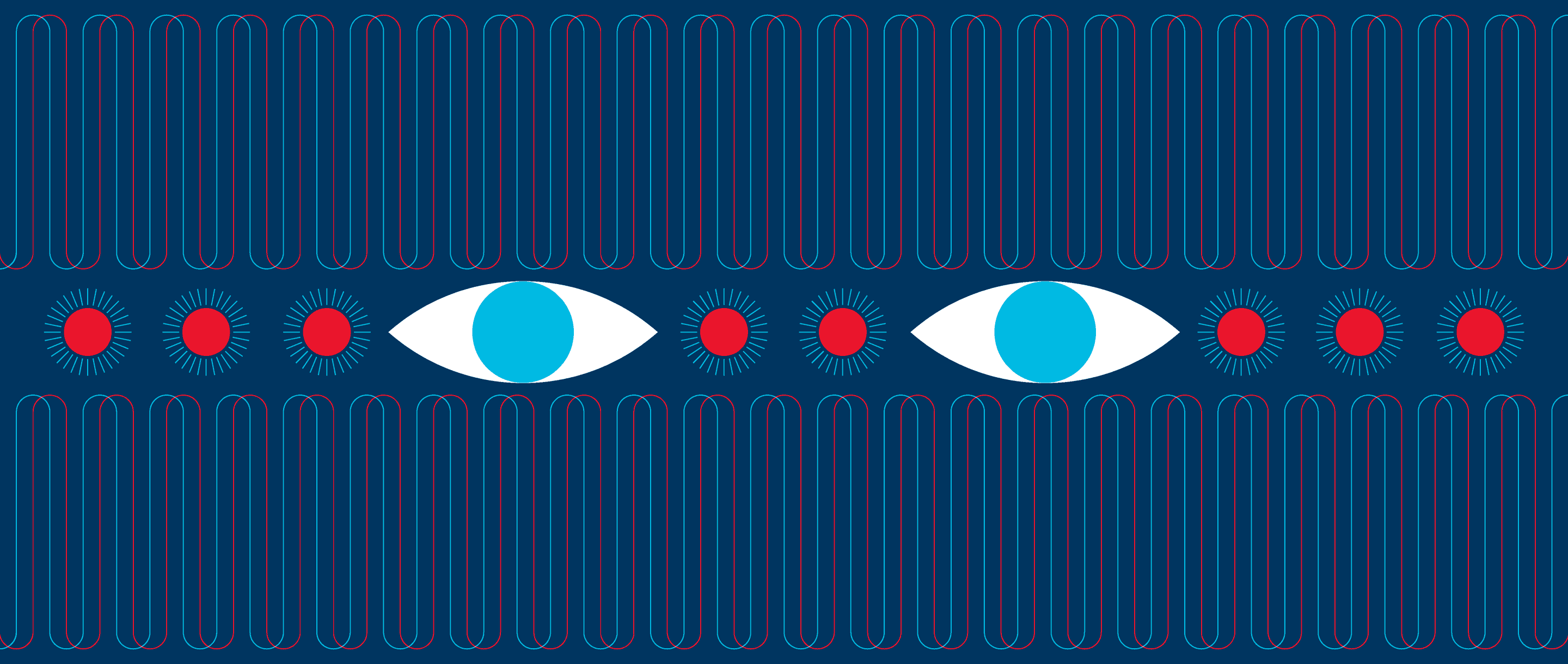
James O’Brien for Quanta Magazine
Introduction
Discoveries that transcend boundaries are among the greatest delights of scientific research, but such leaps are often overlooked because they outstrip conventional thinking. Take, for example, a new discovery for treating dementia that defies received wisdom by combining two formerly unrelated areas of research: brain waves and the brain’s immune cells, called microglia. It’s an important finding, but it still requires the buy-in and understanding of researchers to achieve its true potential. The history of brain waves shows why.
In 1887, Richard Caton announced his discovery of brain waves at a scientific meeting. “Read my paper on the electrical currents of the brain,” he wrote in his personal diary. “It was well received but not understood by most of the audience.” Even though Caton’s observations of brain waves were correct, his thinking was too unorthodox for others to take seriously. Faced with such a lack of interest, he abandoned his research and the discovery was forgotten for decades.
Flash forward to October 2019. At a gathering of scientists that I helped organize at the annual meeting of the Society for Neuroscience in Chicago, I asked if anyone knew of recent research by neuroscientists at the Massachusetts Institute of Technology who had found a new way to treat Alzheimer’s disease by manipulating microglia and brain waves. No one replied.
I understood: Scientists must specialize to succeed. Biologists studying microglia don’t tend to read papers about brain waves, and brain wave researchers are generally unaware of glial research. A study that bridges these two traditionally separate disciplines may fail to gain traction. But this study needed attention: Incredible as it may sound, the researchers improved the brains of animals with Alzheimer’s simply by using LED lights that flashed 40 times a second. Even sound played at this charmed frequency, 40 hertz, had a similar effect.
Today, brain waves are a vital part of neuroscience research and medical diagnosis, though doctors have never manipulated them to treat degenerative disease before now. These oscillating electromagnetic fields are produced by neurons in the cerebral cortex firing electrical impulses as they process information. Much as people clapping their hands in synchrony generate thunderous rhythmic applause, the combined activity of thousands of neurons firing together produces brain waves.
These waves come in various forms and in many different frequencies. Alpha waves, for example, oscillate at frequencies of 8 to 12 hertz. They surge when we close our eyes and shut out external stimulation that energizes higher-frequency brain wave activity. Rapidly oscillating gamma waves, which reverberate at frequencies of 30 to 120 hertz, are of particular interest in Alzheimer’s research, because their period of oscillation is well matched to the hundredth-of-a-second time frame of synaptic signaling in neural circuits. Brain waves are important in information processing because they can influence neuronal firing. Neurons fire an electrical impulse when the voltage difference between the inside and outside of the neuron reaches a certain trigger point. The peaks and troughs of voltage oscillations in brain waves nudge the neuron closer to the trigger point or farther away from it, thereby boosting or inhibiting its tendency to fire. The rhythmic voltage surging also groups neurons together, making them fire in synchrony as they “ride” on different frequencies of brain waves.
I already knew that much, so to better understand the new work and its origins, I sought out Li-Huei Tsai, a neuroscientist at MIT. She said the idea of using one of these frequencies to treat Alzheimer’s came from a curious observation. “We had noticed in our own data, and in that of other groups, that 40-hertz rhythm power and synchrony are reduced in mouse models of Alzheimer’s disease,” she said, as well as in patients with the disease. Apparently, if you have Alzheimer’s, your brain doesn’t produce strong brain waves in that particular frequency. In 2016, her graduate student Hannah Iaccarino reasoned that perhaps boosting the power of these weakened gamma waves would be helpful in treating this severe and irreversible dementia.
To increase gamma wave power, the team turned to optogenetic stimulation, a novel technique that allows researchers to control how and when individual neurons fire by shining lasers directly into them, via fiber-optic cables implanted in the brain. Tsai’s team stimulated neurons in the visual cortex of mice with Alzheimer’s, making them fire impulses at 40 hertz. The results, published in 2016 in Nature, showed a marked reduction in amyloid plaques, a hallmark of the disease.
It was a good indication that these brain waves might help, but Tsai’s team knew that an optogenetic approach wasn’t an option for humans with the disease, because of ethical concerns. They began to look for other ways of increasing the brain’s gamma wave activity. Tsai’s MIT colleague Emery Brown pointed her to an older paper showing that you can boost the power of gamma waves in a cat’s brain simply by having it stare at a screen illuminated by a strobe light flickering at certain frequencies, which included 40 hertz. “Hannah and our collaborators built a system to try that sensory stimulation in mice, and it worked,” Tsai told me. The thinking is that the flashing lights whip up gamma waves because the rhythmic sensory input sets neural circuits “rocking” at this frequency, like when people rock a stuck car out of a rut by pushing together in rhythm.
In fact, the strobe lights had an additional effect on mice: They also cleared out amyloid plaques. But it wasn’t clear exactly how the optogenetic stimulation or the flashing-light therapy could do that.
Following a clue from Alois Alzheimer himself, the researchers quickly shifted their attention from neurons to microglia. In Alzheimer’s first description of brain tissue taken from patients with “presenile dementia,” which he examined under a microscope near the turn of the 20th century, he noted that the deposits of amyloid plaques were surrounded by these immune cells. Subsequent research confirmed that microglia engulf the plaques pockmarking these patients’ brains.
Tsai and colleagues decided to check out these immune cells in the animals whose brain waves they’d boosted. They observed that microglia in all the treated animals had bulked up in size, and more of them were digesting amyloid plaques.
How did these cells know to do this? Unlike immune cells in the bloodstream, which are unaware of neuronal transmissions, the brain’s microglia are tuned in to the rhythms of electrical activity in the brain. While immune cells in the bloodstream and microglia in the brain both have cellular sensors to detect disease and injury, microglia can also detect neurons firing electrical impulses. That’s because they have the same neurotransmitter receptors that neurons use to transmit signals through synapses. This gives microglia the ability to “listen in” on information flowing through neural networks and, when those transmissions are disturbed, to take action to repair the circuitry. Thus, the right brain waves can drive microglia to consume the toxic protein deposits.
“I find this intersection [between brain waves and microglia] to be one of the most exciting and intriguing results of our work,” Tsai told me. Her team reported last year in Neuron that prolonging the LED strobe-light flashing for three to six weeks not only cleared out the toxic plaques in mice brains but also prevented neurons from dying and even preserved synapses, which dementia can destroy.
The team wanted to know if other types of rhythmic sensory input could also rock the neural circuits like a stuck car, producing gamma waves that resulted in fewer amyloid plaques. In an expanded study in Cell, they reported that just as seeing flashes at 40 hertz resulted in fewer plaques in the visual cortex, sound stimulation at 40 hertz reduced amyloid protein in the auditory cortex. Other regions were similarly affected, including the hippocampus — crucial for learning and memory — and the treated mice performed better on memory tests. Exposing the mice to both stimuli, a light show synchronized with pulsating sound, had an even more powerful effect, reducing amyloid plaques in regions throughout the cerebral cortex, including the prefrontal region, which carries out higher-level executive functions that are impaired in Alzheimer’s.
I was amazed, so just to make sure I wasn’t getting unduly excited about the possibility of using flashing lights and sounds to treat humans, I talked to Hiroaki Wake, a neuroscientist at Kobe University in Japan who was not involved with the work. “It would be fantastic!” he said. “The treatment may also be effective for a number of neurodegenerative disorders like Parkinson’s disease and ALS,” where microglia also play a role. He notes, however, that while the link between microglia and brain oscillations is well founded, the biological mechanism by which 40-hertz stimulation prods microglia into removing the plaques and rescuing neurons from destruction remains unknown.
Tsai said the mystery may be solved soon. A team of researchers at the Georgia Institute of Technology, including Tsai lab veteran Annabelle Singer, laid out a possibility in a February paper. They reported that in normal mice, gamma stimulation with LED lights rapidly induced microglia to generate cytokines, proteins that neurons (and immune cells generally) use to signal one another. They’re one of the main regulators of neuroinflammation in response to brain injury and disease, and the microglia released them surprisingly quickly, within just 15 to 60 minutes of the stimulation. “These effects are faster than you see with many drugs that target immune signaling or inflammation,” Singer said.
Cytokines come in many forms, and the study found that getting the microglia to produce different kinds required specific frequencies. “Neural stimulation doesn’t just turn immune signaling on,” Singer said. It took a particular rhythm to produce these particular proteins. “Different types of stimulation could be used to tune immune signaling as desired.”
That means doctors could potentially treat different diseases just by varying the light and sound rhythms they use. The different stimuli would rock the neurons into producing appropriate brain wave frequencies, causing nearby microglia to release specific types of cytokines, which tell microglia in general how to go to work repairing the brain.
Of course, it may still be a while before such treatments are available for patients. And even then, there may be side effects. “Rhythmic sensory stimulation likely affects many types of cells in brain tissue,” Tsai said. “How each of them senses and responds to gamma oscillations is unknown.” Wake also pointed out that rhythmic stimulation could do more harm than good, because such stimuli could induce seizures, common in many psychiatric and neurodegenerative disorders.
Still, the potential benefits are great. Tsai’s team has just begun assessing their strobe-light method on patients, and they’re sure to be joined by others as more researchers learn of this promising work. (Most experts I talked to were not aware of this research until I asked.)
Just as new species spring up at the boundaries between ecosystems, new science can flourish at the interface between disciplines. It takes a sharp eye to spot it, but as Richard Caton found, it can also require a bit of persuasion to convince others.