The Hunt for Earth’s Deep Hidden Oceans
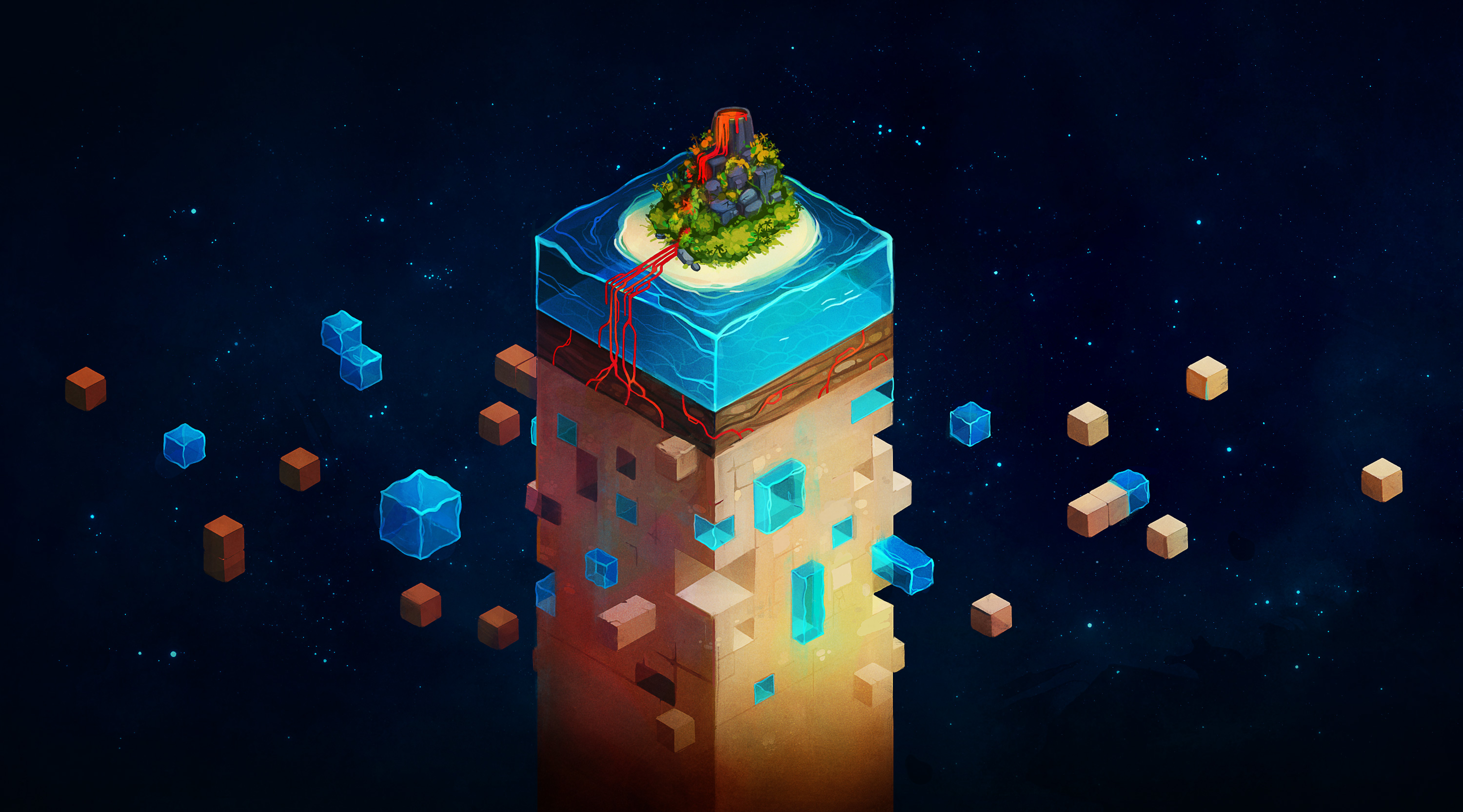
Olena Shmahalo/Quanta Magazine
Introduction
A couple hundred pebble-size diamonds, plucked from Brazilian mud, sit inside a safe at Northwestern University. To some, they might be worthless. “They’re battered,” said Steve Jacobsen, a mineralogist at Northwestern. “They look like they’ve been through a washing machine.” Many are dark or yellow, far from the pristine gems of jewelers’ dreams.
Yet, for researchers like Jacobsen, these fragments of crystalline carbon are every bit as precious — not for the diamond itself, but for what is locked inside: specks of minerals forged hundreds of kilometers underground, deep in Earth’s mantle.
These mineral flecks — some too small to see even under a microscope — offer a peek into Earth’s otherwise unreachable interior. In 2014, researchers glimpsed something embedded in these minerals that, if not for its deep origins, would’ve been unremarkable: water.
Not actual drops of water, or even molecules of H20, but its ingredients, atoms of hydrogen and oxygen embedded in the crystal structure of the mineral itself. This hydrous mineral isn’t wet. But when it melts, out spills water. The discovery was the first direct proof that water-rich minerals exist this deep, between 410 and 660 kilometers down, in a region called the transition zone, sandwiched between the upper and lower mantles.
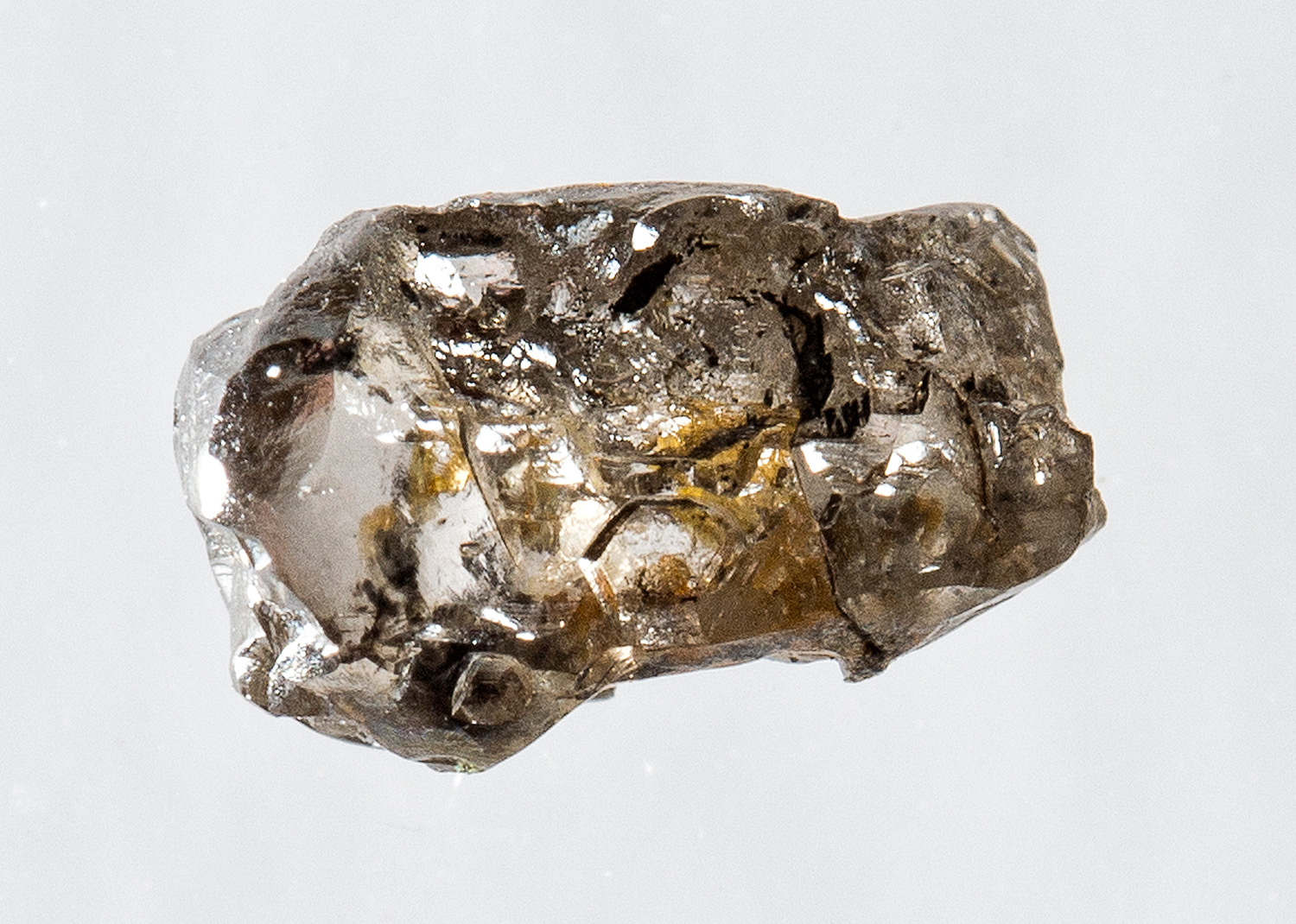
This diamond from Juína, Brazil, originally formed deep inside Earth. It contains an inclusion of ringwoodite, a water-bearing mineral.
University of Alberta
Since then, scientists have found more tantalizing evidence of water. In March, a team announced that they had discovered diamonds from Earth’s mantle that have actual water encased inside. Seismic data has also mapped water-friendly minerals across a large portion of Earth’s interior. Some scientists now argue that a huge reservoir of water could be lurking far beneath our feet. If we consider all of the planet’s surface water as one ocean, and there turn out to be even a few oceans underground, it would change how scientists think of Earth’s interior. But it also raises another question: Where could it have all come from?
Water World
Without water, life as we know it would not exist. Neither would the living, dynamic planet we’re familiar with today. Water plays an integral role in plate tectonics, triggering volcanoes and helping parts of the upper mantle flow more freely. Still, most of the mantle is relatively dry. The upper mantle, for instance, is primarily made of a mineral called olivine, which can’t store much water.
But below 410 kilometers, in the transition zone, high temperatures and pressures squeeze the olivine into a new crystal configuration called wadsleyite. In 1987, Joe Smyth, a mineralogist at the University of Colorado, realized that wadsleyite’s crystal structure would be afflicted with gaps. These gaps turn out to be perfect fits for hydrogen atoms, which could snuggle into these defects and bond with the adjacent oxygen atoms already in the mineral. Wadsleyite, Smyth found, can potentially grab onto lots of hydrogen, turning it into a hydrous mineral that produces water when it melts. For scientists like Smyth, hydrogen means water.
Deeper in the transition zone, wadsleyite becomes ringwoodite. And in the lab, Jacobsen (who was Smyth’s graduate student in the 1990s) would squeeze and heat bits of ringwoodite to mimic the extreme conditions of the transition zone. Researchers doing similar experiments with both wadsleyite and ringwoodite found that in the transition zone, these minerals could hold 1 to 3 percent of their weight in water. Considering that the transition zone is a roughly 250-kilometer-thick shell that accounts for about 7 percent of Earth’s mass (by comparison, the crust is only 1 percent), it could contain several times the water of Earth’s oceans.
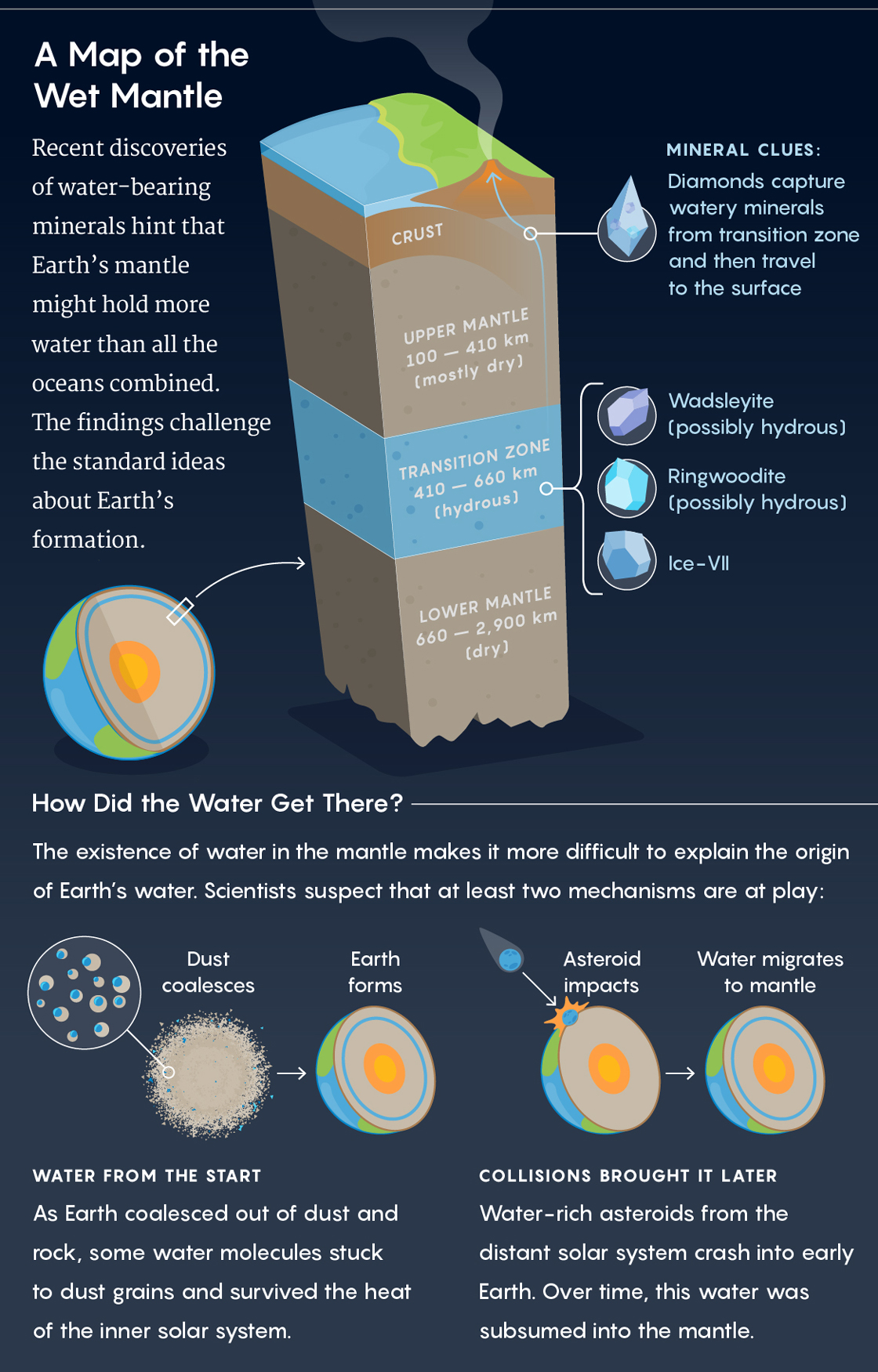
Lucy Reading-Ikkanda/Quanta Magazine
These experiments, however, only gauge water capacity. “It’s not a measurement of how wet the sponge is, it’s a measurement of how much the sponge can hold,” said Wendy Panero, a geophysicist at Ohio State University.
Neither were the experiments necessarily realistic, since researchers could only test lab-grown ringwoodite. Apart from a few meteorites, no one had ever seen ringwoodite in nature. That is, until 2014.
Tantalizing Clues
While soccer fans converged on Brazil for the 2014 World Cup, a small group of geologists headed to the farmlands around Juína, a city almost 2,000 kilometers west of Brasilia. They were on the hunt for diamonds that had been panned from local rivers.
As diamonds form in the heat and high pressure of the mantle, they can trap bits of minerals. Because diamonds are so tough and rigid, they preserve these mantle minerals as they’re blasted to the surface via volcanic eruptions.
The researchers bought more than a thousand of the most speckled, mineral-filled crystals. One of the scientists, Graham Pearson, took several hundred back to his lab at the University of Alberta, where, inside one particular diamond, he and his colleagues discovered ringwoodite from the transition zone. Not only that, but it was hydrous ringwoodite, which meant it contained water — about 1 percent by weight.
“It’s an important discovery in terms of plausibility,” said Brandon Schmandt, a seismologist at the University of New Mexico. For the first time, scientists had a sample of the transition zone — and it was hydrated. “It’s definitely not crazy, then, to think other parts of the transition zone are also hydrated.”
But, he added, “it would also be a little crazy to think that one crystal represents the average of the entire transition zone.” Diamonds, after all, form only in certain conditions, and this sample might come from a uniquely watery place.
To see how widespread hydrous ringwoodite could be, Schmandt teamed with Jacobsen and others to map it using seismic waves. Due to convection, hydrous ringwoodite can sink, and as it drops below the transition zone, the rising pressure wrings water out, causing the mineral to melt. Just beneath the transition zone where mantle material is descending, these pools of molten minerals can abruptly slow seismic waves. By measuring seismic speeds under North America, the researchers found that, indeed, such pools appear common below the transition zone. Another study measuring the seismic waves under the European Alps found a similar pattern.
Abundant mantle water got yet another boost in March when a team led by Oliver Tschauner, a mineralogist at the University of Nevada, Las Vegas, discovered diamonds that contain actual pieces of water ice — the first observation of freely existing H2O from the mantle. The samples might say more about the wet conditions that formed the diamond than the existence of any ubiquitous reservoir. But because this water — a high-pressure form called ice-VII — was found in a variety of locations across southern Africa and China, it could turn out to be relatively widespread.
“A couple years from now, we’ll find ice-VII is much more common,” said Steve Shirey, a geologist at the Carnegie Institution for Science. “It’s telling us we have the same story that hydrous ringwoodite is telling us.”
But if the story is that the mantle is brimming with water, the cliffhanger leaves us wondering how it all got there.
Watery Origins
According to the standard tale, Earth’s water was imported. The region around the sun where the planet formed was too hot for volatile compounds like water to condense. So the nascent Earth started out dry, getting wet only after water-rich bodies from the distant solar system crashed into the planet, delivering water to the surface. Most of these were likely not comets but rather asteroids called carbonaceous chondrites, which can be up to 20 percent water by weight, storing it in a form of hydrogen like ringwoodite.
But if there’s a huge stockpile of water in the transition zone, this story of water’s origin would have to change. If the transition zone could store 1 percent of its weight in water — a moderate estimate, Jacobsen said — it would contain twice the world’s oceans. The lower mantle is much drier but also voluminous. It could amount to all the world’s oceans (again). There’s water in the crust, too. For subduction to incorporate that much water from the surface at the current rate, it would take much longer than the age of the planet, Jacobsen said.
If that’s the case, at least some of Earth’s interior water must have always been here. Despite the heat in the early solar system, water molecules could have stuck to the dust particles that coalesced to form Earth, according to some theories.
Yet the total amount of water in the mantle is a highly uncertain figure. At the low end, the mantle might hold only half as much water as in the world’s oceans, according to Schmandt and others.
On the high end, the mantle could hold two or three times the amount of water in the oceans. If there were much more than that, the additional heat of the younger Earth would have made the mantle too watery and runny to fracture the continental plates, and today’s plate tectonics may never have gotten started. “If you have a bunch of water in the surface, it’s great,” said Jun Korenaga, a geophysicist at Yale University. “If you have a bunch of water in the mantle, it’s not great.”
But many uncertainties remain. One big question mark is the lower mantle, where extreme pressures turn ringwoodite into bridgmanite, which can’t hold much water at all. Recent studies, however, suggest the presence of new water-bearing minerals dubbed phase D and phase H. Exactly what these minerals are like and how much water they might store remains an open question, Panero said. “Because it is a wide-open question, I think that the water content in the mantle remains open for debate — wide open.”
Measuring Earth’s interior water storage isn’t easy. One promising way is to measure the electrical conductivity of the mantle, Korenaga said. But those techniques aren’t yet as advanced as, say, using seismic waves. And while seismic waves offer a global view of Earth’s interior, the picture isn’t always clear. The signals are subtle, and researchers need more precise data and a better understanding of the properties of more realistic mantle material, instead of just ringwoodite and wadsleyite. Those two minerals constitute about 60 percent of the transition zone, the rest being a complex mix of other minerals and compounds.
Finding more diamonds with hydrous minerals would help, too. In Jacobsen’s lab, that job falls to graduate student Michelle Wenz. For each diamond, she uses powerful X-rays at Argonne National Laboratory to map the location of every mineral speck, of which there may be half a dozen. Then, to identify the minerals, she blasts X-rays onto each bit and measures how the rays scatter off its crystal structure. Of the hundreds of diamonds in the lab, all from Brazil, she’s gone through about 60. No water yet.
Water or not, she said, these capsules from the deep are still amazing. “Each one is so unique,” she said. “They’re a lot like snowflakes.”
Correction: This article was revised on July 11, 2018, to correct a typographical error; it is the mantle, not the ocean, that could hold two or three times the amount of water in the oceans.