The Spooky Quantum Phenomenon You’ve Never Heard Of
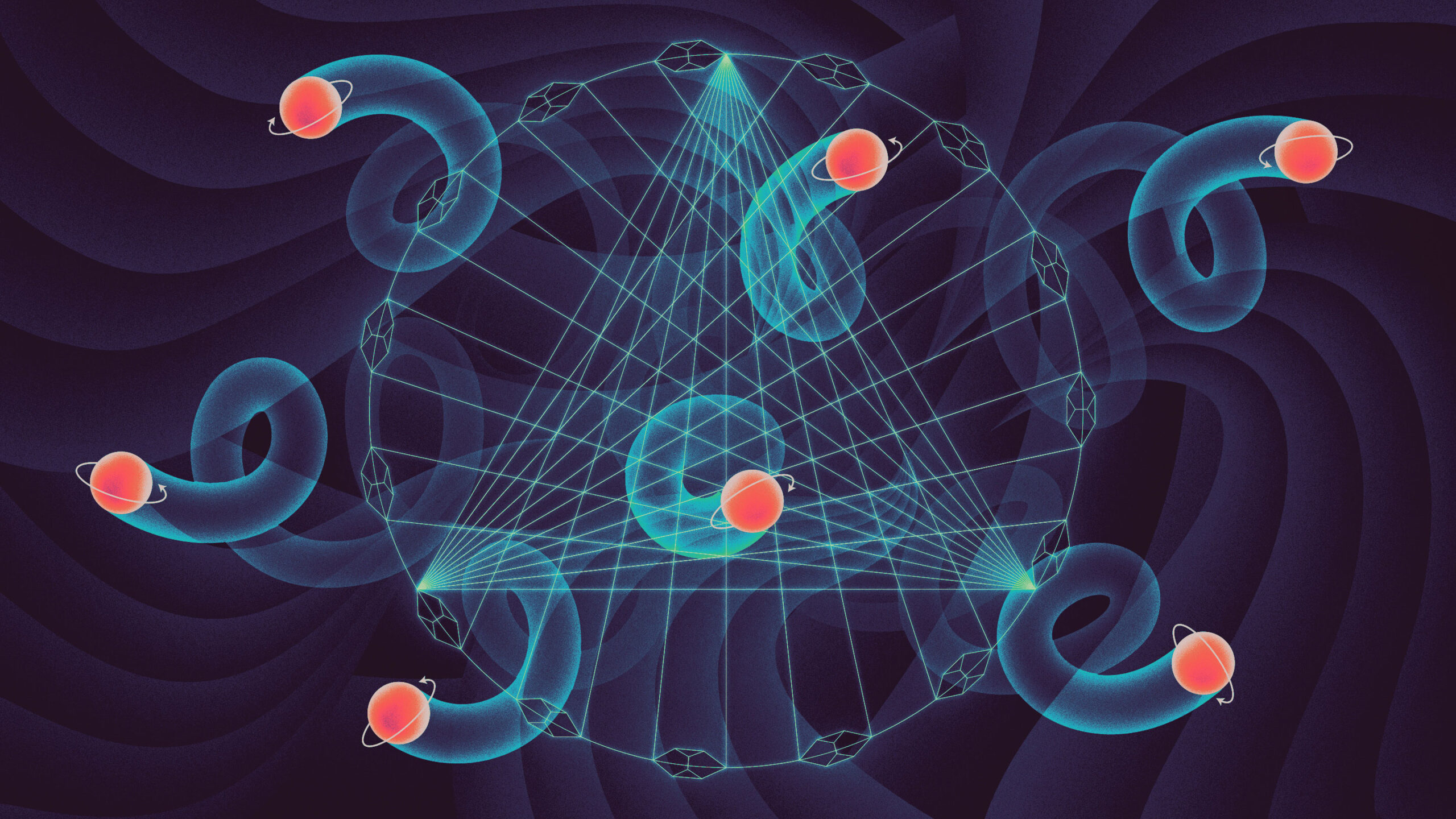
In their proof that the world is contextual, Simon Kochen and Ernst Specker created a network of possible values of a particle’s spin measured in different directions.
Kristina Armitage for Quanta Magazine
Introduction
Perhaps the most famously weird feature of quantum mechanics is nonlocality: Measure one particle in an entangled pair whose partner is miles away, and the measurement seems to rip through the intervening space to instantaneously affect its partner. This “spooky action at a distance” (as Albert Einstein called it) has been the main focus of tests of quantum theory.
“Nonlocality is spectacular. I mean, it’s like magic,” said Adán Cabello, a physicist at the University of Seville in Spain.
But Cabello and others are interested in investigating a lesser-known but equally magical aspect of quantum mechanics: contextuality. Contextuality says that properties of particles, such as their position or polarization, exist only within the context of a measurement. Instead of thinking of particles’ properties as having fixed values, consider them more like words in language, whose meanings can change depending on the context: “Time flies like an arrow. Fruit flies like bananas.”
Although contextuality has lived in nonlocality’s shadow for over 50 years, quantum physicists now consider it more of a hallmark feature of quantum systems than nonlocality is. A single particle, for instance, is a quantum system “in which you cannot even think about nonlocality,” since the particle is only in one location, said Bárbara Amaral, a physicist at the University of São Paulo in Brazil. “So [contextuality] is more general in some sense, and I think this is important to really understand the power of quantum systems and to go deeper into why quantum theory is the way it is.”
Researchers have also found tantalizing links between contextuality and problems that quantum computers can efficiently solve that ordinary computers cannot; investigating these links could help guide researchers in developing new quantum computing approaches and algorithms.
And with renewed theoretical interest comes a renewed experimental effort to prove that our world is indeed contextual. In February, Cabello, in collaboration with Kihwan Kim at Tsinghua University in Beijing, China, published a paper in which they claimed to have performed the first loophole-free experimental test of contextuality.
The 117th Direction
The Northern Irish physicist John Stewart Bell is widely credited with showing that quantum systems can be nonlocal. By comparing the outcomes of measurements of two entangled particles, he showed with his eponymous theorem of 1965 that the high degree of correlations between the particles can’t possibly be explained in terms of local “hidden variables” defining each one’s separate properties. The information contained in the entangled pair must be shared nonlocally between the particles.
Bell also proved a similar theorem about contextuality. He and, separately, Simon Kochen and Ernst Specker showed that it is impossible for a quantum system to have hidden variables that define the values of all their properties in all possible contexts.
In Kochen and Specker’s version of the proof, they considered a single particle with a quantum property called spin, which has both a magnitude and a direction. Measuring the spin’s magnitude along any direction always results in one of two outcomes: 1 or 0. The researchers then asked: Is it possible that the particle secretly “knows” what the result of every possible measurement will be before it is measured? In other words, could they assign a fixed value — a hidden variable — to all outcomes of all possible measurements at once?
Quantum theory says that the magnitudes of the spins along three perpendicular directions must obey the “101 rule”: The outcomes of two of the measurements must be 1 and the other must be 0. Kochen and Specker used this rule to arrive at a contradiction. First, they assumed that each particle had a fixed, intrinsic value for each direction of spin. They then conducted a hypothetical spin measurement along some unique direction, assigning either 0 or 1 to the outcome. They then repeatedly rotated the direction of their hypothetical measurement and measured again, each time either freely assigning a value to the outcome or deducing what the value must be in order to satisfy the 101 rule together with directions they had previously considered.
They continued until, in the 117th direction, the contradiction cropped up. While they had previously assigned a value of 0 to the spin along this direction, the 101 rule was now dictating that the spin must be 1. The outcome of a measurement could not possibly return both 0 and 1. So the physicists concluded that there is no way a particle can have fixed hidden variables that remain the same regardless of context.
While the proof indicated that quantum theory demands contextuality, there was no way to actually demonstrate this through 117 simultaneous measurements of a single particle. Physicists have since devised more practical, experimentally implementable versions of the original Bell-Kochen-Specker theorem involving multiple entangled particles, where a particular measurement on one particle defines a “context” for the others.
Question by Question
In 2009, contextuality, a seemingly esoteric aspect of the underlying fabric of reality, got a direct application: One of the simplified versions of the original Bell-Kochen-Specker theorem was shown to be equivalent to a basic quantum computation.
The proof, named Mermin’s star after its originator, David Mermin, considered various combinations of contextual measurements that could be made on three entangled quantum bits, or qubits. The logic of how earlier measurements shape the outcomes of later measurements has become the basis for an approach called measurement-based quantum computing. The discovery suggested that contextuality might be key to why quantum computers can solve certain problems faster than classical computers — an advantage that researchers have struggled mightily to understand.
Robert Raussendorf, a physicist at the University of British Columbia and a pioneer of measurement-based quantum computing, showed that contextuality is necessary for a quantum computer to beat a classical computer at some tasks, but he doesn’t think it’s the whole story. Whether contextuality powers quantum computers is “probably not exactly the right question to ask,” he said. “But we need to get there question by question. So we ask a question that we understand how to ask; we get an answer. We ask the next question.”
A Loophole-Free Test
Some researchers have suggested loopholes around Bell, Kochen and Specker’s conclusion that the world is contextual. They argue that context-independent hidden variables haven’t been conclusively ruled out.
In February, Cabello and Kim announced that they had closed every plausible loophole by performing a “loophole free” Bell-Kochen-Specker experiment.
The experiment entailed measuring the spins of two entangled trapped ions in various directions, where the choice of measurement on one ion defined the context for the other ion. The physicists showed that, although making a measurement on one ion does not physically affect the other, it changes the context and hence the outcome of the second ion’s measurement.
Skeptics would ask: How can you be certain that the context created by the first measurement is what changed the second measurement outcome, rather than other conditions that might vary from experiment to experiment? Cabello and Kim closed this “sharpness loophole” by performing thousands of sets of measurements and showing that the outcomes don’t change if the context doesn’t. After ruling out this and other loopholes, they concluded that the only reasonable explanation for their results is contextuality.
Cabello and others think that these experiments could be used in the future to test the level of contextuality — and hence, the power — of quantum computing devices.
“If you want to really understand how the world is working,” said Cabello, “you really need to go into the detail of quantum contextuality.”