To See Black Holes in Stunning Detail, She Uses ‘Echoes’ Like a Bat
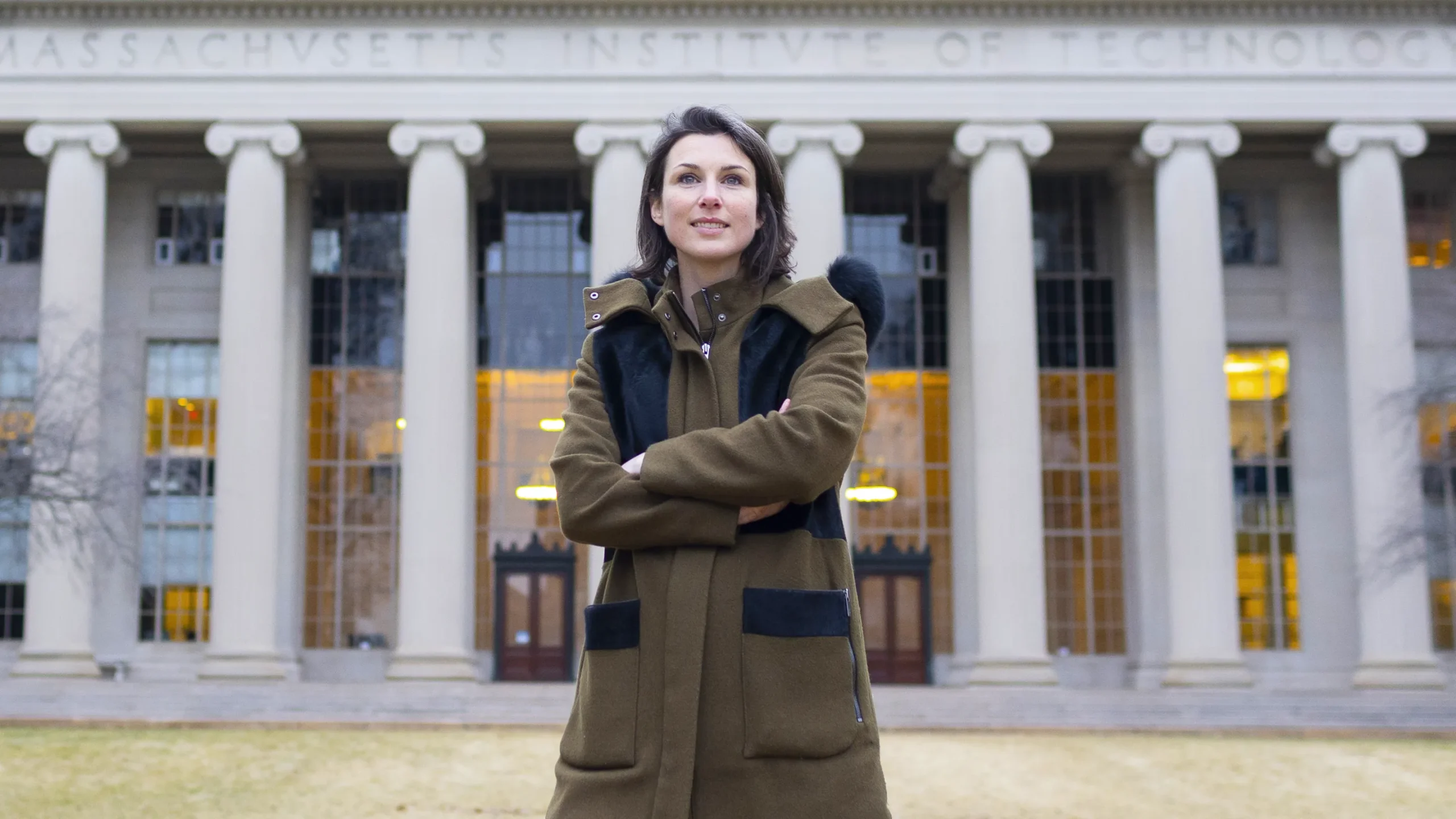
Erin Kara studies the supermassive black holes that pin galaxies to the fabric of the cosmos.
Katherine Taylor for Quanta Magazine
Introduction
Unlike many astronomers, Erin Kara didn’t grow up star-struck. “I don’t have that story of being that little kid staring up at the stars and wanting to do this, which gave me some anxiety,” she said. “Did that make me a fake?”
But after discovering astrophysics as an undergraduate, Kara was hooked. Now an observational astrophysicist at the Massachusetts Institute of Technology, she visits mind-bending territory. Like an astronaut in the film Interstellar, she explores the regions near gargantuan black holes. Her goal is to better understand how those black holes behave and how they continually reshape galaxies across the universe.
Packed with mass equal to millions or billions of suns, supermassive black holes lurk at the center of nearly every galaxy. Energetically, these black holes are like galaxies’ dark, beating hearts.
“They’re not just ornamental. They’re not just sitting there passively,” Kara said. “They’re actually dictating how the galaxy evolves and why the galaxy looks the way that it does.”
Kara tries to understand the environment near these black holes, where most of their energy is released. By carefully tracking the gas and plasma that swirls near a black hole — forming what’s known as an accretion disk — she can approximate the black hole’s mass, for example. That nearby gas and plasma can also help reveal how feasting black holes create extreme cosmic structures such as relativistic jets — gigantic beams of superheated plasma accelerated to nearly the speed of light.
But there’s a huge problem that Kara and other researchers must overcome: These target black holes are so far away that conventional imaging techniques can’t resolve their immediate surroundings. To reconstruct the immediate environment around a black hole, Kara turns to the X-ray light given off by the accretion disk. She measures subtle delays in when that light reaches Earth. By doing so, she can infer the structure of the gas and plasma with remarkable resolution. Kara’s work on this method — called reverberation mapping — has exposed black holes’ feeding frenzies in unprecedented detail, with findings that include the first X-ray “echoes” ever seen from a black hole shredding a star. Kara received the American Astronomical Society’s 2022 Newton Lacy Pierce Prize, which recognizes outstanding achievements in observational astronomy over the preceding five years.
Quanta Magazine recently spoke with Kara about her path into science, the nuts and bolts of reverberation mapping, and the black holes that keep her up at night. The interview has been condensed and edited for clarity.
You spend a lot of time thinking about black holes, to the point where you have even converted simulations of them into sounds. Many people think of black holes as “weird” or “spooky” or “eerie.” Do you buy that characterization?
There’s this stereotype that black holes are nasty things that we should all be afraid of, but what’s amazing is that black holes actually give us life, if you want to take it to that level of abstraction. The reason we’re all here is that the gas in our galaxy is distributed such that stars could form, which eventually led to the planet that we live on. This was, in some ways, dictated by our galaxy’s central black hole.
Black holes aren’t, like, a demise and the end of everything. They’re actually, in some ways, a beginning.
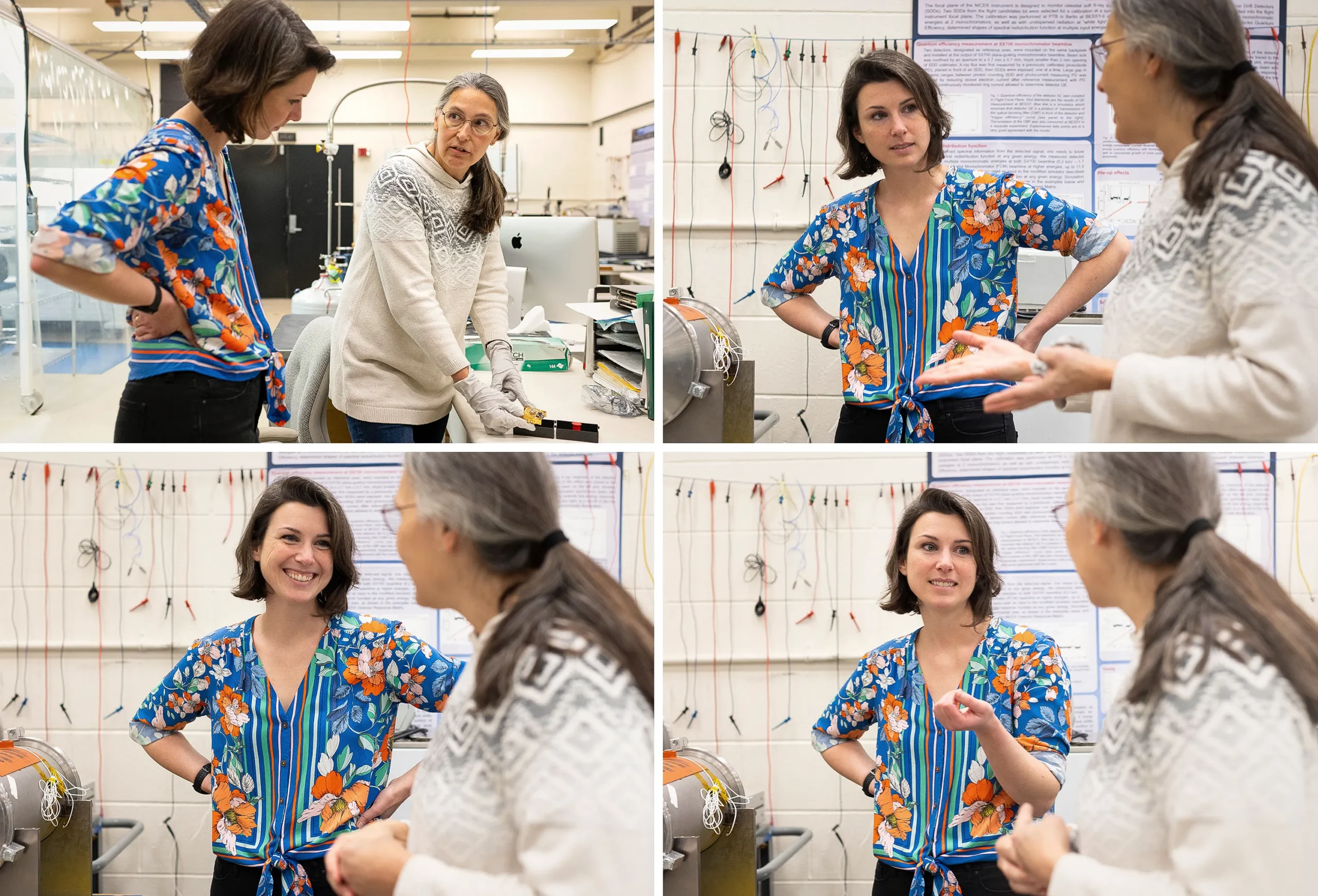
Katherine Taylor for Quanta Magazine
At the big-picture level, what are the questions about black holes that you’re most interested in addressing?
If you understand how gas flows behave in the strongly curved space-time around a black hole, then you can use that information to measure the fundamental properties of the black hole: its mass and its spin, a measure of how fast it’s rotating.
Could you describe the general setting of the black holes you’re looking at?
They’re all surrounded by disks of gas and dust. These accretion disks can get hot enough on their own to emit optical and ultraviolet radiation, but they’re not hot enough to create X-rays. What we see with our telescopes is that accreting black holes — which are in the process of consuming those disks — always produce X-rays. If you see X-rays from a billion light-years away, it’s probably from an accreting supermassive black hole.
How are the black holes creating those X-rays?
We know that there must be some really high-energy plasma around the black hole that’s creating these X-ray photons. We call that the corona — like the hot plasma that’s around the sun. A black hole’s corona is like the crown on top of the accretion disk. It can get extremely hot, and it produces a lot of X-rays. The material in the accretion disk is about a million kelvins, but the corona is a billion kelvins. We don’t actually know a lot about this corona, and one of the big questions is: How does it form? What’s its geometry?
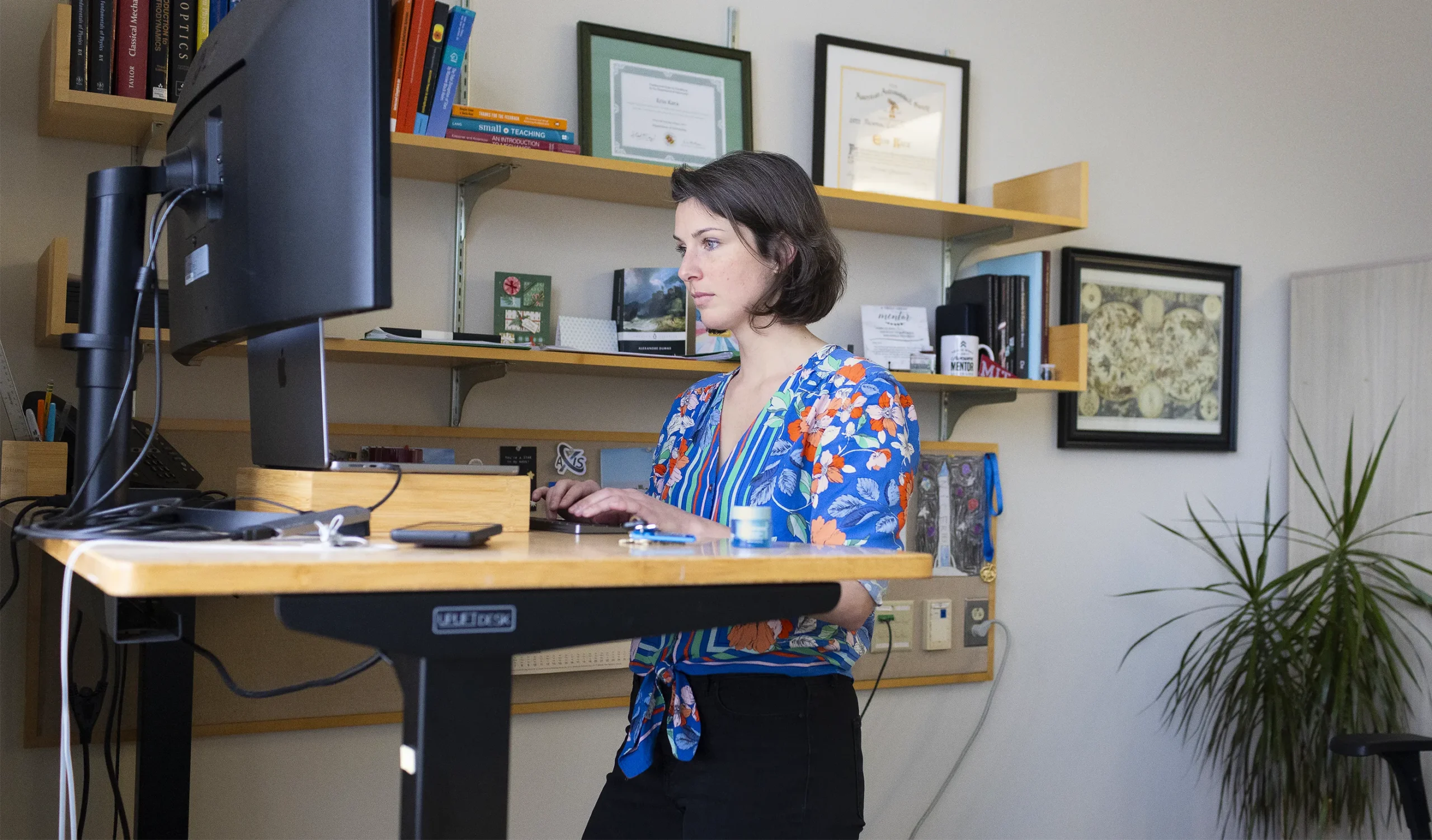
To map the darkest of galactic hearts, Kara uses the X-rays produced by the maelstrom of matter surrounding black holes.
Katherine Taylor for Quanta Magazine
So how can the technique you use, reverberation mapping, go from collecting X-rays to revealing the corona and its surroundings?
You have this corona around a black hole, as well as an accretion disk. And as the corona irradiates that colder accretion disk with X-rays, it causes ions in the disk to radiate X-rays of their own, mainly through fluorescence.
Fundamentally, these secondary X-rays are like echoes of light, so we call them reverberation echoes. What we’re doing is measuring time delays between a primary flash of X-ray light from the corona and the corresponding echoes from the accretion disk. If we can measure those echoes, then we can reconstruct what it looks like around the black hole.
It’s akin to how bats use echolocation. They can’t see the dark cave that they’re flying through, but they know that the echo will come back at them with some delay, and they can use the fact that the echo is traveling at the speed of sound to map out the dark cave. We’re doing that, except with light traveling at the speed of light.
I was about to say, the walls around a bat are not moving at relativistic speeds, so I imagine that things are a little bit dicier when you’re doing this.
[Laughs.] It’s a little bit more complicated than that, just slightly …
What does it take, then, to go from your data — X-ray photons in a bucket — to a map of the region around a black hole?
Originally, we were just looking for a range of X-ray wavelengths that we knew was dominated by emission from the corona, and a range that we knew was dominated by that echo. We found that if you converted the time delay between those ranges into a distance for light to travel, it roughly corresponded to mapping the gas flows very close to the black hole — something within a few times the event horizon’s radius.
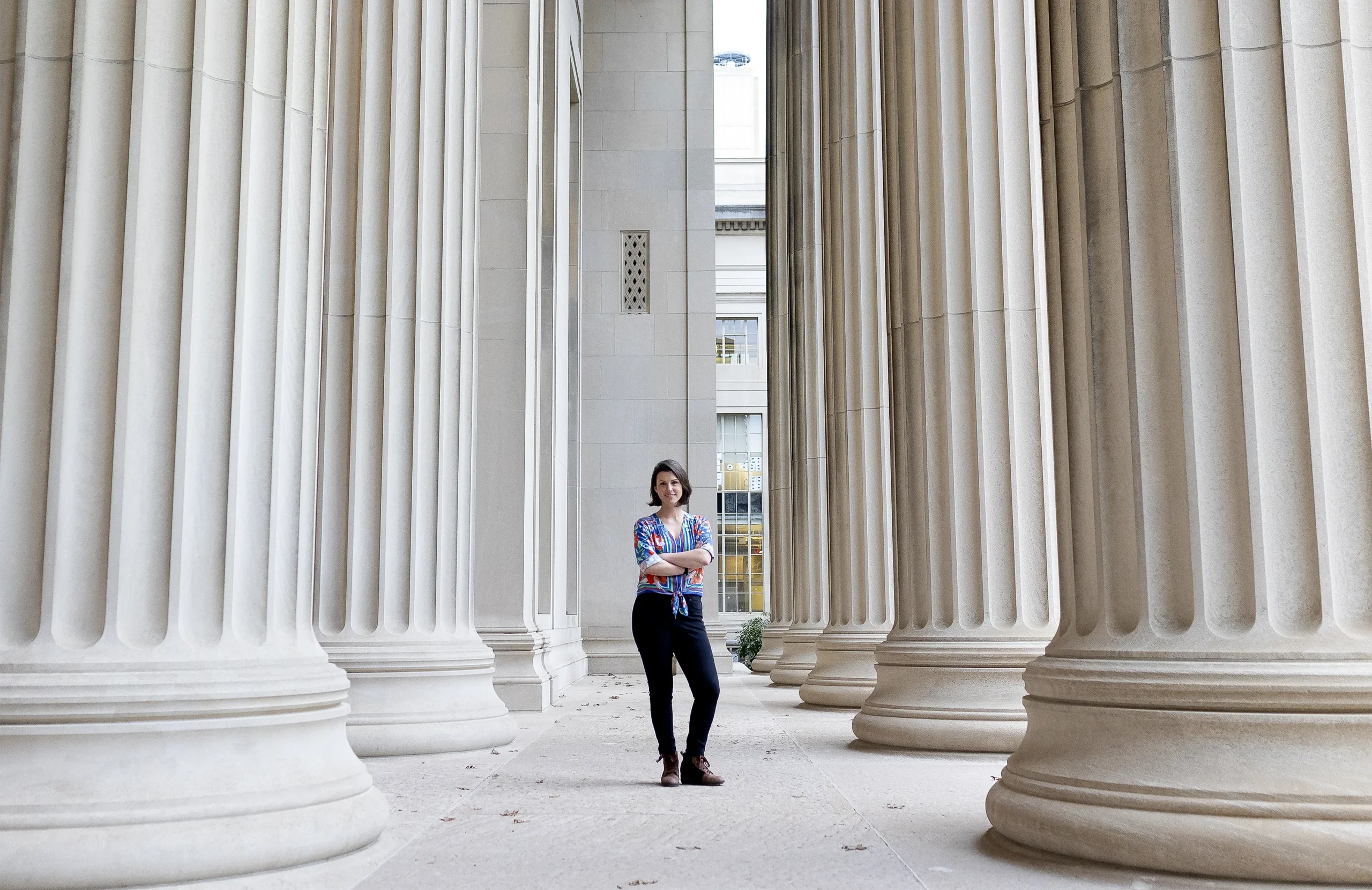
Unlike many of her colleagues, Kara didn’t always know she wanted to be an astrophysicist.
Katherine Taylor for Quanta Magazine
Now we try to create many different simulations of corona-accretion disk systems and then identify the ones that look like the data that we see. We do this with what are called general relativistic ray-tracing simulations. Ray-tracing simulations are used in all sorts of video games, and it’s the same kind of principle: We take a point that’s a model for the corona, we shine light rays from it in all different directions, and we just follow where those light rays go. Some of them will go to a distant observer, and some of them will fall into the disk, irradiate the disk, and then bounce off and reflect into the plane of the telescope.
How does reverberation mapping differ from, say, what the Event Horizon Telescope did to obtain direct images of black holes?
The Event Horizon Telescope has been just amazing for imaging the black holes at the center of our galaxy and M87, but it really can only do those two black holes. More than that, if we want to get at the question of how black holes grow, we need to look at black holes that are actively growing right now — or rather, black holes that are extremely luminous in X-rays.
The black hole at the center of our galaxy must have had this phase where it was actively growing and eating a lot of material. Right now, it’s not doing that — and it’s precisely because it’s not growing very much that the Event Horizon Telescope can image it directly. To study those black holes that are actively growing, where there’s high-density material rushing toward the black hole, we need another technique. And that’s where reverberation mapping comes in.
Are there any specific black holes that you’ve looked at that stand out to you as particularly informative?
My general research tactic is to find black holes that behave in a way we kind of expect. If you can understand the details of those systems, and you can really cleanly trace the geometry around the black hole, then you can make precision measurements of the mass and the spin of the black hole.
One of my favorites right now is an X-ray binary called MAXI J1820+070. It’s not a supermassive black hole; it’s just 10 times the mass of the sun. It’s in a companion system with a star, and it’s pulling gas off of that star and forming an accretion disk and a corona. It’s just a very clean system, and it’s so close to Earth: It’s about a thousand times brighter for us than these supermassive black holes.
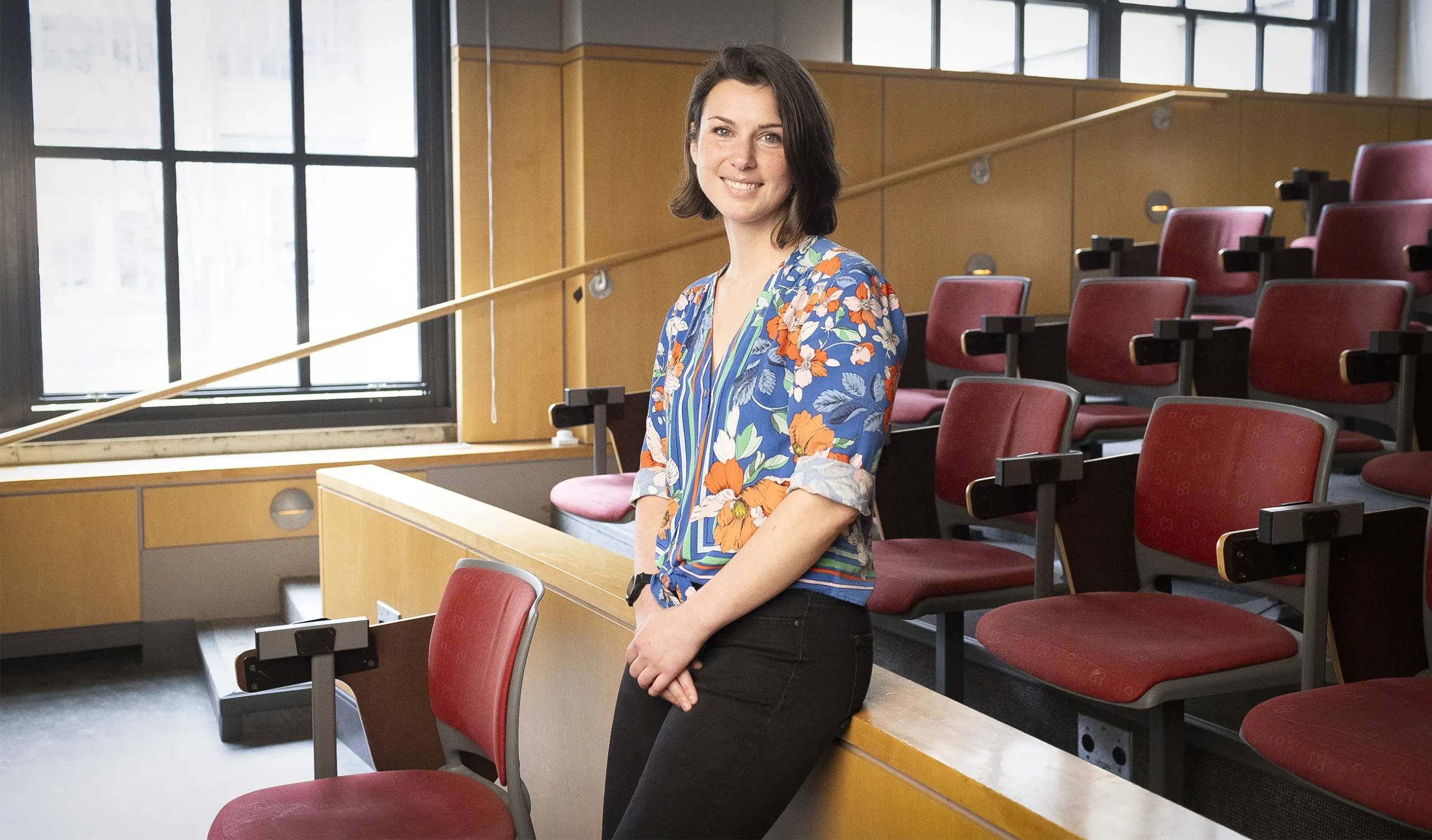
“There’s this stereotype that black holes are nasty things,” Kara said. “But what’s amazing is that black holes actually give us life.”
Katherine Taylor for Quanta Magazine
And on the flip side, which black holes stand out to you as particularly extreme or particularly weird?
I like to study the normal guys, and then I like to crank things up and see where things break. What’s also really fun are the oddball systems that just keep you up at night wondering: How the heck did nature let that happen? And the source that has kept me up at night is called ASASSN-18el, which is a supermassive black hole.
ASASSN-18el was found by the ASAS-SN all-sky survey as one of these black holes that were kind of normal-looking and then, all of a sudden, went into this crazy outburst. We started observing it with optical, UV, and lots of X-ray observations as well, and it just went totally bananas. It had these initial outbursts, and then it turned off — like, a four-orders-of-magnitude decrease in luminosity. And then it turned back on again and became the brightest X-ray source in the extragalactic sky for about a year, and then it began to turn off again. Now it looks like it may be turning back on.
We thought that the timescale for something to turn off by four orders of magnitude would be at least millions of years. And yet we saw it happen in a year — or in a few months. How does something like that happen?
Historically, what we knew about these accreting supermassive black holes — called active galactic nuclei — we learned from surveys where we’d look at them every once in a while. But now we have these all-sky surveys that are scanning the sky a couple of times a week, and we’re just staring at these black holes, seeing what they do. And it turns out that when we weren’t looking at them, they were doing all sorts of crazy things. Now we’re just finally realizing it.
What motivated you to get into astronomy and study black holes, and what keeps you inspired today?
The thing that really got me excited about pursuing astronomy was the discovery aspect: It was just super thrilling to be the first person to look at light that was released from around a black hole a billion years ago. That’s amazing. How is it that we puny human beings can even think about these things, or come together to build technologies to answer some of these huge fundamental questions that people have been asking since the beginning of human thinking?
And I love the community aspect of bringing people together for a common goal. We have this idea about what “science” is — that you were, like, sitting by yourself and inspired by your own creative genius, and you made all of these discoveries on your own. But that’s not what’s super inspiring about it. To me, it’s that we are able to work together and use each other’s expertise to create something so much bigger than any of us.