Diminishing Dark Energy May Evade the ‘Swampland’ of Impossible Universes
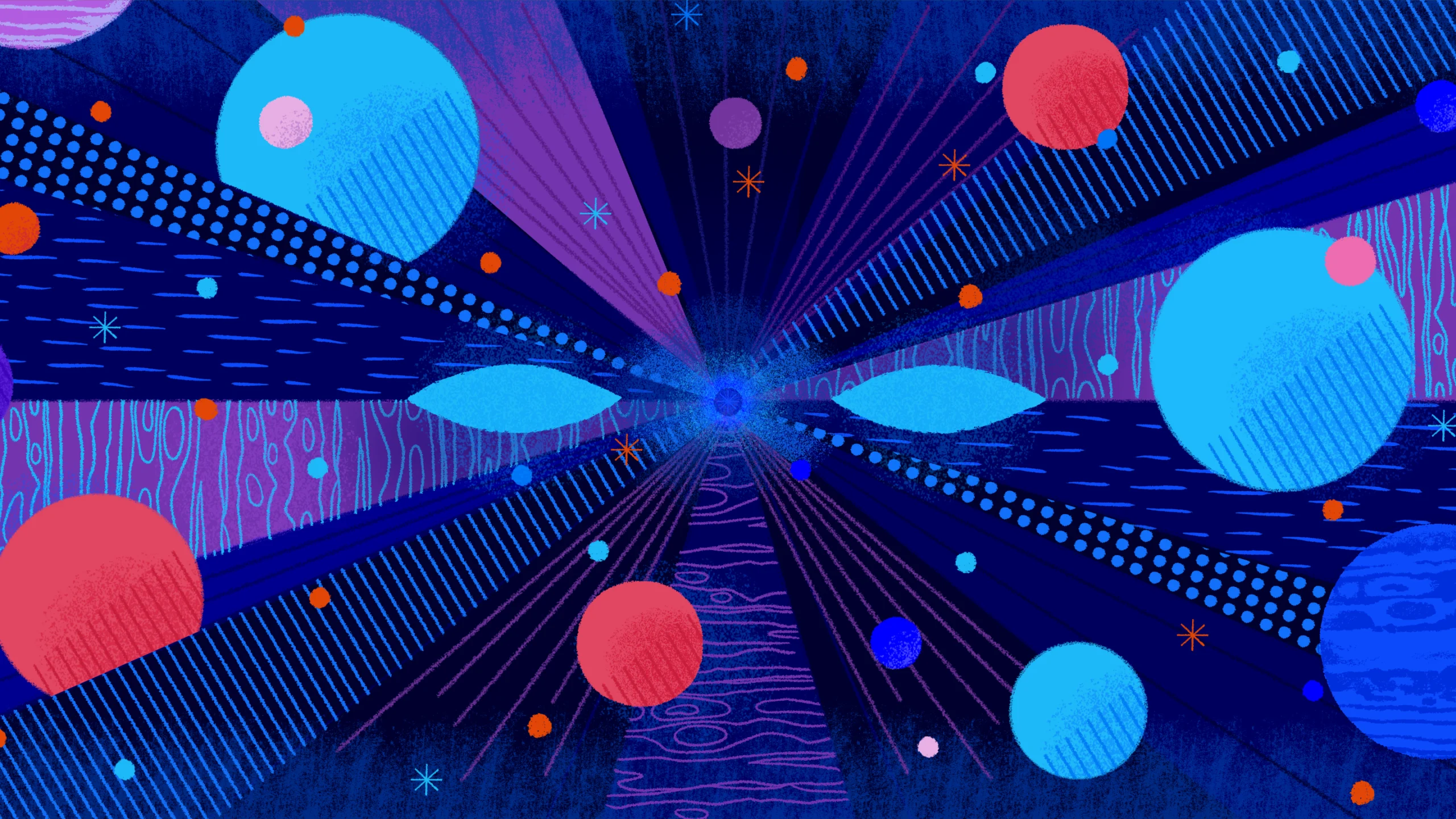
The expansion of the universe is speeding up, but the latest data suggests that the rate of acceleration may be decreasing.
James O’Brien for Quanta Magazine
Introduction
On the morning of April 4, physicists filed into a third-floor meeting room at Harvard University’s Jefferson Laboratory. Word had gotten out that there would be a big announcement from the Dark Energy Spectroscopic Instrument (DESI) collaboration, a group of physicists who are investigating dark energy — a mysterious, repulsive form of energy that permeates the universe. The meeting room at Harvard got so crowded with people wanting to watch the livestream that some sat on the floor. Everyone eventually decided to move to the bigger lecture hall downstairs.
DESI’s announcement lived up to the hype. The group’s results indicated that dark energy, which most physicists have long held to be unchanging, may in fact be weakening.
Since 1998, we’ve known that the expansion of the universe is accelerating. Dark energy is the name given to the accelerant. In the standard theoretical model of the cosmos, dark energy has a simple form: It is spread uniformly in space, maintaining a constant density at all times. Dark energy of this type, known as the cosmological constant, would not get diluted as the universe expands. Rather, as space grows, the amount of dark energy grows with it. And so this accumulating energy expands the universe with ever-increasing gusto.
But the constancy of dark energy is merely a hypothesis, one that the DESI experiment set out to check. The collaboration reported that they had so far mapped and analyzed the locations of 6.4 million galaxies to determine how fast the universe has expanded as a function of time. (More distant galaxies reveal the younger universe.) If dark energy is a cosmological constant, then the acceleration should hold steady. DESI’s data set wasn’t big enough to test this by itself, though the team will be able to do so after mapping a total of 40 million galaxies during the five-year survey. But when the scientists pooled their data with other astronomical observations, the combined data sets favored an evolving dark energy.
The findings fall short of the level of statistical certainty needed to claim a discovery. Right now, DESI is calling it a “hint” that dark energy might be weakening in strength. But according to Nathalie Palanque-Delabrouille, a cosmologist at Lawrence Berkeley National Laboratory and one of the leaders of DESI, “that hint gets stronger as we start combining different data sets. And all these data sets seem to be pointing in the same direction.”
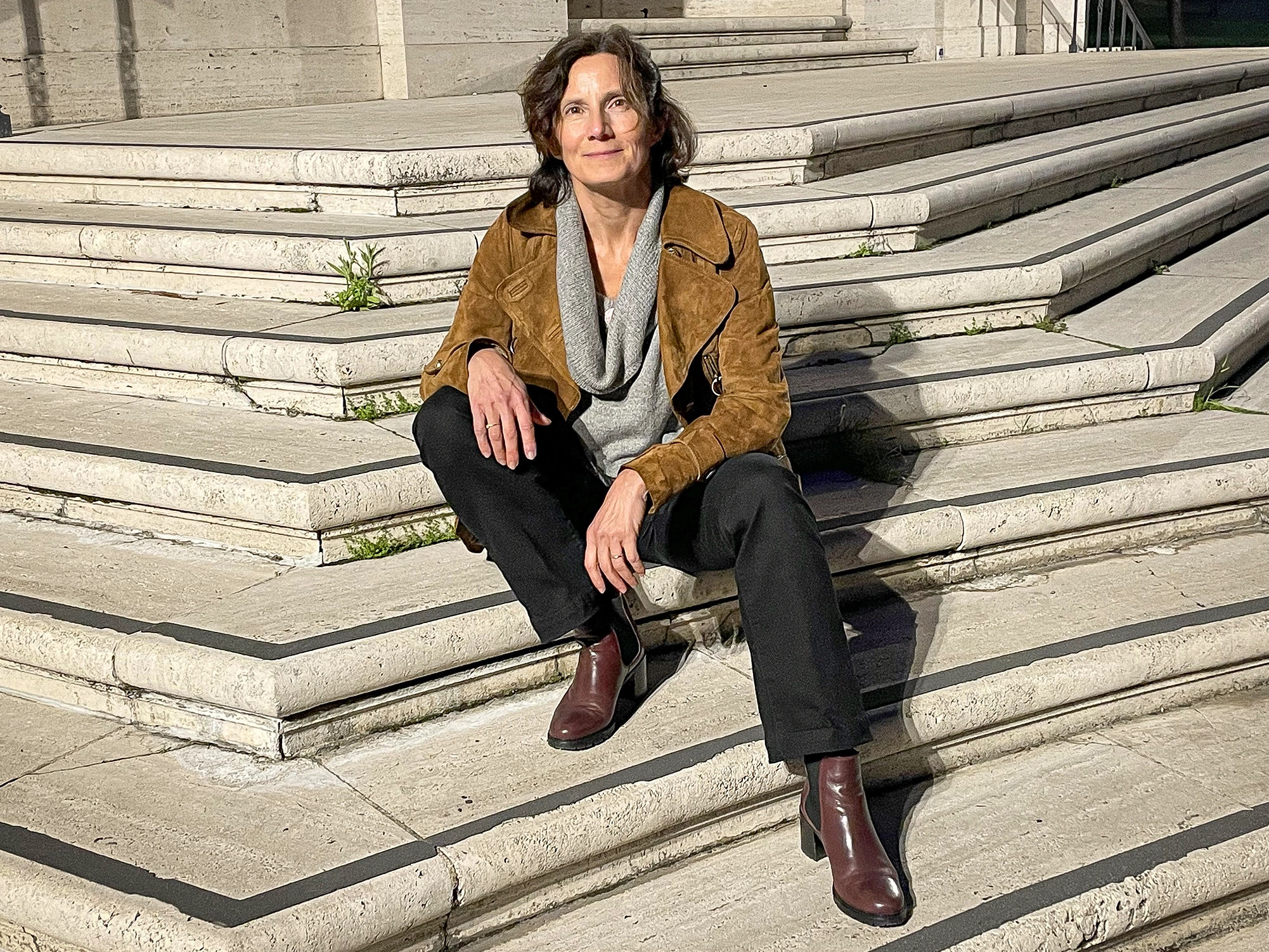
Nathalie Palanque-Delabrouille, a cosmologist at Lawrence Berkeley National Laboratory, is one of the leaders of the DESI team.
Jacques Delabrouille
The measurements will grow increasingly accurate as more data accrues over the next few years.
In the meantime, theorists are not waiting around. DESI’s hint about the nature of dark energy, which comprises 70% of the mass-energy content of the universe, is just too big to sit on. Immediately after the April 4 presentation, the physicists who had gathered at Harvard returned to their blackboards to contemplate the implications of what they’d just heard, as did other researchers worldwide. They drew curves that illustrated where dark energy might be now and where it was heading in the future. “If the data keeps holding up, what does it mean?” asked Alek Bedroya, a physicist who was visiting Harvard from Princeton University at the time of the announcement. “How compatible is the DESI data with our conjectures?”
Part of the intrigue surrounding the DESI news was that Bedroya and some of his colleagues had already boldly hypothesized that dark energy ought to weaken.
Einstein’s Blunder
Befuddlement over dark energy dates back more than a century. In 1917, Albert Einstein introduced the cosmological constant into the equations of his general theory of relativity. He thought that it was necessary to keep his model of the universe static — neither contracting nor expanding — as he believed was the case. Yet Einstein had grave misgivings about the constant, and he removed it from his equations, calling it his “greatest blunder,” shortly after Edwin Hubble’s 1929 discovery that the universe is indeed expanding.
Yet Einstein had been right when he added in his cosmological constant; he just did it for the wrong reasons. In 1984, the physicist James Peebles argued that this repulsive form of energy was needed to counteract gravity and match observations of the universe’s large-scale geometry.
The case for a cosmological constant, or something similar, became airtight in 1998. That year, two independent teams showed that distant supernovas were farther away than expected, indicating that the universe’s expansion is accelerating. No one knew whether the accelerant was Einstein’s cosmological constant or something more complicated, so it was given the vague label “dark energy.”
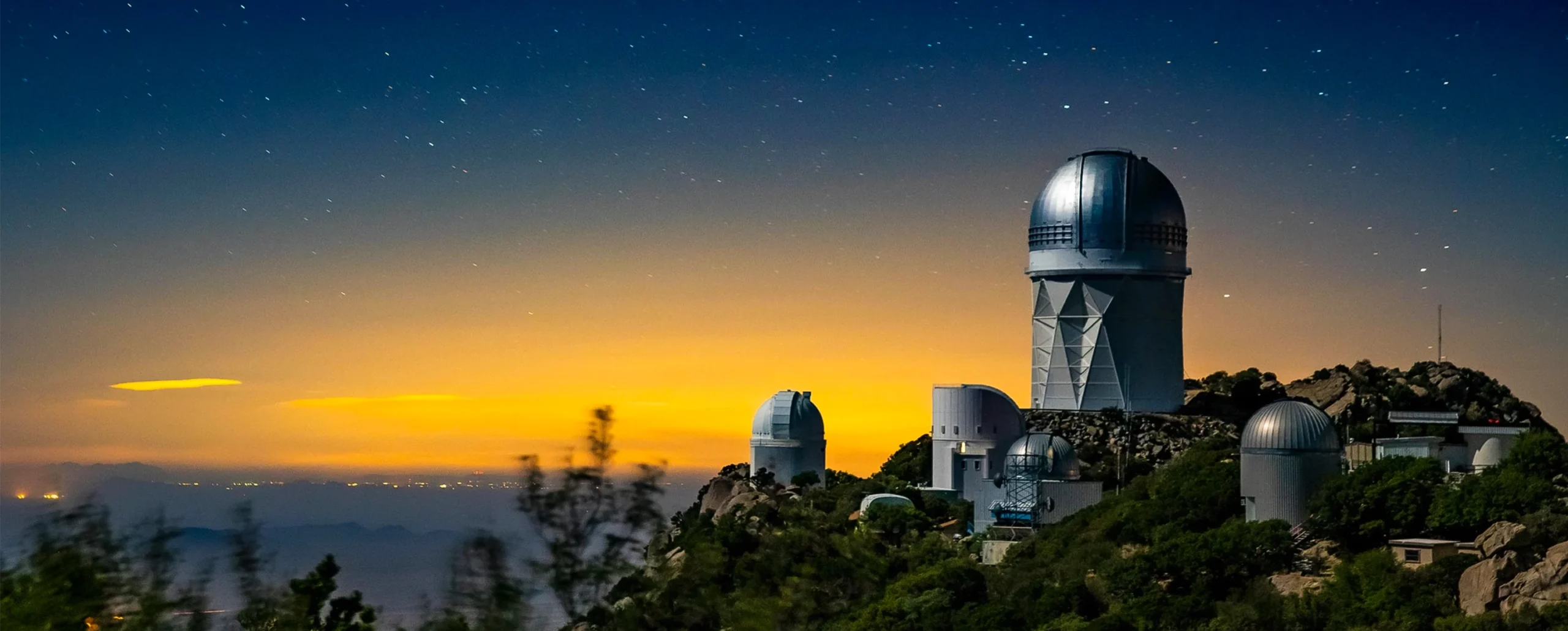
The Dark Energy Spectroscopic Instrument at Kitt Peak National Observatory in Arizona has produced the largest 3D map of our universe to date.
Marilyn Sargent/The Regents of the University of California, Lawrence Berkeley National Laboratory
Whatever it is, its actual density is minuscule — so small that theorists have struggled to explain it. The most straightforward explanation of what the energy is comes from quantum physics, which has long predicted that space should be suffused with substantial amounts of energy. The quantum fields that permeate space fluctuate in strength, never staying exactly at zero; particles continually spring into existence and disappear just as quickly. These quantum fluctuations should contribute to the energy of ambient space. But when physicists do the math, they find that the effect could be as much as 10120 times bigger than the observed abundance of dark energy.
This has been called “the worst theoretical prediction in the history of physics.” The discrepancy has confounded physicists for decades. One possible explanation for the tiny value, advanced by the late Nobel laureate Steven Weinberg, remains one of the best guesses anyone has. Weinberg’s argument rests on the idea that our universe may be part of a larger ensemble of universes now known as the multiverse. He argued that the density of dark energy might range widely throughout this ensemble, perhaps as positive and negative amounts of energy coming from quantum fluctuations cancel each other out to varying degrees. And he calculated that the value we observe in this universe, though minuscule, is about as big as it could be for galaxies to have formed before cosmic expansion stretched all matter apart. Dark energy’s ultra-low density is a necessary condition for the existence of humans who ponder conundrums like this.
Quintessential Dark Energy
The idea that dark energy changes over time dates back to a 1998 paper by the physicists Robert Caldwell, Rahul Dave and Paul Steinhardt. They labeled dark energy of this kind “quintessence.”
Quintessential dark energy could either increase or decrease in density. If it is decreasing, there is a nice mental picture, said Caldwell, a physicist at Dartmouth College. He described the evolution as “like a ball rolling down a hill.” The altitude of the ball reflects the density of dark energy at any given moment. (If dark energy were a cosmological constant, the ball would be stuck on a flat plateau.)
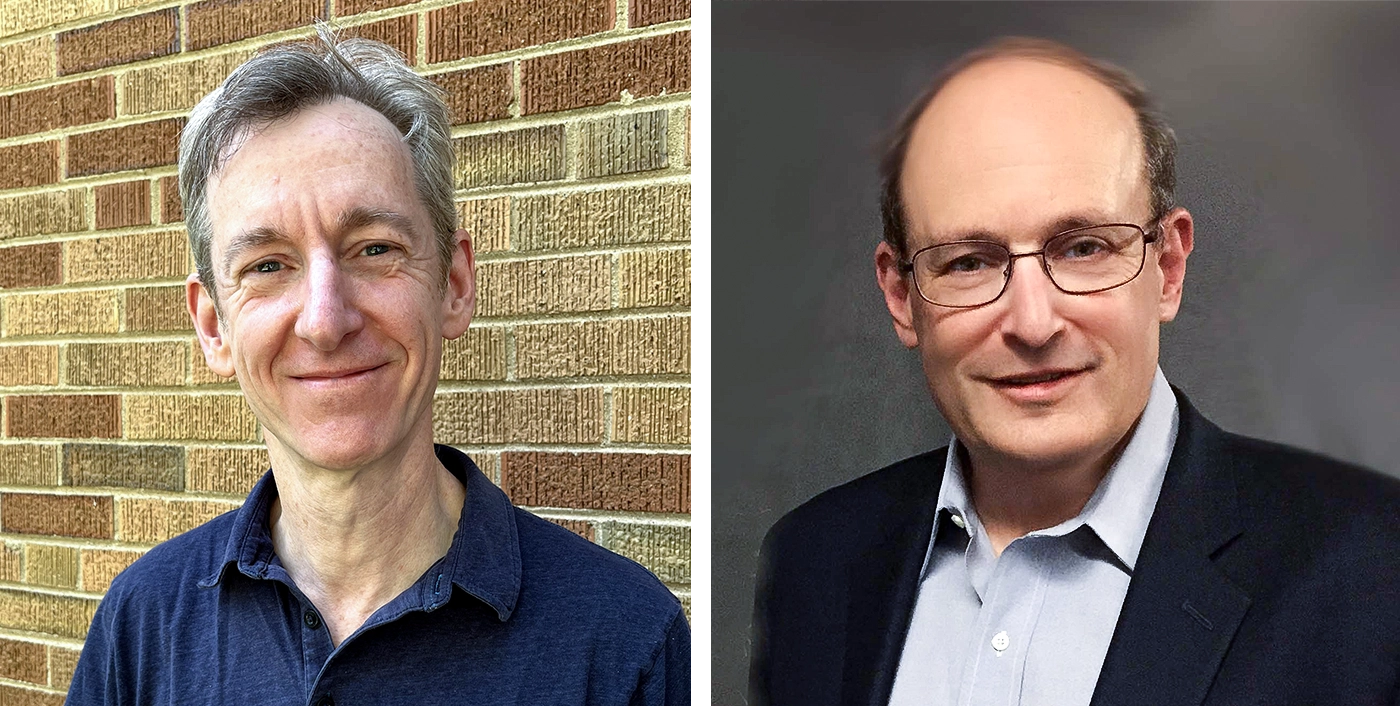
The physicists Robert Caldwell (left) of Dartmouth College, Paul Steinhardt (right) of Princeton University and Rahul Dave proposed a theory of varying dark energy known as quintessence.
From left: Courtesy of Robert Caldwell; Courtesy of Paul Steinhardt
According to this model, dark energy could, in theory, increase as well, but such a situation seems unnatural. The mental picture in this case would be a ball rolling uphill, explained Steinhardt, a physicist at Princeton. It’s “something you don’t normally see,” he said. “It would take a ball or hill with some exotic properties, and maybe some strange physics, for that — uphill rolling — to happen.” Physicists call this situation the “phantom regime.”
Yet, puzzlingly, the DESI team’s April paper suggested that for most of our universe’s history, dark energy sat squarely in the phantom regime. Only recently, according to their analysis, did it cross over into the realm of normal quintessence.
Some cosmologists find that scenario implausible.
A week after DESI released their data, Marina Cortês and Andrew Liddle of the University of Lisbon challenged the phantom dark energy interpretation. Cortês characterized DESI’s data acquisition and processing as “superb” but said, “We think it is much more likely that future data will support the cosmological constant than some weird phantom dark energy model.”
In late April, three physicists proposed a cosmological model that fit the data without ever crossing into the phantom regime. “That, theoretically, is what we would expect from evolving dark energy,” said Kim Berghaus, a physicist at the California Institute of Technology and one of the paper’s authors.
Then, in May, Steinhardt and the Princeton graduate student David Shlivko came to a similar conclusion. “While the DESI group said their data favors phantom dark energy,” Steinhardt said, “we showed you can have a quintessence model with no phantom dark energy that fits the data just as well.”
The notion of quintessential dark energy strikes Steinhardt as eminently plausible — a more natural prospect, even, than a cosmological constant, which he considers “strange because of its constancy. The whole thing that makes energy interesting is that you can transform it from one form to another, and there are backreactions: If you change one thing, it must have effects on other things.” A cosmological constant would be a curious outlier in this regard, Steinhardt said. No other form of energy we know of “is strictly like that — forever constant and unaffected by anything else.”
Dark Energy Meets the Swampland
In fact, Steinhardt and many other physicists were already thinking about this issue when the DESI group made its announcement. Steinhardt was spending the academic year at Harvard as part of the Swampland Initiative, a research program dedicated to identifying the general principles and constraints that any quantum theory of gravity must adhere to. The initiative, which brought together about 100 researchers from around the world, was led by the Harvard physicist Cumrun Vafa, who came up with the swampland idea in 2005.
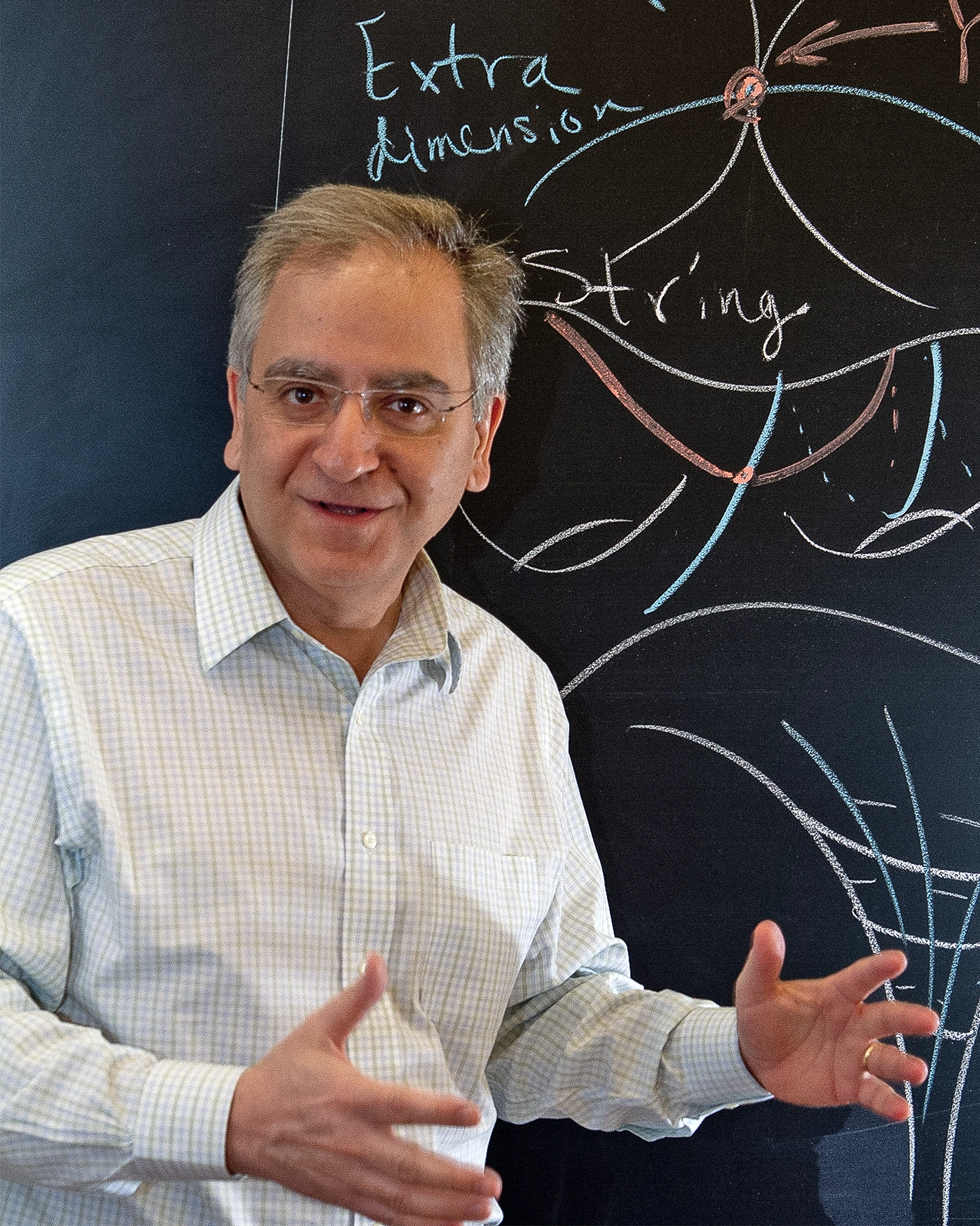
Cumrun Vafa, a theoretical physicist at Harvard University, started the swampland program, an effort to rule out logically inconsistent properties of universes.
Hayward Photography for Quanta Magazine
Since the 1980s, Vafa has made major contributions to string theory, which replaces the pointlike objects of particle physics with tiny strings. The vibrational states of these strings correspond to the different particles and forces of nature, including the force of gravity. The theory posits that strings vibrate in 10 space-time dimensions — four of them big and six too small to see. The geometric arrangement of the six imperceptibly small dimensions influences the amount of dark energy in the four-dimensional macroscopic realm we inhabit. While string theory may or may not describe our world at a fundamental level, it does provide a consistent theoretical model for how quantum mechanics and gravity could come together.
One aspect of string theory, which might be viewed as a deficiency, is that it permits a vast number of possible arrangements of the six tiny dimensions. Each of these unique arrangements corresponds to a universe with distinctive properties. The point of the swampland program, Vafa said, is to study these myriad possibilities — along with thought experiments about black holes and quantum gravity — and try to identify general principles that consistent universes all obey. These possible universes comprise “the landscape.” Hypothetical universes with properties that contradict the general principles are dubbed “the swampland.” Our universe must stand on solid ground, so the physicists try to apply the principles they’ve gleaned from their studies to generate new conjectures about reality itself.
The first swampland-based look at dark energy came out in 2018. In it, Vafa and three co-authors found that none of the reliable solutions to string theory they could come up with corresponding to universes with positive cosmological constants were stable. That means the density of dark energy “always wants to fall down,” Vafa said. That’s in keeping with the natural tendency of physical systems to move to a lower energy. He and his colleagues derived a formula that specified exactly how fast the density of dark energy should fall — a formula that, they argued, any consistent universe should follow.
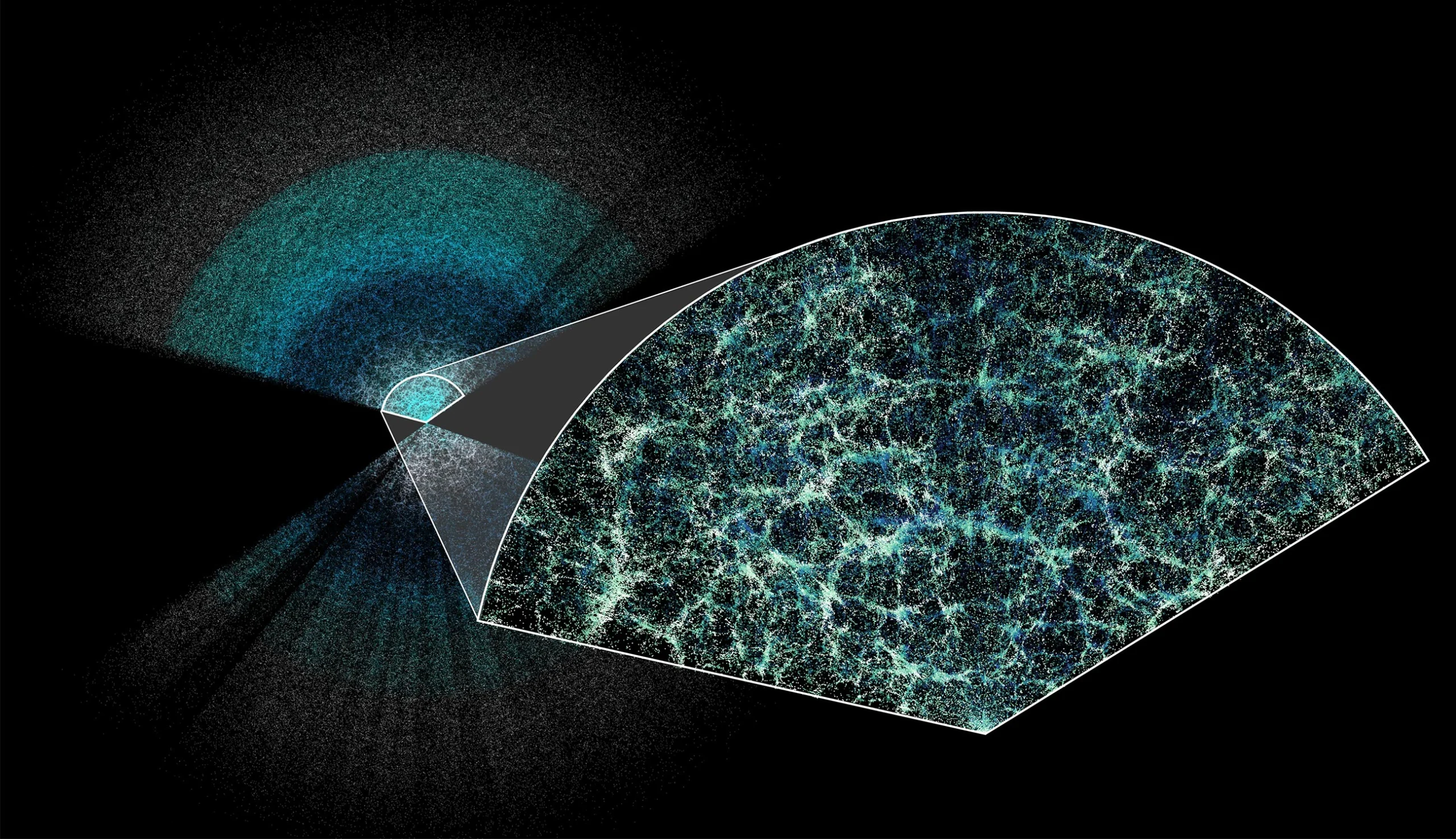
Earth is at the center of this thin slice of DESI’s 3D map of the universe. Magnification reveals the distribution of galaxies and voids.
DESI SkySlice
At the time, the work seemed to conflict with what physicists thought they knew about the universe, with its presumed cosmological constant. And some string theorists still argue that stable universes with positive cosmological constants can exist in string theory; one attempt at constructing such a solution appeared earlier this month. Nevertheless, in light of DESI’s “hint” that the dark energy density is indeed dropping, the conjecture from Vafa and his co-authors now looks prescient.
Trans-Planckian Censorship
There’s another thought experiment that calls into question the very idea of an eternally unchanging cosmological constant.
Let’s say that the expansion of the universe accelerates at a constant rate. Eventually, the expansion gets so fast that even really small things instantly blow up.
In particular, we can consider the smallest distance imaginable — the “Planck length,” which is thought to be the smallest spatial increment that’s observable even in principle. The opposite end of the cosmic ruler is the Hubble horizon, the distance to the edge of the observable universe, beyond which we cannot see. In a universe with a positive cosmological constant, it’s only a matter of time — a lot of time — before the Planck length should grow to the size of the Hubble horizon. According to the trans-Planckian censorship conjecture (TCC), formulated by Bedroya and Vafa in 2019 and updated last year, this should never happen.
It’s a commonsense kind of statement, Bedroya said. “Something that is sub-Planckian” — and hence fundamentally unobservable — “shouldn’t get to the scale where we can observe it.” Nor should it get to a scale that is too big for us to observe it. “The smallest length scale that makes sense in your theory,” he said, “shouldn’t stretch out to be bigger than the biggest length scales that make sense in your theory.”
Assuming that dark energy is the cosmological constant, a straightforward calculation can tell you how long cosmic expansion would have to keep accelerating before the Planck length would start stretching beyond the Hubble length. That amount of time, Bedroya and Vafa computed, is at most 2 trillion years. Dark energy could not behave like a cosmological constant beyond that point.
The TCC conjecture allows for two different scenarios. Dark energy could experience a slow, steady decline. The other possibility is that dark energy holds steady for a while, like a ball stuck in a dip or valley, midway down the hill. But in an instant — occurring somewhere between 10 billion and 1 trillion or so years from now — that will change. In a process called quantum tunneling, the ball will blast through the mound that has been confining it and immediately start rolling down the hill.
If DESI’s preliminary result is confirmed, that would align with option 1.
For Steinhardt, the allure of the swampland endeavor is how it draws on different ideas to make testable predictions about how the universe must be. We’re now entering the regime of measurement, he noted, “where we can actually test these predictions. If they are correct, we should see clear signs of time-varying dark energy very soon.”
‘Biggest Problem in the Universe’
Will DESI’s hint hold up? The team has already collected two more years’ worth of galaxy location data, and an analysis of this larger data set, according to Palanque-Delabrouille, could be out by spring.
Until then, many cosmologists are reserving judgment. “Based on the evidence that we have now, I am not willing to discard the [constant dark energy] model just yet,” said Licia Verde, a cosmologist at the University of Barcelona and a DESI member.
But if the initial DESI finding is confirmed, it will tell us something crucial about dark energy and its future. “Even more importantly,” Vafa said, “we can deduce that this is marking the beginning of the end of the universe. By ‘end,’ I don’t mean nothing happens after that. I’m saying something else happens that is very different from what we have now.” Perhaps dark energy will fall until it settles into a stabler, possibly negative value. With that, a new universe, with new laws, particles and forces, would replace the current one.
“We’re desperate for a clue,” Caldwell said. Dark energy is “literally the biggest problem in the universe.”