What Could Explain the Gallium Anomaly?
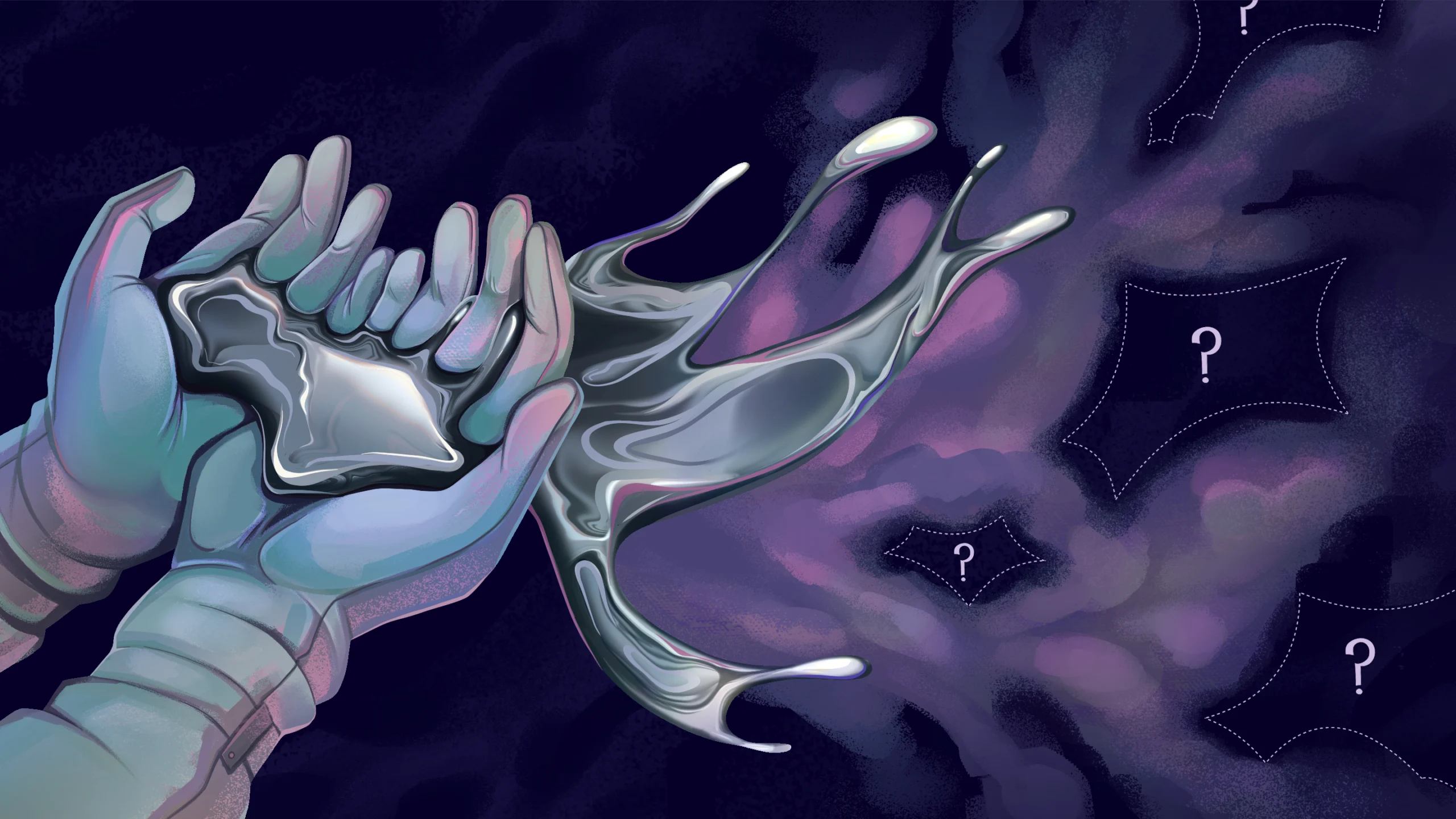
Gallium occasionally converts into germanium, but not as often as expected.
Nico Roper/Quanta Magazine
Introduction
Deep in the Caucasus Mountains, on the border between Russia and Georgia, an unusual experiment is taking place. In an underground lab shielded by a mountain of rock, highly radioactive material sits inside a vat of liquid gallium, blasting out particles called neutrinos that break the gallium down into atoms of germanium.
The goal is to resolve a little-known mystery of physics: the gallium anomaly. “I think it’s one of the most compelling anomalies in neutrino physics that we have today,” said Ben Jones, a neutrino physicist at the University of Texas, Arlington. Some three decades ago, in a previous version of the current experiment, scientists first detected a dearth of the expected germanium atoms that still can’t be explained.
Since then, physicists have worked to rule out possible mismeasurements or inaccuracies that could explain the anomaly. Now they’ve eliminated another one. Eric Norman, a nuclear physicist at the University of California, Berkeley, and colleagues have announced that one possible solution, an incorrect calculation of the half-life of germanium, can’t be the cause.
“The half-life is correct,” Norman said. “This is not the explanation for the gallium anomaly.”
That leaves few possibilities. One is that some still-unknown experimental defect caused the anomaly. Perhaps a different mismeasurement is throwing things off, or a misunderstanding of nuclear physics. Or maybe, just maybe, the anomaly points to a monumental discovery, the existence of a new type of elementary particle called a sterile neutrino. Sterile neutrinos were initially proposed to explain why the masses of the three known neutrinos are so tiny, but they could also account for at least some of the invisible “dark matter” that fills the cosmos.
“We cannot find some huge uncertainty in our experimental procedures,” said Vladislav Barinov, a particle physicist at the Institute for Nuclear Research of the Russian Academy of Sciences who works on the experiment in the Caucasus. “Is it a new type of neutrino? We don’t know.”
Neutrino Village
At the height of the Cold War, before the fall of the Berlin Wall in 1989 and the subsequent dissolution of the Soviet Union, an unlikely partnership arose in the form of an experiment called SAGE, the Soviet-American Gallium Experiment. “The Soviet Union had a phenomenal group of theoretical scientists,” said Steven Elliott, a nuclear physicist at Los Alamos National Laboratory who worked on the project. But they lacked money and access to certain technologies that would make SAGE possible, he said. “Los Alamos was able to provide those types of resources.”
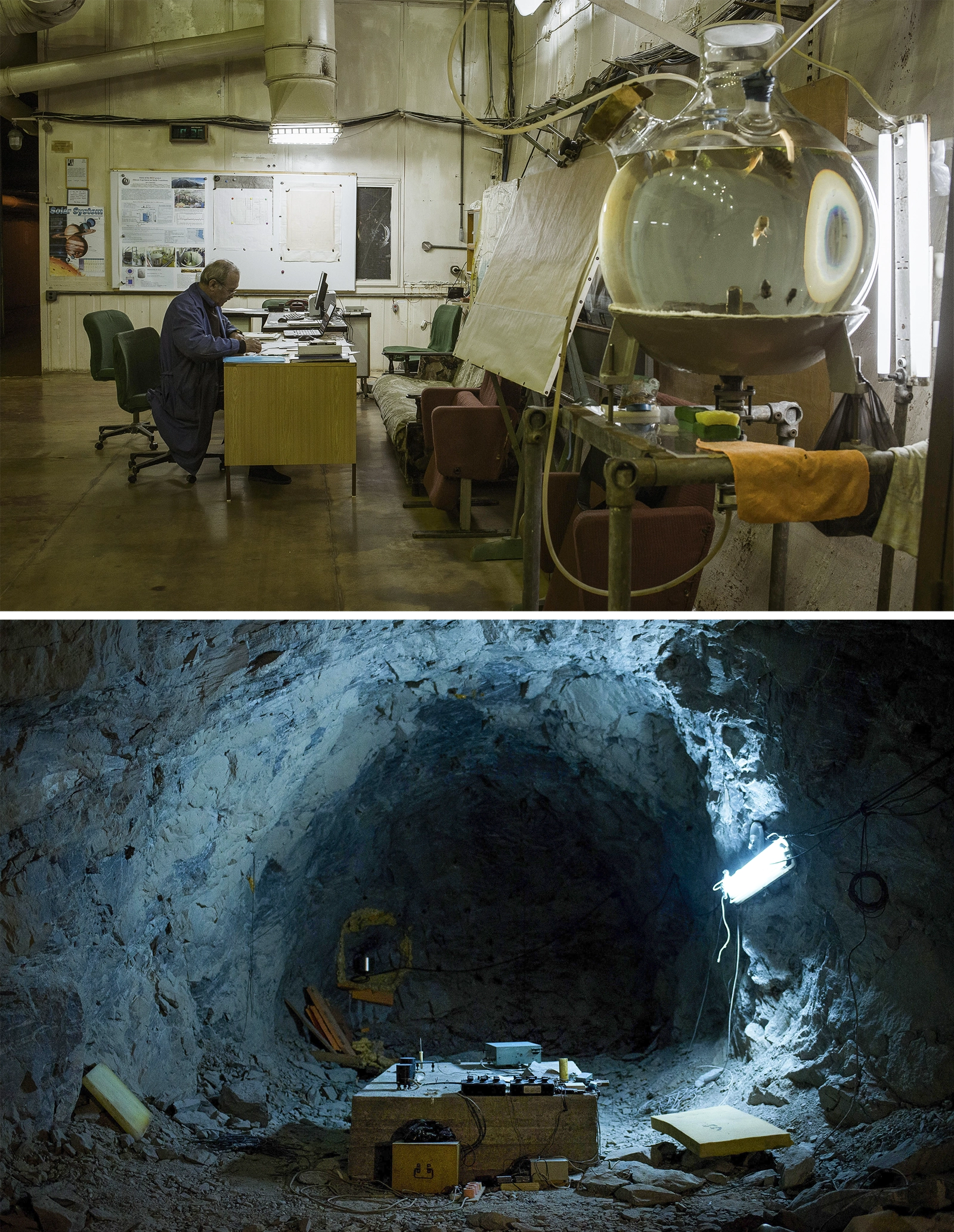
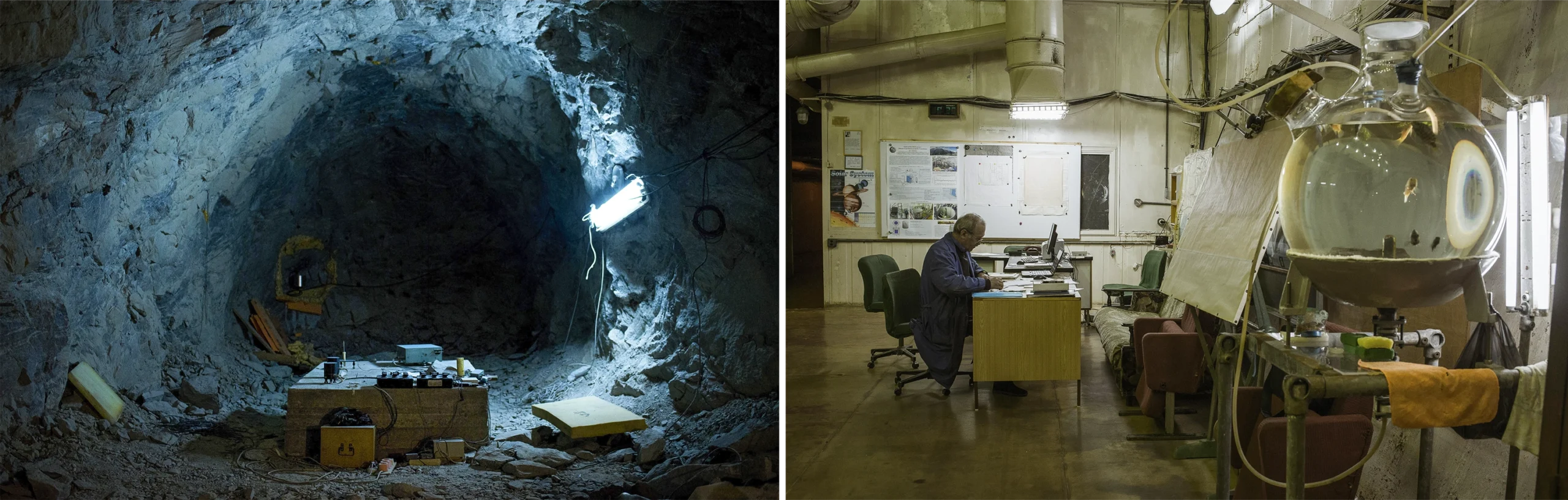
For the last half-century, physicists have studied neutrinos in experiments deep underground at the Baksan Neutrino Observatory in Russia’s Caucasus Mountains. In the lab that houses the BEST experiment, fish serve as an early warning system about any leaking radiation.
Maxim Babenko/The New York Times
SAGE was constructed at the Baksan Neutrino Observatory, a neutrino physics facility built in the 1960s and 1970s inside a mountain in Russia’s Baksan Valley, about 3 miles from the Georgian border. The 13,000-foot-tall Mount Andyrchi shielded the facility from cosmic rays and other sources of noise, allowing precise neutrino experiments to take place.
A nearby residential area called Neutrino Village housed the families of the scientists who worked at the facility, as well as visiting international scientists like Elliott. “I did go out for a number of trips,” he said. “I found it an adventure.”
SAGE began in 1989 and continued for more than 20 years despite attempts by the Russian government to sell its gallium, a precious metal that’s liquid at room temperature. The project was designed to investigate the solar neutrino problem, a measured deficit of neutrinos streaming from the sun. Specifically, scientists were finding a shortage of electron neutrinos, one of three known types, or “flavors.” That problem was ultimately resolved in the 2000s with the Nobel Prize-winning discovery that neutrinos oscillate between flavors as they travel. By the time many of the electron neutrinos from the sun reach Earth, they have become something else.
SAGE used a tank of 57 metric tons of gallium. Incoming electron neutrinos would occasionally combine with a neutron inside a gallium atom and convert it into a proton, turning the gallium into germanium. The scientists counted the germanium atoms in a monthlong extraction process. They chose gallium for the experiment because it has a “low threshold for this reaction,” Elliott said. A similar experiment began in Italy in 1991, called Gallex.
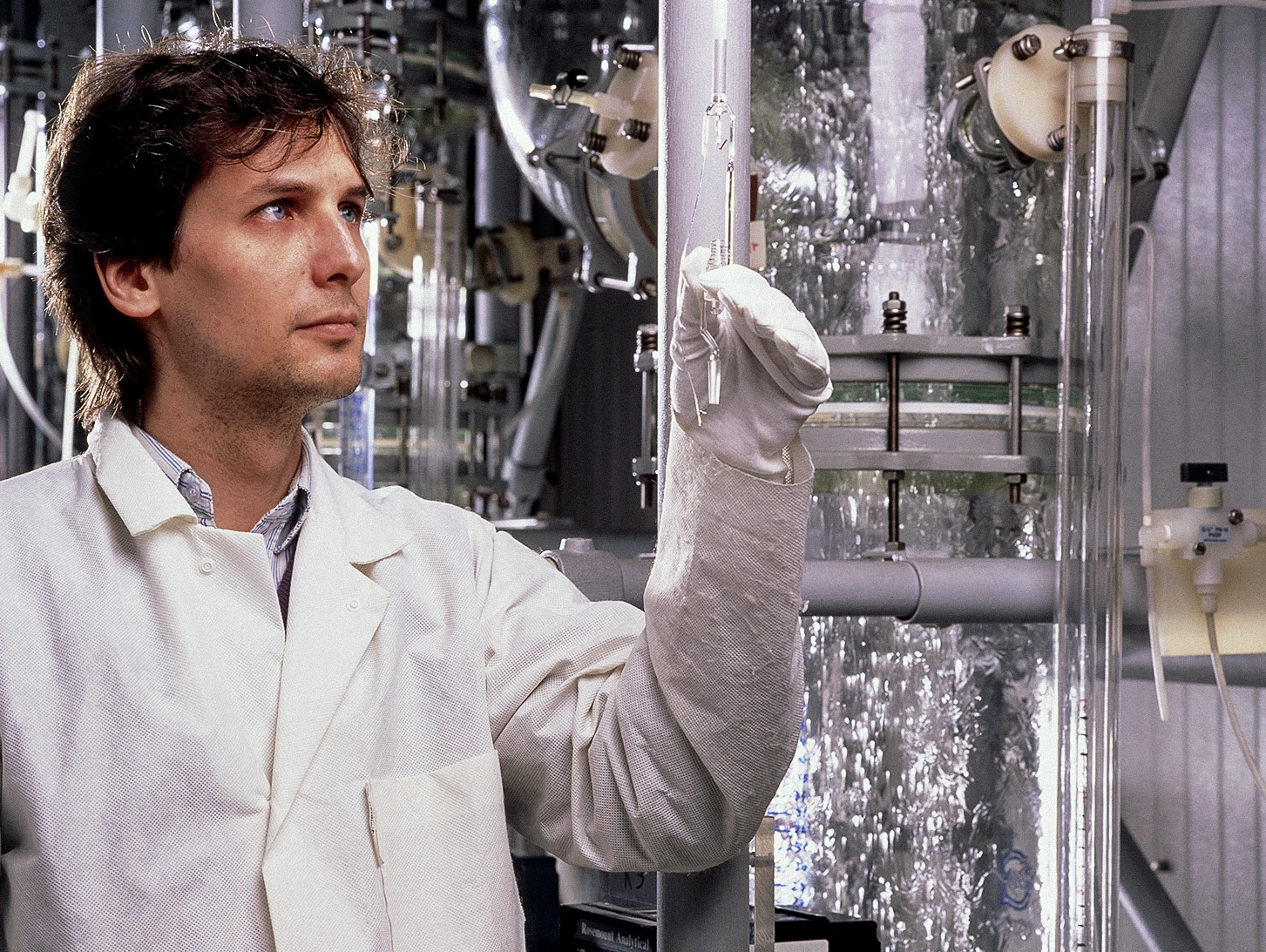
A researcher with the Gallex experiment, which ran in the 1990s at Gran Sasso National Laboratory in Italy, is shown holding a device called a proportional counter that was used for detecting germanium atoms.
Tommaso Guicciardini/INFN/ScienceSource
In the mid-1990s, researchers tweaked both experiments to use neutrinos from radioactive elements. They hoped to avoid unknown errors related to the solar neutrino problem. But both experiments generated roughly 20% less germanium than expected — surprise results that couldn’t have been caused by the solar neutrino problem. “They exactly knew the source activity and how many neutrinos are produced,” said Inwook Kim, a nuclear physicist at Los Alamos. Soon, the puzzling discrepancy had a name: the gallium anomaly. “It was really surprising,” Barinov said.
A follow-up experiment that began at Baksan in 2014, called the Baksan Experiment on Sterile Transitions (BEST), uses two gallium chambers instead of one, to determine whether the anomaly could be explained by the distance from the source of the neutrinos. “BEST was constructed to resolve this tension,” said Barinov, who has worked on the experiment since 2015. But both chambers have continued to show a shortfall relative to what models predict. “It’s a really unusual result,” he said.
Half-Life Theory
Repeated results from BEST continue to show the anomaly as recently as 2022. One chamber contained only 79% of the expected amount of germanium, the other only 77%. “Everybody was hoping that anomaly would go away,” said Wick Haxton, a theoretical physicist at Berkeley. “There is still not any clean understanding of what’s going on.”
A possible explanation was floated: that the half-life of germanium-71 (the specific isotope produced in the experiment), measured in 1985 to be 11.43 days, was actually longer. The same constant controls germanium-71’s decay rate and the rate at which gallium captures neutrinos to produce that germanium. That means a longer germanium-71 half-life would imply a lower rate of neutrino capture and hence germanium production, which could explain the lack of germanium seen by SAGE, Gallex and BEST.
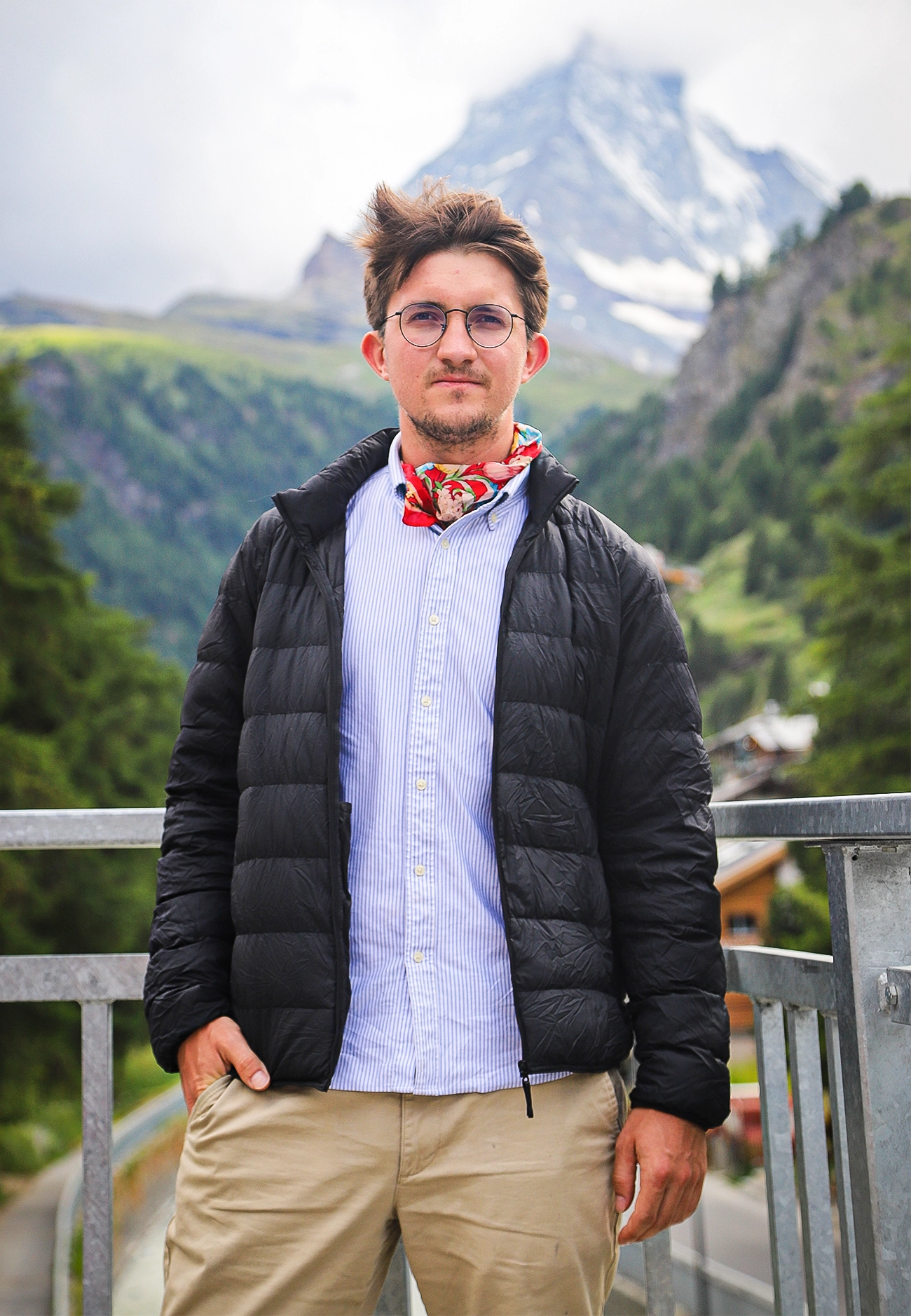
Vladislav Barinov, a particle physicist at the Institute for Nuclear Research of the Russian Academy of Sciences, is part of the team that reported the anomalous results of the BEST experiment.
Sviatoslav Borisov
Norman and colleagues published a reinvestigation of this half-life in Physical Review C in late May. Using a nuclear reactor at the McClellan Nuclear Research Center at the University of California, Davis, they irradiated “very pure germanium material,” Norman said, producing germanium-71. They then analyzed the samples over 80 days to see how long it took the atoms to decay.
They arrived at a half-life of 11.468 days, extremely close to the 1985 measurement, ruling the half-life out as the explanation for the gallium anomaly. While no one ever quite believed the original half-life measurement to be wildly incorrect, researchers still considered it worth checking. “It was a measurement that needed to be done,” Jones said.
Another proposed explanation was that physicists had miscalculated the probability of neutrinos from the source interacting with the gallium. But in September 2023, Haxton and his colleagues also ruled out this possibility. “You can’t get rid of the anomaly,” he said.
That leaves physicists in an uncomfortable position. Either there is still some error that no one has thought of, or, as Haxton put it, “something unusual is going on with neutrinos.” For instance, the experiments might point to a controversial additional type of neutrino, undetected by most other experiments, that might also help to explain dark matter.
Sterile Neutrinos
The three known flavors of neutrinos, which are all millions of times lighter than electrons, interact with other elementary particles via the weak force, which makes them detectable. Sterile neutrinos, on the other hand, would interact only via gravity. If they’re much heavier than the known neutrinos, their existence could explain why the known neutrinos are so light, through an inverse relationship hypothesized around 1980 called the seesaw mechanism.
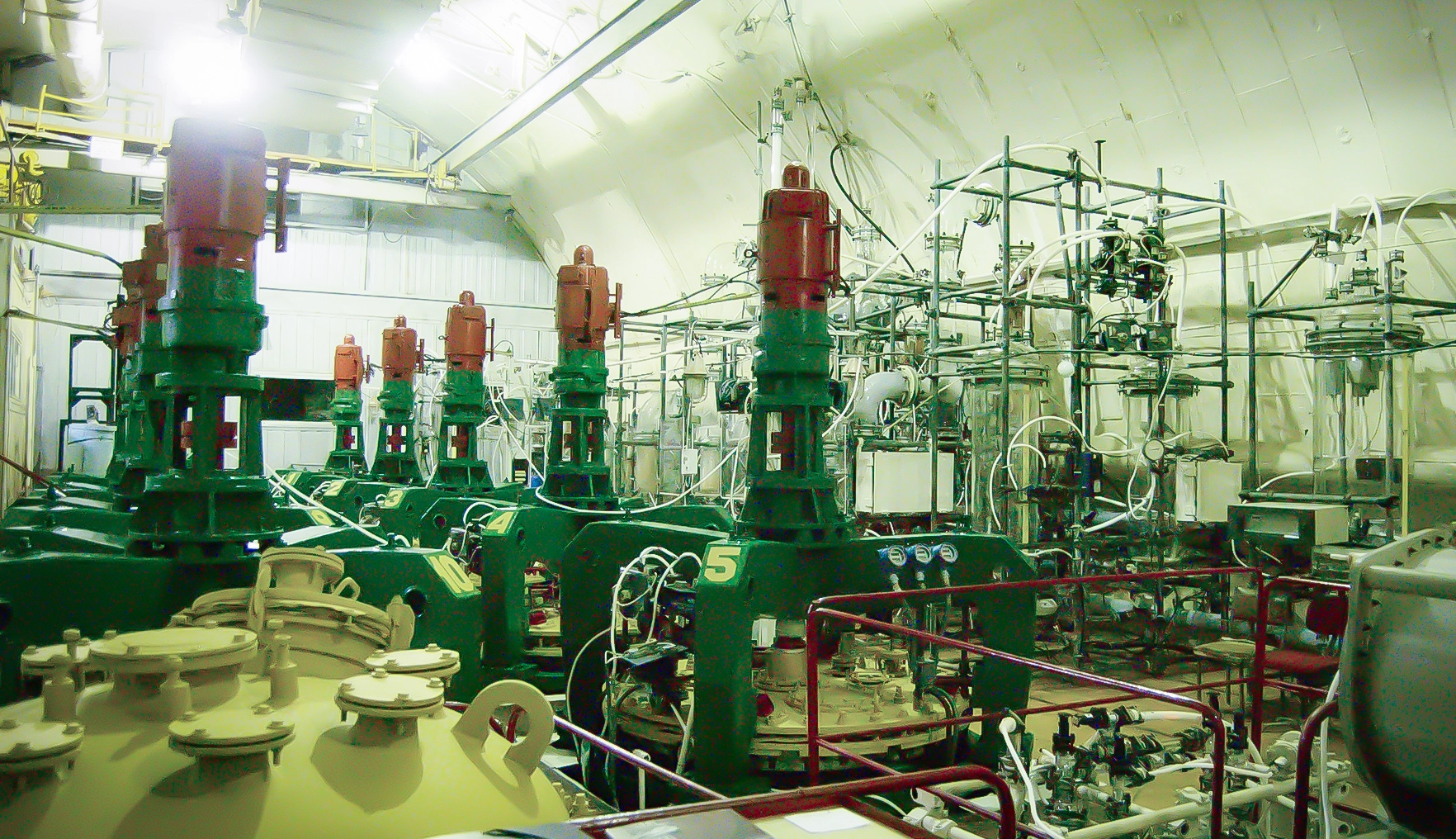
The experimental apparatus used in the SAGE and BEST experiments at the Baksan Neutrino Observatory.
Konstantin Malanchev
The gallium anomaly, however, would point toward a lighter-weight sterile neutrino, with the electron neutrinos emitted by the radioactive source sometimes oscillating into a sterile neutrino that wouldn’t interact with the gallium.
In some models, lightweight sterile neutrinos could comprise a fraction of the universe’s dark matter, though not all of it because they would be too light to gravitationally shape the universe in the way dark matter does. “They could be a small subset of it,” said Lindley Winslow, an experimental nuclear and particle physicist at the Massachusetts Institute of Technology.
Other attempts to find sterile neutrinos by studying neutrino oscillation patterns, however, have been largely unsuccessful. The number of researchers who support the light sterile neutrino “is sort of shrinking,” Winslow said. Kevork Abazajian, an astrophysicist at the University of California, Irvine, said they are the “underdogs of the particle physics community.”
If they do exist, light sterile neutrinos will “wreak havoc” on our current understanding of cosmology, Abazajian said, including ideas of how atoms formed in the minutes following the Big Bang and the theory of the cosmic microwave background, the remnant heat from the initial expansion of the universe. “You would expect to see the presence of this extra neutrino,” Abazajian said. However, he added that recent work has shown that alternative models of the sequence of events in those first minutes “can accommodate light sterile neutrinos.”
In lieu of other explanations for the gallium anomaly, light sterile neutrinos remain a possibility that we just can’t eradicate. “I’ve been a bit skeptical of the sterile neutrino hypothesis, but I can’t tell you why it’s not right,” Elliott said. “There’s never been a convincing explanation of why the experiment might be wrong.”
While Russia’s invasion of Ukraine “has complicated things,” Elliott said, the collaboration between the U.S. and Russia on BEST is still ongoing, for now. Barinov says the team at Baksan is considering using a new source of neutrinos, such as zinc, to further test the result. They may even construct a third chamber of gallium around the source. For now, the anomaly remains unsolved, with no sign of a resolution on the horizon. “It has us all puzzled,” Haxton said.