What Is Life?
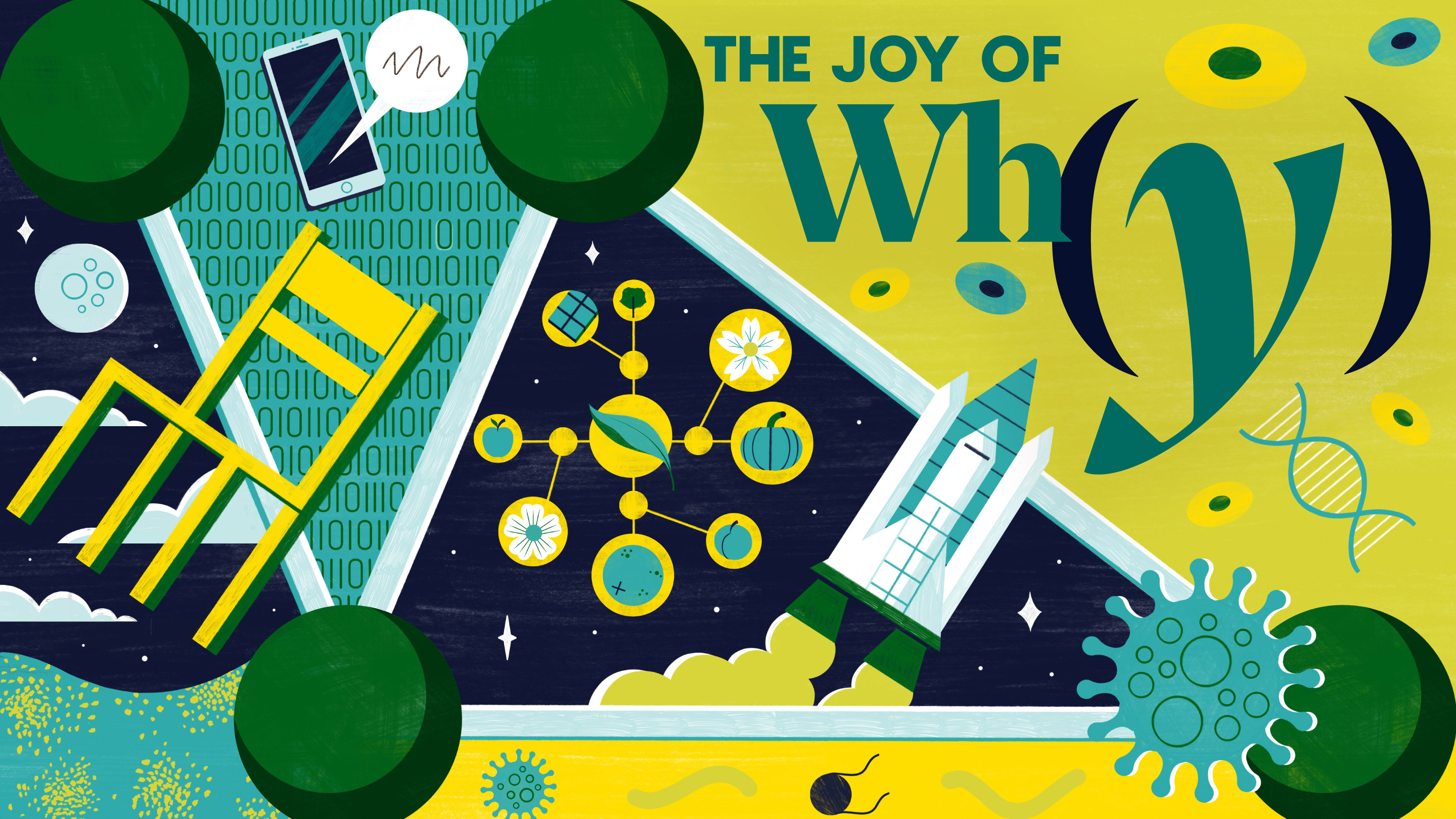
Michael Driver for Quanta Magazine
Introduction
Scientists don’t really agree on a definition for life. We may recognize life instinctively most of the time, but any time we try to nail it down with set criteria, some stubborn counterexample spoils the effort. Still, can we really search for life on other worlds, or understand the earliest stages of life on this planet, if we don’t know what to look for? On this episode, Steven Strogatz speaks with Robert Hazen, a mineralogist, astrobiologist and senior staff scientist at the Carnegie Institution’s Earth and Planets Laboratory, along with Sheref Mansy, professor of chemistry at the University of Alberta, to learn more about how new taxonomies and a “cellular Turing test” might help us answer this essential question.
Listen on Apple Podcasts, Spotify, Google Podcasts, Stitcher, TuneIn or your favorite podcasting app, or you can stream it from Quanta.
Transcript
Steven Strogatz (00:02): I’m Steve Strogatz, and this is The Joy of Why, a podcast from Quanta Magazine that takes you into some of the biggest unanswered questions in math and science today.
In this episode, we’re going to be talking about what it means to be alive. What is life? Can you define it? Scientists don’t actually agree on a definition. It sounds weird, right? I mean, most of us would say with some confidence that a bird is alive and a chair is not. But going deeper, scientists ask questions like this: To be considered alive, does something have to be able to reproduce? Does it have to be a product of evolution through natural selection? Does it need to have a metabolism, and be able to process energy?
(00:51) Any definition along these lines is riddled with exceptions. For instance, is a virus alive? Well, viruses do evolve, but they don’t replicate on their own. They use the host’s cellular machinery to make more copies of themselves. The question of what life is also matters, because if we’re going to be looking for life on other planets, don’t we need to at least have some idea of what we’re looking for?
(01:15) Later on in this episode, we’ll hear from Sheref Mansy, professor of chemistry in the department of chemistry at the University of Alberta. But first, joining me now is Robert Hazen. He’s a mineralogist, astrobiologist and senior staff scientist at the Carnegie Institution’s Earth and Planets Laboratory. Bob, thanks so much for joining us today.
Robert Hazen (01:38): Oh, it’s a pleasure. Thanks so much, Steve.
Strogatz (01:40): Great. Well, let’s jump right into this. Why is it so hard for scientists to agree on something that, common-sensically, most people would say they already understand? Like, we know that a plant is alive and a rock is not. Why is it so hard to come to some agreement about the definition of life?
Hazen (01:57): Yeah, that seems strange, doesn’t it? Because we all know things that are alive. And we all know things that aren’t alive. And yet, it’s that gray area in between. So when we start saying, this is alive and this is dead, that’s fine. But when you say everything either has to be alive or dead, you’re setting up a false dichotomy. Because the taxonomy of what it means to be alive, I think is much, much richer than just dead or alive.
Strogatz: Hmm. How so?
Hazen (02:29): Well, think about it, you have an origin of life. So, that’s a really good metric. There was a point in our Earth’s history when there wasn’t a single living thing. It was a blasted surface, it was covered with volcanoes and magma, and it was just basically inhospitable. There was no place that life could even get a tiny foothold. But gradually, as the Earth cooled, as oceans formed, as the atmosphere became more palatable for some kind of living thing, we think there was a process. A historical process, the origin of life, in which chemical systems became gradually more complex, became more interesting. And at some point, yes, there was a first cell that probably had proteins and DNA. But there had to be something before that, and where do you draw the line? It’s just difficult to say there’s an absolute point in space and time when there was no life, and then the next point in space and time there was.
Strogatz (03:26): Interesting, interesting. So, the way you’re phrasing it, it sounds like it’s an issue of chemical complexity or something like that.
Hazen (03:34): It’s a question of chemical complexity. But it’s also a much more basic question of taxonomy. You know, it’s so easy for humans to think in dichotomies. Good, bad; black, white; day, night. These are things that make life simple. It means we can categorize things very, very quickly. And early on in human history, this was the defense mechanism, because you had to make decisions very, very quickly. Whether or not you were going to shake that person’s hand or shoot an arrow at them. So we needed to make these decisions.
But we don’t have to do that when we’re thinking about the larger issues of the natural world. The natural world is amazingly intricate and complex. And how those chemical complex systems emerge, and at what point a complex chemical system is something that we truly will call alive, is not at all obvious.
Strogatz (04:24): So, I get your point about the gray zone. I mean, it’s — black and white is usually too simple, applied to practically anything. That there’s always some — some ambiguity in between. Nevertheless, let’s say, in the case of space missions that NASA’s running, maybe off in the future, we will be trying — or even with earlier missions, when we sent probes to Mars and that kind of thing, there was a quest to see if we could detect life. And so, you would think in order to address that question objectively, you have to have some criteria for what you mean by — have you found it or not?
Hazen (04:56): And NASA did. NASA did have criteria. And mostly, it has to do with what I would call chemical idiosyncrasies. So, organic molecules, molecules made with a carbon backbone, are everywhere in the cosmos. Anywhere there’s carbon, you get this thing called “organic chemistry.” Lots of different kinds of molecules, they’re just sort of a jumble, a mush of these things [that] form.
(05:21): But life is very, very particular. And one thing I think we can say is, if something is alive, it’s going to put its energy into making a few molecules that work really well. And ignoring the vast number of molecules that don’t do much of anything. So, if you have a system that has the biological overprint, it’s going to show very specific groups of molecules. Maybe molecules that are what are called “chiral,” or left- and right-handed, maybe you’ll have a predominance of just the left-handed or just the right-handed molecule. Maybe you’ll have just strings of carbon that have multiples of 2, 2-4-6-8, rather than all the other odd numbers as well. Maybe you’ll have some other characteristic that wouldn’t form just by a random process, but forms by a selective process. So that’s what NASA was looking for. And I think that’s a smart thing to do.
Strogatz (06:13): That’s very interesting. The idea of chemical selectivity, you say, could be or at least was proposed by NASA to be a possible — well, nowadays, we speak of biosignatures, I don’t know if that would be the language they would have used at that time.
Hazen (06:26): Yeah, exactly right. That you’re looking for biosignatures. So I think if you see those chemical idiosyncrasies, you can say, wow, something really interesting happened here. And it doesn’t look like just the normal natural process, it looks like there was some real selection for function. Molecules that did a job, you know, they metabolized or they, they help build strong cellular structures or something like that. So, I think that’s what they were looking for.
(06:56) But the fact of the matter is, that doesn’t define life, does it? It just says, we’re looking for something that we think is a characteristic of the kind of life that we’re familiar with. How many other kinds of life might there be out there? And, and that’s something we just don’t know. We don’t have enough information to build a taxonomy. To say, these things are alive, and these things are dead, and these things have some other interesting chemical features that may be lifelike but don’t quite get us there.
Strogatz (07:23): What would be some others, then, besides functional?
Hazen (07:27): There’s chemical systems that might be able to make exact copies of themselves, but they wouldn’t undergo mutation and natural selection. There are chemical systems that might template themselves, so they grow laterally, and they get larger and larger, and they seem to grow, but they don’t really have this characteristic of encapsulating a separate entity that we think of as being lifelike. But they’re all interesting systems and they’re all part of a kind of continuum of chemical complexity. And to me, the much more interesting challenge is to develop this taxonomy.
(08:02) Now, think about the Linnaean classification system, where you have kingdoms and below the kingdoms, you have phyla, and you have orders and so forth. Well, maybe in our taxonomy of chemical complexity, we’d have a kingdom of nonliving things, and the kingdom of living things, and we’d have a kingdom of ambiguous things. And then beneath that, we’d have a whole bunch of other subcategories and subtypes, and we’d begin realizing that the universe is an amazing and wonderful place, and that chemistry does just extraordinary things, some of which we call life.
Strogatz (08:36): So, you’ve been emphasizing chemistry so far, which is interesting to me, given that I think of you as a person with a lot of expertise in mineralogy and geology. What about those fields? How do those overlap in this very expansive picture of the question of life and other interesting phenomena?
Hazen (08:55): It’s a really good question. And it goes to a more fundamental aspect of human nature.
Yeah, I’m trained in geology and mineralogy, so I see the origin of life in terms of geology and mineralogy, or geochemistry. It’s a process of chemical complexification. That the origin of life occurred on a nonliving planet, which means what you had is geology and chemistry. You didn’t have life. You were trying to jumpstart life, in a sense. So that’s my perception. Other people, like Sheref, who you’re going to be talking to, I mean, he has much more of a biochemical background. And so he thinks very deeply about DNA and RNA and information, that’s another aspect of life as we know it. Passing information from one generation to the next and storing it and copying it. Boy, that’s, that’s a molecular challenge.
(09:44) And I can imagine some really interesting chemical systems, some of which might even have attributes that we think of as lifelike, but don’t necessarily carry information. They’re just chemical systems that, because of the nature of the molecules themselves, just sort of reorganize themselves in fascinating ways. So there’s so many attributes to this and to me, trying to pin down one very specific set of criteria and say, “this is life and everything else isn’t” — it kind of defeats the whole purpose of exploring the wonder of nature, which itself is so infinitely varied and complex. Nature is what nature is, and we try to impose a taxonomy on it. But that doesn’t mean that we have all those nuances.
Strogatz (10:31): So then, getting back to NASA for a second, are there are some kinds of things that you think they should be looking for when searching for life on other planets? Or should they just be kind of going for the most glorious, rich, bountiful taxonomy they can come up with?
Hazen (10:46): Aha! Why not both? Because, you think about it. One thing we do have a hunch about is habitability. That is sort of the range of temperature, pressure, composition. A water-rich world, a sunlit world, you have to have energy, you have to have various other criteria that allow chemical systems to do interesting things. If it’s, if it’s, everything’s molten, or a vapor, it’s much too hot. If everything’s frozen, and nothing moves, like on Pluto, then that seems much too cold. So, so we do think there’s some sweet spots. And we do think there are things we can look for, like liquid water, or some other fluid, but water is the only one that really seems to do the job.
(11:28) We need to look for carbon-based molecules, because it seems like carbon’s the only element that forms the kind of richly varied backbones that you need for the structures of what we think of as life. And I really don’t believe in cloud-based life or, you know, electronic life, or life in a plasma or something like that. I mean, that just, you don’t see the kinds of structures that you need, that spell what I think of as the complexity of a living system. So there are parameters, and that’s what NASA is looking for. Let’s look for water-rich worlds, let’s look for worlds that have the right kind of temperature and pressure and atmospheric composition. And rocks and minerals play a really interesting role. And they provide all sorts of chemical elements in addition to carbon, that might be essential for a complex chemical system.
Strogatz (12:21): If you’ll allow me to get personal with you for a second, I’m sure you have some personal favorite things that you would love to see answered before you finish your career. Are there some you would share with us, things that puzzle you the most, or that are sort of dream questions you’d like to see us get more clues about?
Hazen (12:37): I would love to see microbes brought back from Mars. Microbes that had a different biochemistry, a different genetic code, if that’s part of the story, because that would indicate there was something called a second genesis. Second genesis, in the business of origin of life, just means that life has arisen more than once. And, you know, in the universe, there’s the old adage, “It’s 0, 1, many.” No life is sort of dull, but we know that’s not true, because we have one example. As soon as you find a second example, especially if it’s in our solar system, then you know life is absolutely everywhere. Because it just — it just arises, it’s as natural as the forming of basalt on a terrestrial planet. Life is just another chemical process that happens inevitably
(13:24) We don’t know if that’s true. And that’s what I’d love to know. I’d love to know that life was an inevitable consequence. And you know, the fact that I’ve spent a significant part of my career studying aspects of the origin of life means that I philosophically planted my flag in that field. If life never happened, or happened only once in the entire history of the cosmos, then it would be futile to try to study its origin. If it’s an incredibly rare chance event that only happens through the juxtaposition of just the right rocks and water and chemistry and so forth, and it only happens in one in every billion planets, then again, we’re not going to be able to reproduce it in the laboratory. Even if it occurs commonly, but it takes 100 million years for it to get started, it’s going to be really, really hard to do it, you know, in the four years of a postdoctoral fellowship. So I would like to think that life, once we figure out the tricks, that it’s something you can actually do in the laboratory.
Strogatz (14:22): Yeah, that’s such a fascinating question. I mean, everybody who’s thought about this wonders — I can remember reading a book by Francis Crick on this, Life Itself, where he’s talking about directed panspermia. I had never taken it seriously until I read his book, that if the probability of spontaneous formation of life is small enough, we really could be alone. I mean, just sort of on Copernican grounds, that, that we’re never anything special, I was always led to believe, of course there must be life — as soon as we find planets in other solar systems. I mean, it just seems like hubris to think we’re the only instance of life in the universe. But we don’t know, logically, as you say, the probability could be so, so tiny, we might be the only one.
Hazen (15:05): Steve, you’re absolutely right, we might be the only one. Or it might be that the only other living worlds, there may be thousands or millions of them in our Milky Way galaxy, but they’re so far away, and they’re so noncommunicative, we may never know. But I do think that this is a problem that if the answer is we’re not alone, that’s something we can actually hope someday to learn. The negative is going to be really hard to prove. But all you need to do is find that one other living world, and then we have a very profound insight about the way the cosmos works.
Strogatz (15:39): Wow. It’s a cosmic thought. You know, I’m sort of encouraged by how quickly life started here. Speaking of geology, like let’s put it in a geological perspective. Give me the numbers, roughly, how old the Earth is and how soon it starts to teem with life.
Hazen (15:56): Sure. So, Earth began to form at 4.567 billion years ago. And it was not habitable for the first period of time. It may have had a window of habitability for a few tens of millions of years, and then that huge impact, the Theia impact that formed the moon, and that just smooshed everything — the whole planet was encircled by a magma ocean, glowing, red hot, that had to cool. So that may have been 4.45 billion years ago, I think, something on that order, maybe as recently as 4.4. But that’s the kind of extreme beginning date that we can think about. And we know that by 3.8, life was well established. We have stromatolites, we have other signs of life that were clearly there.
(16:47) So that’s a block of, what, 600 million years, but I think life started much, much more quickly. But that’s a hunch, I think probably we are looking at millions or tens of millions of years for a process to occur. If it’s going to happen, you know, chemistry, you’ve got a vast surface area of Earth, you’ve got millions of years to play with, you’ve got all different kinds of chemical systems and fluxes. And so, Earth is a great experimental laboratory for chemistry. And with hundreds of millions of years to play with over the entire surface of the planet. Wow, that’s, that’s a lot of combinations of chemicals you can try. And life pops out of it.
Strogatz (17:25): It’s really this wonderful vision you’re giving us here, where it’s not just, now, geology and mineralogy and chemistry, but you’re bringing astronomy into it, too, with this story of the impact. Maybe just expand on that for a second? I’m not sure I’ve ever heard this idea of Theia — you’re talking about the origin of the moon, where the moon came from.
Hazen (17:44): So the origin of the moon. When Earth was first forming, it was sort of a solar system dance. You had all these bodies that were gravitationally greedy, and they kept, like vacuum cleaners, sweeping up all the smaller worlds. And so, in this game, the largest body always wins. Whatever has the most mass wins. And for a few tens of millions of years, Earth was competing with another smaller body, maybe about the size of Mars, is what I’ve seen the calculations say. And so these two bodies were — they’d pass close to each other and they wouldn’t quite kiss. But on one very dramatic day, one very dramatic moment, they collided.
(18:28) The smaller body, which has been called Theia, that’s the mother of the moon in Greek mythology. Theia collides with Earth. There’s just this epic mixing and mashing and baking of all the ingredients. And part of what’s left over becomes the moon, and part of what’s left over becomes part of Earth. And that clump of stuff that is blasted off the surface during the collision consolidates into the moon. And so we have this beautiful object in the sky. Much, much closer back then, by the way. The moon was probably only a few tens of thousands of miles away, which means it looked very, very large in the sky and the tidal effects were huge. But gradually the moon has been receding as — even as it does today. And that changes the whole surface condition of Earth. Earth was uninhabitable immediately after that collision. But then things settled down. Oceans, with big tides early on, and the moon shining in the sky and receding year by year and somewhere in that period of tens or hundreds of millions of years, life caught on.
Strogatz (19:40): Thank you, Bob, this has been so intriguing. I mean, really mind-blowing, actually, to think about the interplay of — well, I’m tempted to say names of subjects that we learn in school: Astronomy, geology, mineralogy, chemistry, biology, but the way you tell it, it’s really just one beautiful big story of, I don’t know how to summarize it. What would you say? What’s the end of my sentence there?
Hazen (20:02): Steve, it’s one unified web of knowledge. It’s a way of knowing science as this most remarkable way for humans, to look at our natural world. Not think about chemistry or geology or physics or astronomy, biology as separate things. But it’s one interconnected web, in which we see this amazing process of evolution, evolution of planets and moons, evolution of our solar system, and then the origin and evolution of life.
Strogatz (20:30): Thank you, Bob, thank you so much for joining us today.
Hazen: Thank you, Steve.
Announcer (20:37): Want to know what’s happening at the frontiers of math, physics, computer science and biology? Get entangled with Quanta Magazine, an editorially independent publication supported by the Simons Foundation. Our mission is to illuminate basic science and math research through public service journalism. Visit us at quantamagazine.org.
Strogatz (21:02): So, as we just heard, Bob Hazen questions the usefulness of a rigid definition of life, preferring instead to identify the characteristics of interesting chemical systems that display complex behavior. Our next guest has spent a lot of time thinking about cells that mimic life. When thinking about what life is, he agrees that evolution is a satisfying thing to look for. So is the ability to reproduce. But these criteria do not fully capture what we mean when we speak about life. For him, understanding what life is more broadly includes an organism’s ability to persist over time and interact with other living things. Sheref Mansy is a professor of chemistry in the department of chemistry, University of Alberta. He joins me now. Welcome.
Sheref Mansy (21:49): Thank you for having me.
Strogatz (21:50): It’s really a great pleasure. I’m very excited to hear about your work, which I found very captivating, I have to say. Okay, so let’s start with this thorny issue of trying to define life. I’ve heard in other interviews that you’ve given that you say you have conflicting emotions about this issue of trying to define life. What do you mean by that?
Mansy (22:09): I mean, I understand the criticisms, when people say that it’s perhaps not even worth the time to try to articulate what it is that we mean by life. I’ve, you know, some of my colleagues in the field at times will say things a bit provocative such as, you know, life is a term that’s just for poets, and scientists have no business using it. These things can of course seem like a distraction. But at the same time, I find it really bizarre that we have scientists all around the world that are trying to build something, but we can’t even say what it is that we’re trying to build. And how do you make progress in such a scenario?
Strogatz (22:44): Well, what’s so hard about it? I mean, maybe you could explain to us. Because, you know, the average person thinks, “I recognize something, this — the desk in front of me as we talk, that’s not alive.”
Mansy (22:55): I think the big problem is that every time somebody proposes a definition of life, there’s always somebody that can pop up and give an example of something that either we clearly perceive to be alive that doesn’t fit the definition, or even the other way around, you know, things that seem to fit the definition, but are not alive. So, you know, you can think of some sort of classic examples, like a mule. You know, so many people, you’ll ask, why isn’t your desk alive? They’ll say, “Well, it doesn’t reproduce, you know, living things are capable of reproduction.” But we have examples of living things, like a mule, that clearly everybody thinks is alive, but is incapable of having offspring.
(23:30) And then you can play the sort of opposite game as well, you know. So, salt crystals, you know, there’s lots of crystallographers out there. And, and one of the tricks that crystallographers use to, to grow more crystals is they crush old ones, and they use the little itty-bitty pieces of their old crystals to seed the growth of new crystals. And you can do that even with just salt, you don’t need to do that with proteins. And that’s an example of replication. But, you know, nobody’s deceived by that, right? Nobody confuses replicating salt crystals as being alive.
Strogatz (23:57): Right, right. So maybe not super useful to put a sharp boundary. Or to articulate necessary and sufficient conditions for life, because it seems like we could keep finding exceptions. But, on the other hand, it feels like, as you say, it’s hard to look for life elsewhere, like if we’re trying to find life on other planets, if we have no concept what we’re looking for. And so, to that end, you’ve mentioned this, this criterion of persistence over time. Wonder if you could unpack that for us a little bit.
Mansy (24:27): You know, basically, all things tend towards disorder, right? That’s sort of the second law of thermodynamics. And living things are out-of-equilibrium chemical systems. And so, if they weren’t alive, they would just sort of decay down back to their disordered component parts. That’s basically what we would call death, you know? Equilibrium essentially equals death.
(24:46): So what are these processes? What is the chemistry and physics behind life that essentially is always able to keep it out of equilibrium and persist over time to maintain that state, that highly ordered, sort of thermodynamically unfavorable state, over time? Obviously, it’s not forever, we do die, unfortunately, at one point. But that is an aspect, you know, that I guess you could just call it metabolism. But I think a lot of times, even there, when people use that word metabolism, they’re not really thinking of this angle. They’re more thinking in terms of, you know, molecule A gets converted to molecule B, then gets converted to molecule C. They don’t seem to really be thinking about what is the sort of trick that biology uses to keep itself away from equilibrium and maintain this highly ordered state over time.
Strogatz (25:30): So I’d really like to get into some work that you did over the past several years in your lab, about what we could call a cellular Turing test. What’s your take on it? I mean, give us the background. What was the Turing test? What was it trying to do in its day? And then let’s talk about, you know, your adaptation of it to the world of life.
Mansy (25:50): Sure. I mean, I should say that I wasn’t the very first person to come up with this idea. There’s a paper — you know, I just am highlighting all of my horrible memory, I think it was in Nature Biotechnology or Nature, where there was a lot of British scientists that sort of came together and said that this perhaps could be one way that we could address this lack of a definition of life to help us make progress. And they had proposed the cellular Turing test in this paper. And that was just one of those things that when I saw, I thought that, you know, I think I’m actually able to sort of put those pieces together in the lab and try to pull off a model system there.
(26:23) So basically, the whole idea is, you know, these same sorts of problems existed in the artificial intelligence field, which I don’t work in, artificial intelligence, so probably you can speak much better about that than I can. But basically, it was the same sort — from my understanding, the same sort of problem. You know, how can we tell if a machine or a computer program is displaying intelligence if we’re incapable of even defining intelligence in the first place? They had the same stupid arguments that we have in my field, you know, fighting back and forth as to what is the right definition and what exactly, you know, types of experiments we should be doing. And so what Turing essentially proposed is, let’s forget about this stupid fight, and come up with a functional test. And if you pass this test, then you know, we haven’t defined intelligence, but you’re at least moving in the right direction.
(27:09) And so, I always give a sort of very modern, I hope, modern take of it. You know, imagine that you’re on your cell phone, chatting with a friend or text messaging with a friend, and your friend has been substituted with a computer program. Are you able to figure out or realize that you’re no longer chatting with your friend? So if you’re not capable of distinguishing between your friend and the computer program that substituted for your friend, then they’ve passed, essentially. You’ve passed the Turing test, the machine or the program has passed. And so it has been, it’s successfully deceived you into thinking that you were chatting with your friend. So we, it was a manner in which you, you avoided coming up with a definition.
(27:48) And the opposite situation is also instructive, right? Because if it fails, and it doesn’t matter why it fails, you know, the response time is a bit strange, the vocabulary is different than how your friend would speak, then you have to go back to the drawing board and develop a better program or a better machine.
(28:02) And so basically, that was the inspiration for several of our projects. We were just seeing, can we build artificial cells that can engage in the same types of chemical communication that natural living cells engage in, and do it so well that they can deceive the natural cells into thinking that they’re speaking to a neighbor as opposed to the things that we built in the lab.
Strogatz (28:22): It’s really a fantastic, very elegant idea. But — to make living cells be fooled by these artificial cells, it’s really an interesting thought. And so, I mean, it sounds like you’ve done a series of experiments in this direction. What are some of them?
Mansy (28:36): So, lots of bacteria — we started off with bacteria, we figured that would be the easiest thing to do, and it’s also closer to the types of stuff I had done in the past. And so bacteria, they engage in chemical communication. Many of them exploit these small molecules called acyl-homoserine lactones. And so we figured, you know, these pathways are pretty well-known. People had engineered bacteria to talk to each other using these same known pathways. So we figured, you know, we should be able to reconstruct these same things in an artificial cell. So that was basically the goal.
(29:07) It turned out, as you know, always in science, to be a bit more challenging than we had anticipated. The synthesis and release of chemical signals from our artificial cells to natural cells was not difficult. I think we never failed in that. Every time we tried to reconstitute a known system in our artificial cells, that always worked. The part that was difficult was being able to sense the living cells through the molecules that they secrete. And I don’t have a good answer as to why that’s difficult. But you know, we’re bad comedians, I suppose, but I guess, as many couples would say, it’s, you know, it’s much easier to talk than to listen or to hear your partner. And so I think that’s the same thing with these artificial cells. It’s easier to engineer these things to talk than it is to listen.
(29:50) But we essentially never failed in speaking, you know, making artificial cells speak by synthesizing or releasing molecules. Getting them to hear was a lot more difficult, and the best one that we were able to reconstitute was the system that was taken from Vibrio fischeri. So it’s just, you know, a marine organism that naturally bioluminesces. And that was the pathway that we were able to fully reconstitute. But basically, in the ends, we were able to put these pieces inside of lipid vesicles to mimic, sort of morphologically somewhat, a cell. And if you take something like Vibrio fischeri, which naturally luminesces when it talks to each other, so when they reach a certain cell density, they know they’ve reached that density through communication with each other, they luminesce. So it’s a very simple kind of qualitative test. And so if we take this bacteria, and we grow them to half the density that they need to be to luminesce, and then we dump in our artificial cells to make up for the missing natural cells, they light up. We did do, you know, fancier experiments than that, but that was probably the most satisfying experiment, because at least visually, we could see right away that we were on the right track.
Strogatz (30:59): Okay. And the main point that you mentioned, I think, was that when there was a high enough density of them, they could act as surrogates for the bacteria, that — I mean, they could fool the bacteria into thinking that the quorum had been achieved, and therefore the living ones would light up. But these cells, these artificial cells, tell me more about them. They’re lipid vesicles. Do they have anything inside them?
Mansy (31:22): Yes. So they are lipid vesicles, fat molecules. They have an internal, let’s say lumen or internal aqueous space. And inside of there, we put the DNA constructs that we’ve engineered, you know. I don’t want to make it sound too fancy, because these are not huge genomes, but we do put, you know, engineered pieces of DNA inside that encodes for the function that we are — we’ve set out to achieve, which in this case was sensing, synthesizing and releasing chemical messages. Then we have to also put in the machinery that’s necessary for transcription and translation. So it’s to convert the information in DNA to RNA, and then that information, of course, into protein, which, you know, will have the enzymatic activity that we require for our cells. In these specific experiments of the cellular Turing test, we did use transcription and translation machinery that came from extracts of E. coli. So these are somewhat ill-defined mixtures.
Strogatz (32:15): Oh, yeah. That’s beautiful. I really like what you were just saying there. And I think that’s a very deep point that doesn’t get emphasized enough, the communal aspect of early life. We have spoken with other guests about the possibility of the massive horizontal gene transfer in very early life. I don’t know, maybe I’m getting carried away, but I like your thought that communication is really early and deep in the story of life, maybe deeper even than, than what we think of as evolution today, for the most part.
Mansy (32:15): And our system was incapable of growing and dividing, all it could do was essentially, you know, listen and speak. That’s all we programmed it to do. To give it more, you know, to endow it with more functionality would certainly take a lot more effort. But nevertheless, you know, I mean, I would say for this one specific task, which I think is a quite important aspect of life, by the way, something that doesn’t often pop up in definitions of life, this ability to organize and communicate with your neighbors. You know, my guess is this came really, really early. So I don’t think that we hit upon some sort of trick here. I mean, I think we are looking at something that is important, because we tend to look at life as really just these individual units, you know, can I build an actual, you know, one single cell or something along those lines, but I don’t think that’s how life works at all. You know, I mean, it is a community affair. Evolution, by definition basically, is a community type of process. If we find life on another planet, we’re not going to find just, like, one organism or one cell. I mean, these things don’t make sense, right? That’s not how biology works.
(33:46) I mean, I have to admit, in my head, I hadn’t really thought of it in exactly that way. I tend, I mean, I guess, you know, we all come at these things with our own biases. And mine, typically, is that I think that a lot of the stuff that we see as coming at different times, probably pieces of it were there from the beginning and emerged together. Because I tend to look at biology as being so incredibly complex. I don’t, I guess I just don’t see biology modular, the way I think a lot of people who try to engineer, you know, in the field of synthetic biology, they’re always trying to sort of put together, and they love using the analogies of Lego pieces and things like that. Which of course, in some ways we incorporate some of the stuff that they do. But I don’t really see biology as being modular. I see it as a very messy, intricate, you know, network of things that probably reflects its origins, you know, probably lots of these different processes that were necessary had to come up around the same time. Otherwise, it just wouldn’t have survived.
Strogatz (34:39): So on this early question that we were posing, about what is life, it sounds like you already hinted at this in a remark you made a few minutes ago that you would not have considered your, your artificial cells to be alive, but they had some of the functionality, right? You say, they had some of the important functions, but you pointed out, they couldn’t reproduce. What else could they not do?
Mansy (35:02): The thing that I find the most frustrating about these systems that we’ve built is they can basically listen once and then respond, or speak once. They’re, they’re not able to engage in a longer conversation, let’s say. And that is something that frustrates me. That reflects, in large part, what I was talking about before, this concept of persistence over time. It lacks a supporting metabolism to sustain this activity, to have turnover, you know, to degrade the molecules that were being used for talking and synthesize new ones. And just being able to sense once and respond once, to me, is not sufficient. If you want to make something that better mimics life, it’s got to persist for longer than that. And if you wanted to use this as a platform, you know, for some sort of technology, I think it would need to survive more than a couple of hours.
Strogatz (35:52): So that gets us into something that I was hoping we could explore together, about this idea that you mentioned, a platform. Please tell us about some of these fascinating studies that you and your group did recently with artificial cells trying to interact with neurons.
Mansy (36:07): If you think about communication, chemical communication, I mean, I would imagine that lots of people, the first thing that pops to their mind, is signal transduction through neurons. And of course, that’s — that’s true, right? And so for us, for a long time, even when we were working on a cellular Turing test, we really liked this idea. Could we build — I mean, I would not call it an artificial neuron, because it’s way too far from that, but something that can engage in communication with neurons? And that’s not just fun intellectually, but I think that also has possibilities for technologies, right? I mean, there are lots of diseases, neurodegenerative diseases like Parkinson’s disease, where essentially, your neurotransmitters like dopamine are not being produced as you get older. And, you know, if you built artificial cells that can sense the concentrations of dopamine, for example, and then synthesize more dopamine whenever the levels get too low, you know, that sounds like a fantastic therapy. You wouldn’t have to sort of flood the patients with tons of drug molecules that may or may not cross the blood-brain barrier and get to where you want to go. You could have artificial cells that are targeted to different parts of the body. Again, there’s a lot of work to get there.
(37:13) And then very locally, you know, whenever the concentrations drop below what’s needed, they can replenish the supplies. They sound really attractive. But again, I think this persistence problem, that, while I find it interesting from a more, let’s say, intellectual perspective on what is life, has very practical implications as well. I mean, what’s the point of taking an artificial cell into your body if it just, you know, falls apart almost immediately?
Strogatz (37:36): As you say, if this artificial cell could exist and thrive for a certain amount of time, and do smart things like sense whether a cancer cell is present, and if so what molecules, what chemotherapeutic agents to dump on that one — we’re not there yet, it sounds like, but that’s the vision, right? That’s the dream, maybe?
Mansy (37:56): Yes, yes, yes, yes. I mean, to be able to encode multiple outputs, obviously, you know, and to increase the capacity for synthesis so that you can actually carry out whatever, you know, the synthesis for whatever drug molecules you need, that I think would just be insanely satisfying.
Strogatz (38:11): And an interesting example of how fundamental thinking about research, like, you might be really driven by your curiosity about origin of life, or thinking about this deep question of what does it mean to be alive or not alive? And then, out of that pure curiosity-driven research comes these fantastic biomedical applications. It’s not far-fetched, I think.
Mansy (38:31): For a long time, I mean, I would run into colleagues that did neurobiology, and this kept popping up over and over again. So we built artificial cells because of the difficulty in sensing stuff. We had them sense the same sort of molecule that would have been secreted from bacteria. So in that sense, the listening component was, you know, listening to bacteria talk. But in response, it could talk to neurons, and so what we actually did was, they were neural stem cells. And this was done in collaboration with three different biology labs, because I have no experience with eukaryotic biology or doing any sort of tissue culture stuff. So this, you know, took a dedicated Ph.D. student, his name is Duhan Toparlak. Basically, these artificial cells could synthesize and release brain-derived neurotrophic factor, which is a neurotrophic factor, as the name suggests, that impacts the differentiation of neurons into, from immature to, let’s say, mature neural cells. So we took these neural stem cells, we incubated them with our artificial cells, we added the molecule that bacteria would secrete, we didn’t actually mix bacteria with the neurons. So it was sensing this molecule in the environment and in response, synthesized and released something that guided, in some sense, the differentiation of neural stem cells. So that’s what we did. It, in some sense, sounds like we did less in some ways than we did with the cellular Turing test. But it took four or five years actually, to get that to work.
Strogatz (40:05): I see. So really, what’s going on in the experiment is there’s artificial cells, there’s neural stem cells, and there’s you dumping in the thing that’s telling these artificial cells, “hey, say something. Say something helpful to make the neurons grow and differentiate.”
Mansy (40:22): Exactly.
Strogatz: And you did it. I mean, sounds like this was a hard experiment from the way you’re describing it.
Mansy: Yeah, painful process. But in the end, we got it to work.
Strogatz: Well, thank you, Sheref. This was super interesting, and I really appreciate your taking the time to talk to us today.
Mansy: It was a lot of fun. Thank you for having me.
Announcer (40:40): Stay up to date on the latest happenings in science and mathematics. Sign up for the Quanta Magazine newsletter. It’s free, landing every Friday in your email inbox. Head to quantamagazine.org for more info on how to sign up.
Strogatz (40:54): The Joy of Why is a podcast from Quanta Magazine, an editorially independent publication supported by the Simons Foundation. Funding decisions by the Simons Foundation have no influence on the selection of topics, guests, or other editorial decisions in this podcast or in Quanta Magazine. The Joy of Why is produced by Susan Valot and Polly Stryker. Our editors are John Rennie and Thomas Lin, with support by Matt Carlstrom, Annie Melchor and Leila Sloman. Our theme music was composed by Richie Johnson. Our logo is by Jackie King, and artwork for the episodes is by Michael Driver and Samuel Velasco. I’m your host, Steve Strogatz. If you have any questions or comments for us, please email us at [email protected]. Thanks for listening.