What Lights the Universe’s Standard Candles?
Introduction
Every year, around 1,000 Type Ia supernovas erupt in the sky. These stellar explosions brighten and then fade away in a pattern so repeatable that they’re used as “standard candles” — objects so uniformly bright that astronomers can deduce the distance to one of them by its appearance.
Our understanding of the cosmos is based on these standard candles. Consider two of the biggest mysteries in cosmology: What is the expansion rate of the universe? And why is that expansion rate accelerating? Efforts to understand both of these issues rely critically on distance measurements made using Type Ia supernovas.
Yet researchers don’t fully understand what triggers these strangely uniform explosions — an uncertainty that worries theorists. If there are multiple ways that they can happen, tiny inconsistencies in how they appear could be corrupting our cosmic measurements.
Over the past decade, support has accrued for a particular story about what sets off Type Ia supernovas — a story that traces each explosion to a pair of dim stars called white dwarfs. Now, for the first time, researchers have successfully re-created a Type Ia explosion in computer simulations of the double white dwarf scenario, giving the theory a critical boost. But the simulations also produced some surprises, revealing how much more we have to learn about the engine behind some of the most important explosions in the universe.
Detonating a Dwarf
For an object to serve as a standard candle, astronomers must know its inherent brightness, or luminosity. They can compare that to how bright (or dim) the object appears in the sky to work out its distance.
In 1993, the astronomer Mark Phillips plotted how the luminosity of Type Ia supernovas changes over time. Crucially, nearly all Type Ia supernovas follow this curve, known as the Phillips relationship. This consistency — along with the extreme luminosity of these explosions, which are visible billions of light-years away — makes them the most powerful standard candles that astronomers have. But what’s the reason for their consistency?
A hint comes from the unlikely element nickel. When a Type Ia supernova appears in the sky, astronomers detect radioactive nickel-56 flooding out. And they know that nickel-56 originates in white dwarfs — dim, fizzled-out stars that retain only a dense, Earth-size core of carbon and oxygen, enshrouded by a layer of helium. Yet these white dwarfs are inert; supernovas are anything but. The puzzle is how to get from one state to the other. “There still isn’t a clean ‘How do you do this?’” said Lars Bildsten, an astrophysicist and director of the Kavli Institute for Theoretical Physics in Santa Barbara, California, who specializes in Type Ia supernovas. “How do you get it to explode?”
Until around 10 years ago, the prevailing theory held that a white dwarf siphoned gas from a nearby star until the dwarf reached a critical mass. Its core would then become hot and dense enough to spark a runaway nuclear reaction and detonate into a supernova.
Then in 2011, the theory was overthrown. SN 2011fe, the closest Type Ia found in decades, was spotted so early in its explosion that astronomers had the chance to look for a companion star. None was seen.
Researchers shifted their interest to a new theory, the so-called D6 scenario — an acronym standing for the tongue twister “dynamically driven double-degenerate double detonation,” coined by Ken Shen, an astrophysicist at the University of California, Berkeley. The D6 scenario proposes that a white dwarf traps another white dwarf and steals its helium, a process that releases so much heat that it triggers nuclear fusion in the first dwarf’s helium shell. The fusing helium sends a shock wave deep into the dwarf’s core. It then detonates.
But is this what really happens?
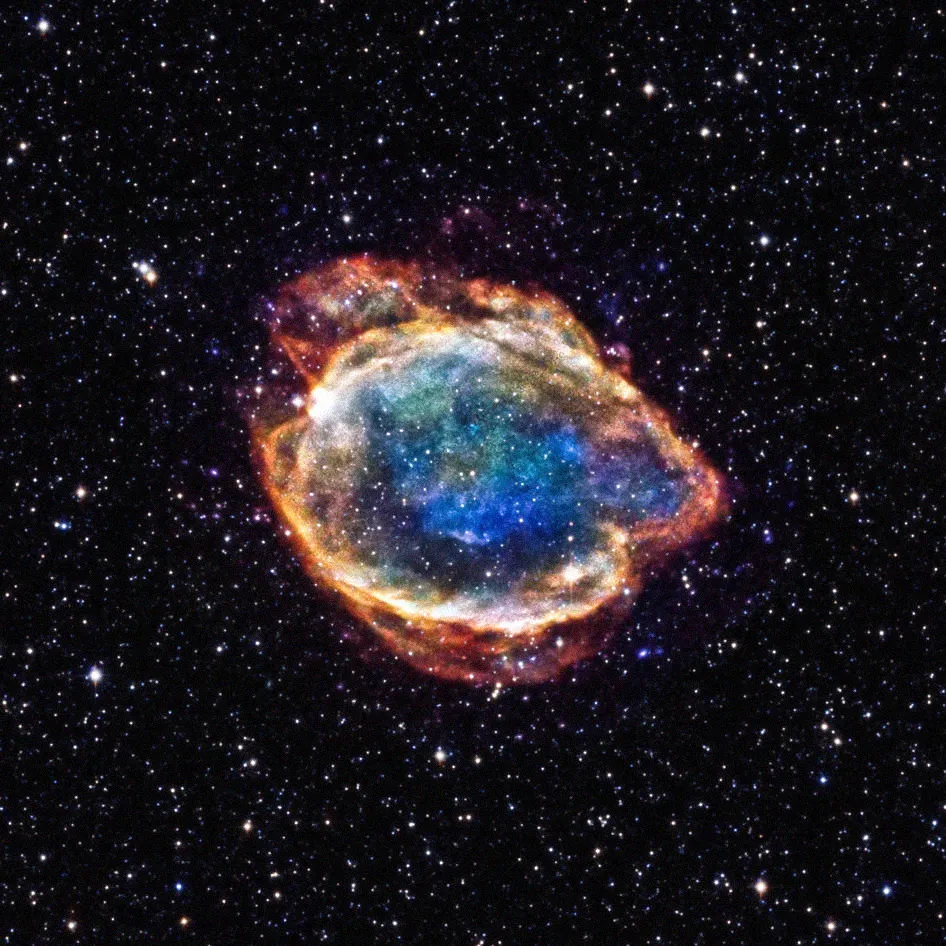
About 4,500 years ago, a Type Ia supernova exploded in our Milky Way galaxy, leaving this remnant of high-energy debris. The red, green and blue colors in the image are representations of low, medium and high-energy X-rays, respectively.
X-ray: NASA/CXC/U.Texas/S.Post et al, Infrared: 2MASS/UMass/IPAC-Caltech/NASA/NSF
Shen thought of a way to check: If there are two white dwarfs rotating around each other, and one explodes as a supernova, nothing will be left to hold onto the other. Like a swinging lasso that’s suddenly released, it should fly away as a “hypervelocity” white dwarf.
If the D6 theory is correct, hypervelocity white dwarfs should be common. If it’s wrong, there should be none.
The opportunity to test the scenario arrived in 2018, when the European Space Agency’s Gaia space telescope released a massive new census of objects in the Milky Way. On the day of the release, Shen and his team stayed up all night analyzing the data. They found three fast-moving white dwarfs. Not many, and not none. This was troubling.
Simulating Supernovas
Around this time, multiple teams set to work on computer simulations to test the D6 hypothesis.
Shen and colleagues published simulations in 2021 that played out the aftermath of a D6 detonation. The radioactive nickel-56 nuclei should disintegrate into additional particles, which will then spend months decaying and interacting in the region around the supernova. (Most of our earthly manganese, nickel and cobalt, and a large fraction of our iron, probably originated in reactions such as these.) To capture the tumult, Shen and company simplified the math: They assumed the supernova is perfectly spherical and then simulated the physics along a single line radiating outward from the center.
Strikingly, this “one-dimensional” simulation yielded the correct luminosity curve. “There was no way I would have seen that coming,” Bildsten marveled. “They’re showing they can get a supernova to fall on the Phillips relation, so that’s pretty exciting.”
To verify that a detonation can happen in the first place, though, two other groups were busy developing sophisticated supercomputer simulations of the D6 scenario in three dimensions.
One of these teams recently showed that the D6 scenario can indeed trigger a supernova. The researchers, led by Ruediger Pakmor at the Max Planck Institute for Astrophysics in Garching, Germany, simulated a primary white dwarf with a thick helium outer layer. As the star sucked even more helium from its companion, its outer layer ignited. The explosion traveled quickly around the white dwarf, sending a shock wave deep inside the core that detonated the carbon and oxygen.
But Pakmor’s simulations also produced a strange result. The shock wave traveling through the primary white dwarf sometimes smacked into the companion dwarf hard enough to trigger a supernova in that star as well. This happened in the simulations when the companion’s mass was less than 70% of our sun’s mass, as is usually the case with white dwarfs.
If both white dwarfs often go supernova together, this could explain why fewer hypervelocity white dwarfs are seen. But astronomers have met the news of Pakmor’s double-supernova simulations with caution. “I’m not convinced it happens,” Shen said, “but that’s a really interesting possibility.”
Another team, led by Robert Fisher at the University of Massachusetts, Dartmouth, used a thinner layer of helium than Pakmor did. In their simulations, they saw the helium ignition travel more slowly around the dwarf, and the resulting shock wave converged to a point off-center relative to the carbon-oxygen core. The core then failed to detonate in a Type Ia supernova.
Both groups are baffled by the contradictory results. Pakmor’s team tried a thinner helium layer like Fisher’s but still found that their system went supernova.
One challenge for these simulations is that the helium thickness and other conditions are just guesses. Another issue is that, to simulate star-size objects, the simulations coarsely divide space into kilometer-size chunks. But the focusing of heat that triggers a detonation occurs on the scale of centimeters. The scientists make choices about how to capture the interaction between these disparate scales.
For now, the book remains open on Type Ia supernova origins. Until the discrepancies can be resolved, both teams are hesitant to conclude that the D6 scenario is responsible for all or even most of them. Still, finally seeing one explode in a supercomputer was a bright step forward, even if seeing two was a surprise.
Correction: February 8, 2023
The European Space Agency, not NASA, runs the Gaia space telescope.